Introduction
In Korea, most slaughterhouse blood is discarded (Kim et al., 2012; Yun et al., 1998). Not only are the costs of slaughterhouse blood disposal high but also the disposed blood is an environmental problem since it is a major source of water pollution (Jang et al., 2011; Jeon et al., 2013). However, slaughterhouse blood has considerable nutritional value (Quigley et al., 2004), and spray-dried animal plasma (SDAP) has been shown to improve the feed intake, weight gain, and morbidity of livestock (Pérez-Bosque et al., 2016; Torrallardona, 2010). These effects are greater in animals during challenge with a pathogen or bacterial toxin (Coffey and Cromwell, 1995; Pérez-Bosque et al., 2008). Furthermore, animal plasma contains useful proteins, such as fibrinogen, albumin, and globulin (Hyun and Shin, 1998; Pérez-Bosque et al., 2016; Pierce et al., 2005), and the beneficial effects of the animal plasma are mainly modulated by its immunoglobulin (Ig) components (Lallès et al., 2009).
Ig derived from animal plasma shows antibacterial activity against some pathogens by inhibiting their growth and anti-toxin activity through binding to lipopolysaccharide (LPS) or toxin (Han et al., 2009). It was also reported that Ig could improve the growth of beneficial bacteria, such as lactic acid bacteria, and inhibit the growth of harmful pathogenic bacteria in the gut (Balan et al., 2011). Although several studies that used whole blood or enzymetreated plasma, have demonstrated effective utilization of slaughterhouse blood in Korea (Hyun and Shin, 1999; Ma et al., 2001; Park, 1997), the blood has not been commercially utilized, and almost all of it is discarded.
In general, slaughterhouse blood accounts for approximately 3.5% of livestock weight, equaling approximately 7.5 L for a cow, 3.5 L for a pig, 50 mL for a duck, and 35 mL for a chicken (Jeon, 2015). Currently, approximately 523 tons of animal blood is being produced in slaughterhouses every day, and the amount of slaughterhouse blood discarded each year is about 126,000 tons (Kim, 2014). The amount of slaughterhouse blood has been consistently increasing annually, and more porcine blood is discarded than any other animal blood (Jeon, 2015). The estimated cost for the treatment of about 35,000 tons of porcine blood waste produced in 2014 was 227 million won (Kim et al., 2016).
Therefore, the aim of this study was to effectively purify an Ig-rich fraction from porcine blood and evaluate its commercial potential by assessing its anti-pathogenic properties using various in vitro assays.
Materials and Methods
Fresh porcine blood was collected from a local slaughterhouse, and immediately mixed with sodium citrate (1%). Plasma was separated from the porcine blood by centrifugation at 10,000×g for 20 min at 4°C (1736R; LaboGene, Korea). Calcium chloride (0.5%) was added to the plasma, which was stirred for 5 min and then incubated for 1 h at room temperature to promote serum separation. Ammonium sulfate (1.7 M) was slowly added to the serum, stirred overnight at 4°C, and then centrifuged at 10,000×g for 20 min. The precipitate was dissolved in distilled water, and the pH was adjusted to 9. The solution was filtered through a 10-kDa cutoff membrane using ultrafiltration (Vivaflow 50 System; Sartorius, Germany), and then the pH was adjusted to 7.4. The final product (PIC) was spray-dried using a pilot spray dryer (Yoojin, Korea).
The bacterial strains used in this study were obtained from the Korean Collection for Type Cultures (Korea) and the Korean Culture Center of Microorganisms (Korea). The pathogenic bacteria were grown in brain heart infusion medium, columbia broth, marine broth, trypticase soy broth, or reinforced clostridial medium for 18 h at 37°C (Table 1). Prior to the experiments, bacteria were subcultured at least three times. For long-term storage, stock cultures were stored in fresh broth containing 20% glycerol at −80°C. The lymphoblast K-562 cell line was purchased from the Korean Cell Line Bank (Korea). These cells were cultured in Roswell Park Memorial Institute 1640 medium (Gibco, USA) supplemented with 10% fetal bovine serum (Hyclone), penicillin (100 U/mL), and streptomycin (100 µg/mL) at 37°C in a humidified atmosphere of 5% CO2.
