Introduction
Alkaline-treatment has been extensively applied to improve hydration properties of lignocellulose compounds, thereby reducing lignin content and exposing hydroxyl groups (binding site to water molecules) of cellulose (Neto et al., 2013; Sangnark and Noomhorm, 2003; Sun et al., 2004). This approach has been particularly used for the economic utilization of agro by-products that contain high amount of lignocellulose compounds, such as sugarcane bagasse (Pandey et al., 2000). Sugarcane bagasse, which is an agro-waste produced from a commercial sugar production, has recently received a great interest as the source of insoluble fiber-rich food ingredient due to its high cellulose content (approximately 50%) and abundant availability (Zhuang et al., 2016; 2017). A previous study has indicated that alkaline hydrogen peroxide treatment could improve hydration and fat retention properties of insoluble fiber fraction from sugarcane bagasse, resulting from the elimination of lignin and its physical swelling (Sangnark and Noomhorm, 2003). Thus, alkaline-treated sugarcane fiber would be used as a functional ingredient for improving water-holding capacity, emulsifying capacity/stability and texture of various processed foods.
Insoluble dietary fiber from several plant resources, mainly containing lignocellulose compounds, has been practically used as a functional ingredient in processed meat products (Elleuch et al., 2011). Since it has an excellent hydration and fat retention properties, insoluble dietary fiber is often incorporated into emulsified meat products to improve cooking yield, emulsion stability, and texture (Thebaudin et al., 1997). When an additional water is formulated to replace certain portion of animal fat, in particular, the hydration property plays an important role in holding the additional water in low-fat meat emulsion system (Choi et al., 2009). In this regard, it has been generally known that the efficacy of insoluble dietary fiber for improving the technological properties of meat emulsion system could be influenced by the types, the pre-treatment methods, and the adding amounts of insoluble dietary fiber, as well as, the original formula of applied meat products (Choi et al., 2008; 2010; Fernández-López et al., 2004).
Taken together, it would be reasonable to hypothesize that the improved functional properties of alkaline-treated sugarcane fiber could result in greater positive impacts on water-holding capacity and texture of meat emulsion, as compared to crude sugarcane fiber. Recently, Zhuang et al. (2017) reported that the addition of 2% alkaline-treated sugarcane fiber could improve water-holding capacity and gel strength of myofibrillar protein gel. In addition, alkaline-treated sugarcane fiber, as a fat replacer, could enhance physicochemical and microstructural properties of low-fat meat emulsion (Zhuang et al., 2016). As mentioned above, some previous studies have indicated that the impacts of insoluble fiber addition on water-holding capacity, emulsion stability and texture could depend upon the fat levels in meat emulsion system (Choi et al., 2015; Cofrades et al., 2000; Hughes et al., 1997). However, there has been little to no information on the efficacy of alkaline-treated insoluble fiber on meat emulsion with different fat levels. Therefore, the objective of this study was to determine the efficacy of alkaline-treatment for improving functional properties of sugarcane bagasse fiber on physicochemical and textural properties of meat emulsions at 5%, 10% and 20% fat levels.
Materials and Methods
Dried sugarcane bagasse was obtained from Audubon Sugar Institute (Baton Rouge, LA), which was dried in a 70°C oven for 2 d and ground using a commercial coffee grinder. Using 40-mesh screen, the triturated bagasse was sieved (crude sugarcane bagasse fiber, CSF) and alkaline-treated according to the procedure described by Kim et al. (2015). CSF was stirred with 10 volumes of distilled water (DW) containing 0.2 g of calcium hydroxide (CaO2, >96%) per g CSF at 90°C for 1 h. The calcium hydroxide-treated sugarcane bagasse slurry was centrifuged (Sorvall RC-5C Plus Centrifuge and SLA-1500 rotor, Kendro Laboratory Products, NC) at 4,500 rpm for 20 min, and then, the supernatant was discarded. The residual insoluble fraction was collected, washed with DW to neutralize the pH (6.2-6.8), hot-air dried (60°C) for 48 h, and used as alkaline-treated sugarcane bagasse fiber (ASF).