ATCC, American Type Culture Collection; KCTC, Korean Collection for Type Cultures; RCM, reinforced clostridial medium; TSB, trypticase soy broth; BHI, brain heart infusion medium; CB, columbia broth; MB, marine broth.
The protein composition of the purified PIC was analyzed by SDS-PAGE in a 12.5% acrylamide gel as described by Laemmli (1970). The samples, which included porcine IgG (Sigma), porcine plasma, PIC, and bovine serum albumin (BSA, Sigma), were mixed with sample buffer (0.125 M Tris-HCl, 4% SDS, 20% glycerol, and 2% β-mercaptoethanol, pH 6.8) and heated for 15 min at 98°C. Electrophoresis was conducted at 20 mA (per gel) for 1 h using a Mini-Protean® Tetra System and PowerPacTM HV (Bio-Rad, USA), and the gel was stained with Coomassie® brilliant blue G-250 (Bio-Rad) for 2 h. The bands on the gel were analyzed with a Molecular Imager® GelDocTM XR plus Imaging system and Image LabTM software (version 5.1; Bio-Rad).
The concentrations of IgG and IgM in the purified PIC were measured by enzyme-linked immunosorbent assay (ELISA). All reagents were purchased from Bethyl Laboratories, Inc. (USA). The sample and reference were diluted to an appropriate concentration with sample diluent (50 mM Tris, 0.14 M NaCl, 1% BSA, 0.05 Tween 20, pH 8.0). Flat-bottom 96-well plates (Nunc) were coated with affinity-purified antibody (diluted in coating buffer), incubated for 1 h at room temperature, and then washed four times with washing solution (50 mM Tris, 0.14 M NaCl, 0.05% Tween 20, pH 8.0). The remaining binding sites were blocked with blocking solution (1% BSA in 50 mM Tris, 0.14 M NaCl, pH 8.0) for 30 min, and then the plate was washed four times. The diluted sample and stand- ard were added to the plate, which was incubated for 1 h, and then washed four times. An HRP detection antibody was added to each well and incubated for 1 h. The plate was washed four times, and then incubated with TMB substrate solution in the dark for 10 min. The reactions were stopped by the addition of 0.18 M H2SO4, and the absorbance was measured at 450 nm using a microplate reader (Emax; Molecular Devices, USA).
The PIC solution was diluted to 5% (w/v) or 10% (w/v) in buffered peptone water (BPW; Difco), and a BSA solution (10%, diluted with BPW) was prepared for use as a positive control. All of the solutions were filtered through disposable membrane filter units (0.8 and 0.45 µm; Advantec®) before use in the growth inhibition test. Pathogen cultures (0.1 mL) were diluted to 1×104 CFU/mL, and then BSA or PIC solution (0.9 mL) was added. The mixtures were cultured for 3 h at 37°C, and then incubated on optimal medium agar for 48 h at 37°C for counting. As a negative control, BPW (0.9 mL) without any protein was used. All measurements were performed in triplicate, and the percent growth was calculated as follows: Growth (%) = (Log10Nm/Log10Nc) × 100; where Nc and Nm are the numbers of pathogens in the control and in test mixture, respectively, after 3 h incubation.
The antigenic cross-reactivity of the PIC was investigated using the method described by Tomita et al. (1995), with a slight modification. Briefly, the pathogen cultures were incubated for 18 h at 37°C and then washed three times with phosphate buffered saline (PBS; Sigma), and the optical density (OD) at 610 nm of the final pathogen suspension was adjusted to 1.0. The pathogenic antigens were bound to a flat-bottom 96-well plate by incubation overnight at 4°C, and washed four times with washing buffer (PBS containing 0.05% Tween 20). The remaining binding sites were blocked by incubation with blocking buffer (3% BSA in PBS) for 1 h at 37°C and then washed four times. Then, the PIC solution (100 μL of 10 mg/mL) was added to each well, and the plate was incubated for 2 h at 37°C. After the plate was washed four times, a HRPconjugated goat anti-pig IgG antibody (Bethyl) was added to each well and incubated for 2 h at 37°C. The absorbance was measured as described above. The positive control was PIC solution without any pathogen suspension. All measurements were performed in triplicate, and the percentage of antigenic cross-reactivity was determined according to the following formula:
Antigenic cross-reactivity (%) = (mean OD450 of pathogenic antigen/mean OD450 of positive control) × 100
The anti-toxin activity of the PIC against LPS was determined using the method of Yu and Kanost (2002), with a slight modification. Flat-bottom 96-well plates were coated with two types of LPS from E. coli O111:B4 or S. enterica serotype Typhimurium (10 μg or 0.1 μg; Sigma) by overnight incubation at 4°C, and then washed four times with washing buffer (PBS containing 0.05% Tween 20). After washing, the plate was blocked by incubation with blocking buffer (3% BSA in PBS) for 1 h at 37°C, and then washed again four times. PIC diluted in PBS was added to each well at various concentrations and incubated for 2 h at 37°C. After the plate was washed four times, an HRP-conjugated goat anti-pig IgG antibody (Bethyl) was added to each well, and the plate was incubated for 2 h at 37°C. The absorbance was measured as described above.
In addition, the binding activity of the PIC to LPS was investigated using the lymphoblast K-562 cell line and the 3-(4,5-dimethylthiazol-2-yl)-2,5-diphenyltetrazolium bromide (MTT) assay according to the method described by Han et al. (2009), with a slight modification. K-562 cells (1×104 cells/well) were cultured in a 96-well plate (Nunc) for 24 h at 37°C in the presence of 5% CO2. PIC solution (50 μL) prepared at various concentrations was mixed with 50 μL of LPS solution (160 μg/mL) from E. coli O111:B4 or S. enterica serotype typhimurium, and the mixture was incubated for 30 min at 37°C. The mixtures were transferred to the plate, which was incubated for 72 h at 37°C. Then, 100 μL of MTT solution (1 mg/mL) was added to each well, and the plate was incubated for 4 h at 37°C. The medium was removed, and 150 μL of dimethyl sulfoxide was added. The plate was shaken for 10 min at room temperature, and then the absorbance at 540 nm was measured using a microplate reader.
Results
The Ig concentrates from porcine blood were effectively purified by eliminating other components, such as albumin (Fig. 1). The heavy and light chains of Ig migrated at approximately 49 and 26 kDa on the SDS-PAGE gel, respectively. The ELISA results showed that, on a protein basis, the PIC consisted of approximately 63.2±2.9% IgG and 7.2±0.4% IgM.