Meat emulsions were prepared by the manufacturing procedure described by Kim et al. (2015). Raw meat (M. biceps femoris) and back fat were obtained from commercially raised pigs at 2 d postmortem, and raw meat was ground through a 3/8 inch plate using a meat grinder (M-12-FS, Mexico). Control meat emulsion was formulated with 60% ground pork, 20% back fat and 20% ice, and six treatment emulsions were prepared using 3 (fat level; 5%, 10% and 20%)×2 (the types of sugarcane bagasse fiber; CSF and ASF) factorial design. The added amount of sugarcane bagasse fiber was fixed as 2%, which was a previously suggested level to anticipate the improved water-holding capacity of myofibrillar protein gel (Zhuang et al., 2017). The main ingredients were individually emulsified with the 2.0% NaCl, 0.3% sodium tri-polyphosphate, 120 ppm sodium nitrite and 500 ppm L-ascorbic acid in a bowl cutter (Cutter C4, Sirman, Italy). Excluding the meat batter portion for emulsion stability analysis, the meat emulsion was stuffed into an edible collagen casing (21 mm in diameter) and cooked in an 80°C water bath until reaching a core temperature of 71°C.
Moisture (method 934.01), protein (method 990.03), fat (method 920.39) and ash (method 942.05) contents of sugarcane bagasse fiber were analyzed by the official AOAC method (Horwitz and Latimer, 2000). Acid detergent fiber content of sugarcane bagasse fiber was analyzed by the filter bag method (Komarek, 1993), and other carbohydrates content was calculated by difference.
Water-binding capacity of sugarcane bagasse fiber was determined with the centrifuge method described by Auffret et al. (1994), and expressed as g of water per g of dry residue. Oil-binding capacity was measured with the method of Abdul-Hamid and Luan (2000), and expressed as mL of oil per g of fiber. Emulsifying capacity of sugarcane bagasse fiber was measured, based on the percentage difference between emulsified layer (mL) and total layer (mL) in vegetable oil-water-sugarcane bagasse fiber emulsion (Yasumatsu et al., 1972).
The morphological property of sugarcane bagasse fiber suspension (1% concentration in distilled deionized water) was observed using a light microscope at 15× and 100× magnifications (Kethireddipalli et al., 2002).
The pH value of homogenized meat emulsion (3 g of sample and 27 mL of DW) was measured using an electronic pH meter (Kim et al., 2015).
The proximate composition of cooked meat emulsion was determined by the same methods described above (Horwitz and Latimer, 2000). Calorie content was calculated according to the Atwater method (Watt and Mersil, 1975), in which energy values of fat, protein and carbohydrates were 9 kcal/g, 4.02 kcal/g, and 3.87 kcal/g, respectively.
To determine technological properties of meat emulsion, emulsion stability and cooking loss were determined. Emulsion stability was measured with the method described by Tahmasebi et al. (2016). Ten grams of meat batter was stuffed into a centrifuge tube and centrifuged at 2,500×g for 5 min (2°C). The tube was heated in a water bath (80°C) for 60 min, and cooled upside down at a room temperature for 45 min for the complete release of the exudates from meat emulsion. The total exudates were weighed, dried in a dry oven (100°C) for 16 h, and reweighed to determine the amount of fat within the exudates. Water and fat release were expressed as a percentage difference against the total weight of meat emulsion. Cooking loss of meat emulsion was evaluated from the percentage of weight difference between uncooked and cooked samples.
Textural properties of meat emulsion were evaluated using a TA-XT Plus Texture Analyzer (Stable Micro Systems Ltd., UK). The cooked meat emulsion was equilibrated to room temperature at 25°C for 3 h (Kim et al., 2015). Three samples (cylinder shape of 2.5 cm height and 2.5 cm diameter) were taken from the central portion of each meat emulsion. A twice compression cycle test (70% compression of the original sample height) was performed with an aluminum cylinder probe (TA-25). Sample deformation curves were obtained with a 50 kg load cell and the analysis condition were as follows: pre-test speed 1.0 mm/s, post-test speed 5.0 mm/s, test speed 5.0 mm/s. Value for hardness (N), springiness (ratio), cohesiveness, gumminess (N), and chewiness (N) were determined as described by Bourne (1978).
Statistical differences in chemical composition and functional properties between CSF and ASF (n=3) were determined using the Student’s t-test procedure of SAS (2008). For meat emulsion, an experimental design was a randomized complete block design with three independent batches (n=3). The data were analyzed using the one-way ANOVA procedure of SAS. Duncan’s multiple range test (p<0.05) was used to determine differences between treatment means.