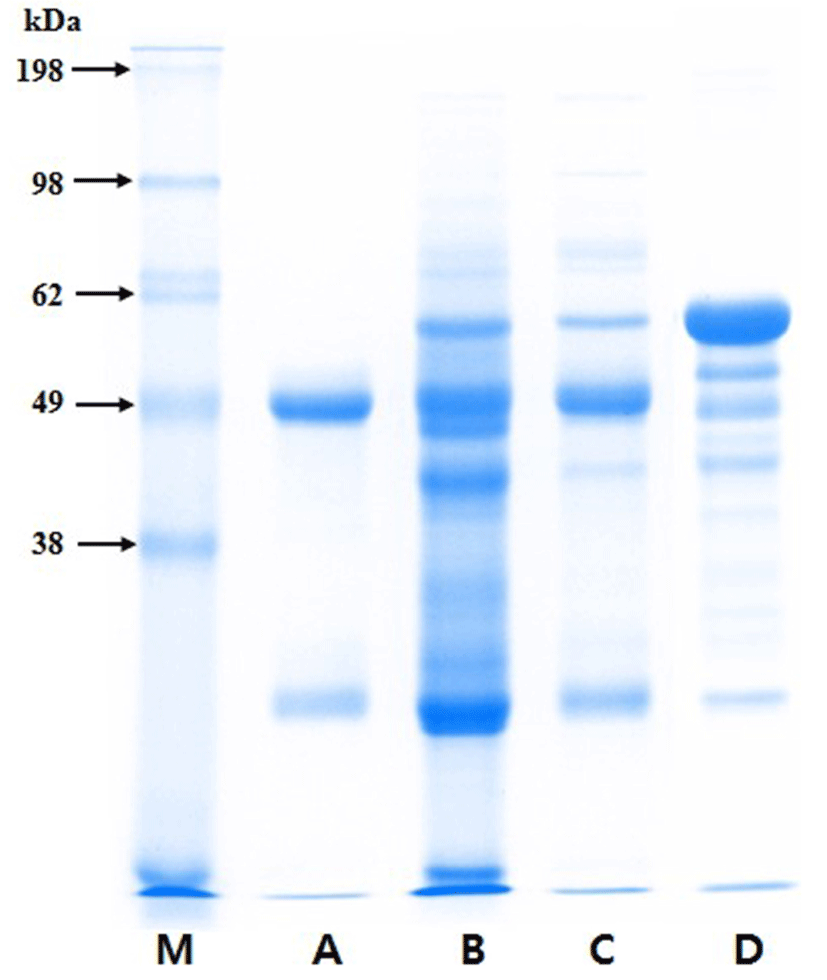
The PIC significantly suppressed the growth of all tested pathogenic bacteria when compared to their growth in the control or BSA treatment. However, the degree of growth suppression activity differed according to pathogen strain and PIC concentration (5% or 10%). Representative inhibitory activities of the PIC against the growth of various pathogens are shown in Fig. 2. When treated with 10% PIC, A. viscosus ATCC 15988 and S. mutans ATCC 25175 were the most effectively suppressed, by about 45-55%. In contrast, the BSA solution did not exhibit anti-growth activity against the tested pathogens.
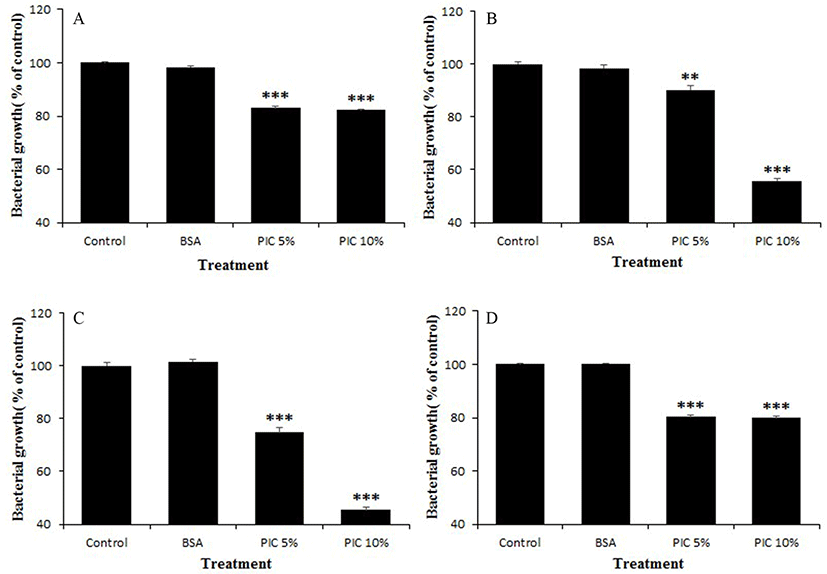
As shown in Fig. 3, the antigenic cross-reactivity of the PIC varied according to the pathogen strain. The PIC was able to bind to all the tested pathogens, including both gram-positive and gram-negative bacteria, shown in Table 1. In particular, the binding activity of PIC to some pathogens, such as C. perfringens ATCC 13124, S. aureus ATCC 25923, S. epidermidis ATCC 12228, E. corrodens ATCC 23834, E. coli ATCC 9637, and V. vulnificus ATCC 27562 was very close to that of the control.
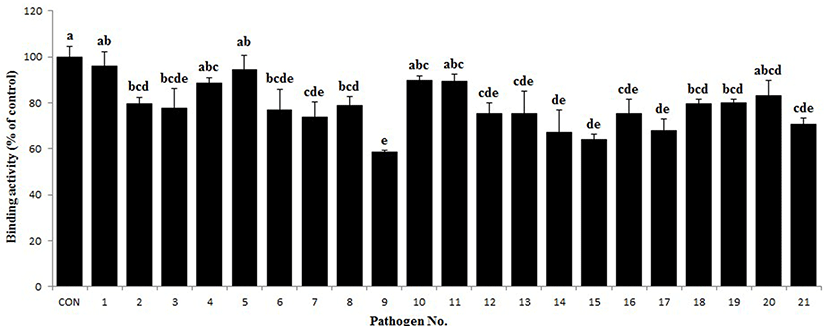
The binding of PIC to LPS from E. coli O111:B4 and S. enterica serotype typhimurium was PIC concentrationdependent (Fig. 4). The slope of the curves rapidly increased to about 50% saturation and then increased moderately with a corresponding increase in PIC concentration. When treated with a larger amount of LPS (10 μg/well), the absorbance values were higher than samples treated with a smaller amount of LPS concentration (0.1 μg/well). The anti-toxin activity of the PIC was also examined using the K-562 cell line. The growth rate of the K-562 cells was decreased by treatment with LPS (160 μg/mL) but, this reduction was restored by the addition of PIC (Fig. 5). Treatment with PIC at 300-500 μg/mL markedly increased the viability of the K-562 cells (p<0.05 or p<0.01), which means that the PIC could bind to LPS, reducing the pathogenicity of LPS.
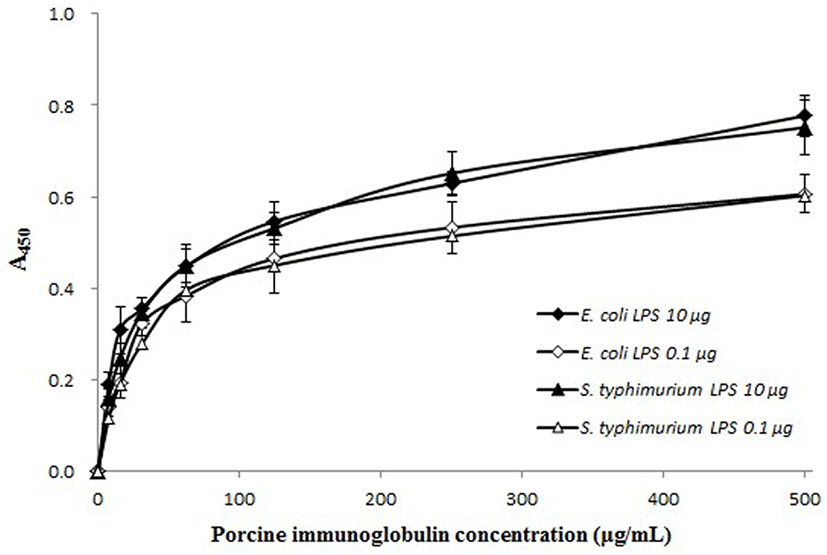
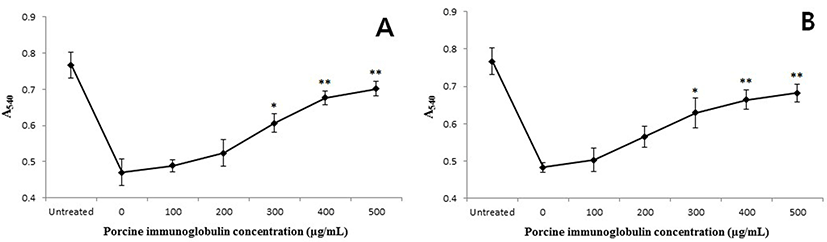
Discussion
It is known that administration of animal plasma in livestock improves intestinal barrier function and mucosal inflammation as well as growth performance (Bosi et al., 2004; Peace et al., 2011). Pierce et al. (2005) reported that the beneficial effects of animal plasma were primarily mediated by the Ig fraction. Administration of Ig increased the levels of anti-inflammatory cytokines in the intestinal mucosa (Pérez-Bosque et al., 2008) and reduced the expression of pro-inflammatory cytokines, such as tumor necrosis factor alpha, interferon gamma, interleukin 2, and interleukin 17, in various in vitro models (Bosi et al., 2004; Moretó and Pérez-Bosque, 2009). Other studies have shown that dietary supplements with Ig improved intestinal health and immunity in clinical trials (Asmuth et al., 2013; Wilson et al., 2013). The Ig concentrate isolated from porcine blood in this study contained approximately 63% IgG on a protein basis. This suggests its potential value as a supplement for functional food or animal diet, because commercial Ig fractions generally contain less than 50% IgG.
In the present study, the PIC not only inhibited the growth of various pathogens but also directly bound to their surface. It was reported that IgM had antibacterial activity and decreased the production of matrix metalloproteinase-1 caused by LPS (Zhou et al., 2007). Petschow et al. (2014) reported that Ig, especially IgG, had antibacterial activity against pathogenic bacteria, because Ig inhibited the ability of