Results and Discussion
Proximate composition of CSF and ASF is shown in Table 1. The proximate composition of sugarcane bagasse fiber was significantly affected by alkaline-treatment. ASF showed a higher moisture content (6.18 vs 5.09 g/100 g; p=0.004), but lower protein (3.59 vs 4.05 g/100 g; p=0.017), fat (0.27 vs 0.51 g/100 g; p=0.034) and ash (2.50 vs 4.63 g/100 g; p=0.002) contents compared to CSF. As a marked discrepancy in proximate composition, alkaline-treatment increased acid detergent fiber content, from 65.29 to 73.09 g/100 g dry matter (p=0.002). On the other hand, other carbohydrate content was decreased from 25.52 to 20.55 g/100 g dry matter (p=0.002). In general, acid detergent fiber includes lignocellulose and silica fractions (Font et al., 2003). Previously, Sangnark and Noomhorm (2003) reported that alkaline hydrogen peroxide treatment increased cellulose content of sugarcane bagasse but decreased hemicellulose and lignin. Kim et al. (2015) indicated that calcium hydroxide treatment increased acid detergent fiber content of insoluble soy hull fiber, resulting from the removal of proteinous components and non-fibrous carbohydrates. Thus, the increased acid detergent fiber content of ASF could be attributed to the elimination of acid-detergent soluble components (proteins and other carbohydrates) along with the degradation of lignin and hemicellulose.
Functional properties of sugarcane bagasse fiber, water-holding capacity, oil-binding capacity and emulsifying capacity, are shown in Fig. 1. Alkaline-treatment of sugarcane bagasse fiber greatly increased water-binding capacity (p=0.002), from 1.87 to 3.02 g of water/g of dry residue (Fig. 1A). However, ASF showed a lower oil-binding capacity (2.67 vs 4.83 mL of oil/g of fiber; p=0.028) and emulsifying capacity (25.83% vs 36.33%; p=0.011) compared to CSF (Fig. 1B and C). The decreased emulsifying capacity could be associated with the decreased oil-binding capacity. According to the Sosulski and Cadden (1982), dietary fiber rich in cellulose had high water-holding capacity, whereas dietary fiber rich in lignin exhibited better oil-binding capacity. Thus, the degradation of lignin due to alkaline-treatment could decrease oil-binding capacity and emulsifying capacity of sugarcane bagasse fiber. As an inconsistent result, Sangnark and Noomhorm (2003) previously reported that alkaline treatment with hydrogen peroxide increased both water-holding capacity and oil-binding capacity of sugarcane bagasse fiber. Moreover, they suggested that the decreased particle size (from >0.3 mm to < 0.075 mm) of alkaline hydrogen peroxide-treated sugarcane bagasse fiber linearly decreased water-holding capacity and oil-binding capacity (Sangnark and Noomhorm, 2003). In this study, the micrographs of 1% sugarcane bagasse fiber suspension (Fig. 2) showed visually distinct differences in particle size between CSF and ASF. ASF was comprised of the large numbers of relatively smaller and thinner particles (Fig. 2C and D) compared to CSF (Fig. 2A and B). Considering the different particle size between CSF and ASF, a further study evaluating functional properties at the same particle size would be performed.
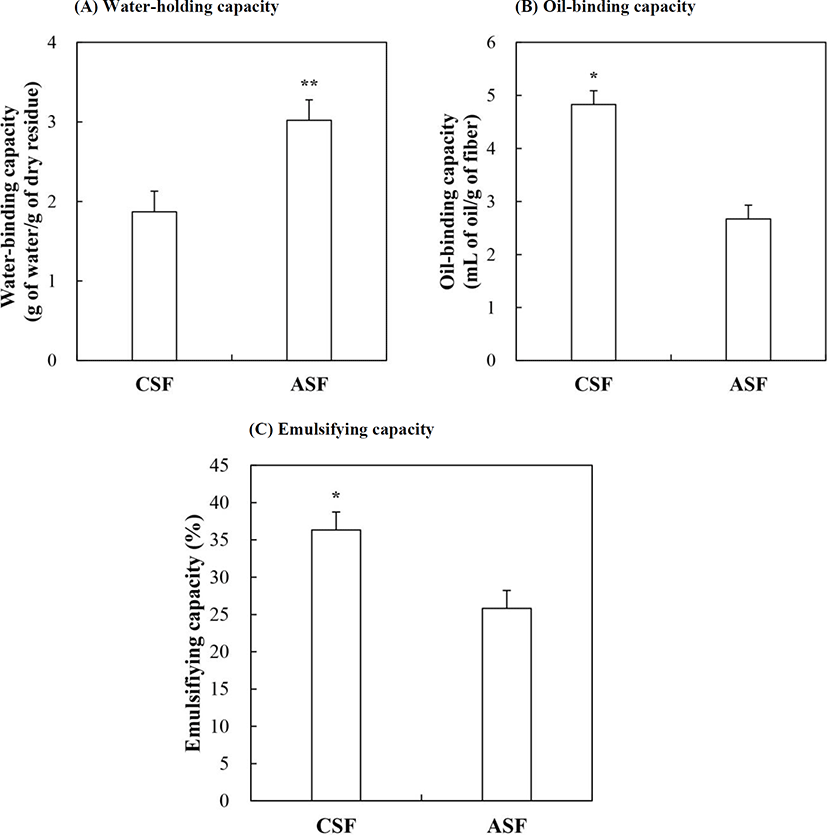
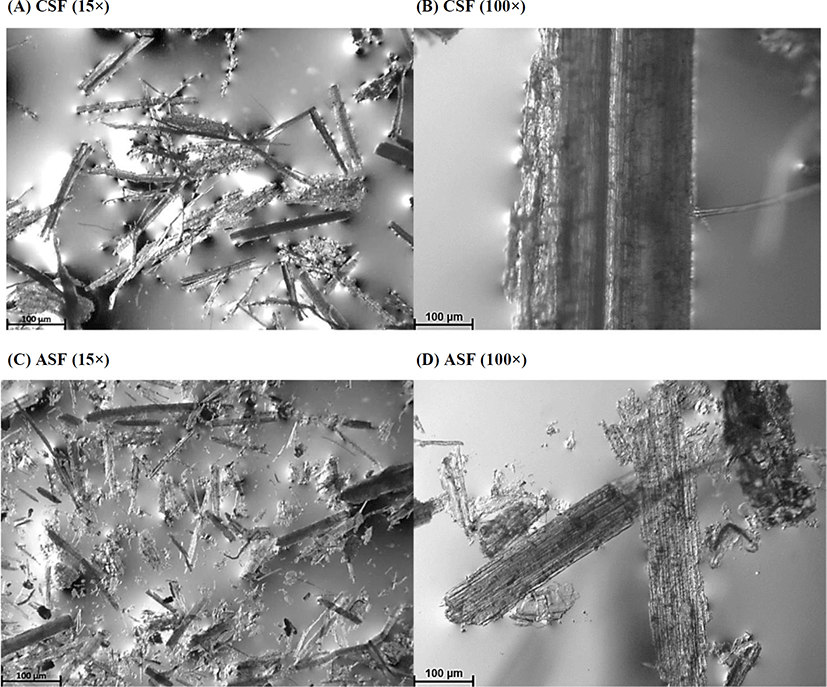
The effect of sugarcane bagasse fiber addition on physicochemical properties of meat emulsions prepared with 5%, 10% and 20% fat levels is shown in Table 2. Similar pH values (6.09-6.19) of cooked meat emulsions were found, regardless of the fat level and the type of sugarcane bagasse fiber (p>0.05). A replacement of back fat with additional water significantly increased moisture content, but decreased protein, fat, ash and calorie contents of the cooked meat emulsions formulated with sugarcane bagasse fiber. When compared to control meat emulsion, the calorie content of meat emulsions prepared with sugarcane bagasse fiber was reduced to 15.5-17.2% at 10% fat level and to 25.8-30.3% at 5% fat level, respectively. When back fat is substituted with additional water in meat emulsion, an increase in moisture content but decreases in fat and calorie contents have been generally found from previous studies (Choi et al., 2010; Grigelmo-Miguel et al., 1999).
However, the addition of sugarcane bagasse fiber unaffected proximate composition and calorie content of cooked meat emulsion prepared with 20% fat level (p>0.05). In a previous study, Zhuang et al. (2016) reported that the addition of pre-emulsion containing 1-3% sugarcane dietary fiber increased moisture and ash contents but decreased fat and protein contents of meat emulsion formulated with 10% pork fat and 10% sesame oil. Such inconsistent result was likely due to a different formula of ingredient such as back fat and vegetable oil. At the same fat levels, furthermore, the types of sugarcane bagasse fiber had no impacts on proximate composition and calorie content of cooked meat emulsions (p>0.05). With the 2% inclusion of sugarcane bagasse fiber, thus, the alkaline-treatment had no impacts on proximate composition of cooked meat emulsion.