pathogens or their toxins, which migrated through the mucus layer into epithelial cells, and damaged pathogens through direct binding. Furthermore, the Fab fragment of IgG imparts diversity, and the Fc fragment can interact with the Fc gamma receptors on some immune cells, including macrophages and monocytes, thus stimulating their phagocytic activity (Petschow et al., 2014). Several studies have reported that the Ig present in SDAP reduced pathogen adhesion to the gut mucosa as well as the intestinal inflammation caused by pathogenic bacteria, thus providing immunological benefits (Pérez-Bosque and Moretó, 2010; Van Dijka et al., 2001).
PIC can bind to LPS on the surface of gram-negative bacteria and reduce its growth-repressing action on K-562 cells. Hence, PIC may protect the intestine from diseases caused by gram-negative bacteria and their toxins. Bacterial LPS can cause various tissue pathologies and intestinal permeability disorders, such as Crohn’s disease, necrotizing enterocolitis, and inflammatory bowel disease, by increasing intestinal epithelial tight junction permeability (Guo et al., 2013; Magata et al., 2015). The enterotoxigenic E. coli (ETEC) and Salmonella strains used in the present study induce severe enteropathy. ETEC adheres to the intestinal epithelium through various routes using colonization factors, which include more than 20 known multimeric structures, and it is the most common cause of diarrhea (O'Ryan et al., 2015). Salmonella strains, as foodborne enteropathogens, cause serious gastrointestinal invasive diseases (MacLennan et al., 2014). The Ig present in plasma might provide effective protection from these bacterial toxin-involved intestinal diseases, and increase phagocytic activity and lymphocyte proliferation (Balan and Moughan, 2013; Niewold et al., 2007). Previous studies have also reported that supplementation with SDAP reduced the expression of inflammatory cytokines and prevented the inflammatory diseases caused by LPS (Pérez-Bosque et al., 2016; Touchette et al., 2002).
Conclusion
In this study, we effectively isolated Ig concentrate from porcine blood. We demonstrated that the produced PIC has anti-bacterial and anti-toxin activities, which may provide protection against pathogenic bacteria. The commercial development of PIC would not only reduce the cost burden of slaughterhouse blood disposal but may also be a potentially valuable functional food material or supplement as an alternative to antibiotics in livestock diets.