The effect of sugarcane bagasse fiber addition on emulsion stability and cooking loss of meat emulsions prepared with 5%, 10% and 20% fat levels is shown in Table 3. The replacement of back fat with additional water caused significant increases in water and fat releases from meat emulsion. This phenomenon has been generally observed from previous studies, resulting from a reduction in water-holding capacity and emulsion stability of meat emulsion with excessive water (Choi et al., 2010; Zhuang et al., 2016). In addition, the inclusion of insoluble dietary fiber could contribute to improve water-holding capacity and oil-binding capacity of meat emulsion, contributing to an increase in emulsion stability (Choi et al., 2010; Kim et al., 2015). A previous study indicated that insoluble sugarcane dietary fiber, as a fat replacer, could enhance emulsion stability in low-fat meat emulsion (Zhuang et al., 2016). In this study, the type o/fablesugarcane bagasse fiber had no impacts on emulsion stability (water and fat releases) of meat emulsion with the same fat levels of 10% and 20%, respectively. At 5% fat level, however, the meat emulsion with ASF had a higher fat release than that with CSF (p<0.05). This result could be associated with the decreased oil-binding capacity and/or emulsifying capacity of ASF, together with unstable emulsion stability due to a high amount of additional water substituting for back fat. In terms of cooking loss, the addition of sugarcane bagasse fiber to meat emulsion formulated with 5% or 10% back fat could contribute to similar to control meat emulsion formulated with 20% back fat without sugarcane bagasse fiber (p>0.05). This result could be due to the hydration and/or oil-binding capacities of sugarcane bagasse fiber. However, the type of sugarcane bagasse fiber had no impact on cooking loss when meat emulsions formulated with the same fat level (p>0.05).
The effect of sugarcane bagasse fiber addition on textural properties of meat emulsions prepared with 5%, 10% and 20% fat levels is shown in Table 4. The addition of sugarcane bagasse fiber significantly increased hardness of meat emulsion at 20% fat level. As a similar result, Zhuang et al. (2016) reported that the inclusion of insoluble sugarcane dietary fiber (1-3%) increased hardness, gumminess and chewiness of low-fat meat emulsion prepared with 10% pork fat and 10% sesame oil. Moreover, Zhuang et al. (2017) indicated that the addition of 2% sugarcane fiber could improve the gel strength of myofibrillar protein gel, thereby changing water distribution. Previously, it has been generally found that a reduction in animal fat reduces all parameters of textural properties in meat emulsion, such as hardness, springiness, cohesiveness, gumminess and chewiness (Cofrades et al., 2013). In this current study, meat emulsion formulated with 10% fat and sugarcane bagasse fiber exhibited a slightly lower hardness (p<0.05) but showed no differences in gumminess and chewiness (p>0.05), when compared to control meat emulsion. Similarly, Zhuang et al. (2016) found that low-fat meat emulsion with 10% pork fat and 2% sugarcane fiber could cause equivalent textural properties to control meat emulsion prepared with 30% pork fat. The springiness and cohesiveness of meat emulsions were unaffected by both of fat level and the type of sugarcane bagasse fiber (p>0.05). Thus, the addition of sugarcane bagasse fiber could contribute to improve the textural properties of low-fat meat emulsion due to its functional properties such as hydration properties and/or emulsifying capacity (Choi et al., 2009; 2010).
Conclusion
The findings from this current study indicate that alkaline-treatment could improve hydration properties of sugarcane bagasse fiber, but reduce oil-binding and emulsifying capacity per se. The addition of 2% sugarcane bagasse fiber could allow reduced-fat meat emulsion (10% fat level) to have equivalent proximate composition, cooking loss and textural properties to control meat emulsion (20% fat level), along with 15.5-17.2% calorie reduction. This confirms that sugarcane bagasse fiber could be a functional food ingredient to produce low-fat meat products. However, the results of this study indicate that the altered functional properties of alkaline-treated sugarcane bagasse fiber (2% addition level) had no impacts on physicochemical and textural properties of meat emulsion formulated with 5%, 10% and 20% fat levels. Further studies determining the effects of particle size of alkaline-treated sugarcane bagasse fiber on technological properties of reduced-fat meat emulsion should be warranted to assess its efficacy for practical use as a fat replacer in emulsified meat products.