Introduction
Meat tenderization occurs largely via endogenous proteolytic enzyme system(s) including the calcium-dependent sarcoplasmic cysteine-proteases calpains (Panduraman and Hwang, 2003). When meat is treated with calcium ions, myofibrillar proteolysis takes place and breaks down the Z-line proteins titin and nebulin (Hopkins, 2014). Myofibrillar and sarcoplasmic proteins undergo autolysis, forming small peptides and free amino acids (Sentandreu et al., 2002; Toldrá and Flores, 1998). These peptides and amino acids are key determinants of both palatable meat taste and flavor via generation of volatile compounds through Maillard reactions and Strecker degradation (Mottram, 1994).
The activity of the calpain system depends on calcium ion concentration (Bunmee et al., 2014) and infusing or injecting meat with calcium salt solutions has a positive effect on tenderness (Lawrence et al., 2003; Rousset-Akrim et al., 1996; Taylor et al., 1995) and flavor ratings (Bunmee et al., 2014; Diles et al., 1994). The calcium ion treatment enhances calpain autolysis while zinc ion treatment inhibits calpain autolytic activity (Ilian et al., 2004). On the other hand, enhancement of calcium, zinc and/or metal ions may decrease the product shelf life and affect oxidative quality, perceived as meat discoloration and off-flavor mainly due to protein and lipid oxidation (Hopkins, 2014; Kerth et al., 1995).
Two theories, though controversial, have proposed that both the calpain system and cathepsins are responsible for postmortem protein degradation and tenderization (Calkins and Seidman, 1988; O’Halloran et al., 1997; Taylor et al., 1995). On the other hand, a large number of scientists (Huff-Lonergan, 2014; Koohmaraie et al., 1989; Uytterhaegen et al., 1994) have reported that the calpain system is the only endogenous enzyme responsible for postmortem tenderization.
It has consistently been shown that the generation of lower molecular weight flavor precursor compounds such as small peptides and amino acids is greatly influenced by postmortem proteolysis in muscle tissue (Ba et al., 2014; Spanier et al., 1997; Toldrá and Flores, 1998). We hypothesized that elevated calpain activity with calcium ion supplementation would enhance the generation of volatile flavor compounds compared to meats with no cathepsin activity. In particular, increasing amounts of lower molecular weight fragments would increase the quality and quantity of volatile compounds and consequently influence the palatability of cooked beef. To the best of our knowledge, there is little scientific information regarding the volatile compounds of Hanwoo beef as a function of the activity of the calpain system. The current study was designed to identify the effect of the calpain system on the quality characteristics and volatile flavor compounds of Hanwoo Longissimus lumborum (LL) muscle.
Materials and Methods
Korean native cattle Hanwoo steers (n=4) were conventionally slaughtered at the age of 28 mon and processed at commercial meat plants according to normal practice. After chilling 24 h, M. longissimus lumborum were sampled from carcasses with quality grade 1, vacuum packaged and immediately transferred to the laboratory. Two consequent experiments were designed to identify the effect of the calpain system on the formation of volatiles. The first experiment was conducted on the LL muscle samples that were injected with a solution containing calcium, zinc or sodium ions (control) and then incubated (exp.1), while a second experiment was done with powdered muscle tissue incubated with salt solutions containing cathepsin inhibitor (exp.2).
For the first experiment, each LL was divided into three equal portions of approximately 250 g. Randomized portioned samples were injected with one of the reaction solutions (50 mM CaCl2, 50 mM ZnCl2 or 154 mM NaCl, pH 5.6). The solutions were injected at volumes of 10% (w/w) of the muscle weight by multiple arranged syringes. The solutions were applied to the steaks in vacuum bags to observe surface penetrating process. Injected samples were soaked without agitation until a 10% added weight was achieved. Injected samples were vacuum- packed and aged at 4°C for 7 d. The aged samples were assessed for pH, color, tenderness, cooking loss, protein degradation, fatty acid composition and volatile compounds.
The injection yield and drip loss were determined by comparing the weight of the sample after injection and after aging respectively to the weight of the raw meat (Diles et al., 1994).
For the second experiment, the LL muscles were powdered in liquid nitrogen using a mini-grinder and kept at -80°C until use. Approximately 5 g of the powdered sample was incubated with one of cocktail solutions (50 mM CaCl2, 50 mM ZnCl2 or 154 mM NaCl, respectively) containing cathepsin inhibitors, Chymostatin and Z-FF-FMK, in a ratio of 1:3 (w/v). The salt/cathepsin inhibitor cocktail was designed to provide a favorable environment for the proteolytic activation of the calpain system while preventing the activation of cathepsins. The mixtures were incubated for 7 d at 4°C in a mild shaker. At the end of the reaction, 100 mM EDTA was added to stop proteolysis, and the samples were analyzed for protein degradation, volatile compounds and fatty acid composition. In all experiments, there were four replicates of each treatment.
The pH was determined in quadruple using an Orion 3 Star pH meter (Thermo Electron Corp., USA). Meat color, cooking loss and WBSF were determined on the same sample steaks (approximately 200 g each) by using method of Dashdorj et al. (2012). Meat color evaluation was done using a Konica Minolta Spectrophotometer CM-2500d (Sinodevices Group, Japan) with an 8-mm measuring port, D 65 illuminant and 10° observer. Three measurements were acquired on the bloomed surface of the meat. After the color measurement, the steaks were immediately used for measurements of cooking loss. Each sample was immediately placed in a plastic bag and heated in a water bath (maintained at 70°C) for 1 h until the core temperature had reached 70°C. The cooked samples were immediately cooled under a running tap water for 30 min. The excess moisture was removed and samples were reweighed to determine cooking loss percentage. Cooked steaks designated for WBSF analysis stored overnight at 4°C for chilling. The WBSF values were measured in an Instron Universal Testing Machine (Model 3342, Instron Ltd., USA) on six replicate core samples with a 0.5-inch diameter probe, a crosshead speed of 400 mm/min and a 40-kgf load cell.
SDS-PAGE was performed by the method of Laemmli (1970) with slight modification. The protein concentration of the sample was adjusted to 2 mg/mL with SDS-PAGE sample buffer (2× treatment buffer, 2-mercaptoethanol, bromophenol blue; pH 6.8), and then heated to 95°C for 5 min. The heated samples (10 µL each) were loaded into wells of 4% Stacking Gel and a 12.5% Separating Gel. A protein broad range marker (BIO-RAD, USA) was also used. A mini SDS-PAGE electrophoresis system (20-cm mini gel) was used and run at 100 V until the dye reached the bottom of the gel. After running the protein on the gel was transferred to a 0.2-μm PVDF (polyvinylidene fluoride) membrane at 200 mA for 60 min in transfer buffer (10% methanol, 192 mM glycine and 25 mM Tris). Subsequently, the membrane was blocked using 5% skim milk at room temperature for 1 h. The membrane was incubated with anti-troponin-T monoclonal primary antibodies (1:2,500 in TBS-T (Tris buffered saline containing 0.05% Tween-20), (JLT-12, Sigma-aldrich, USA) and µ-calpain (1:1,000 in TBS-T) (Sigma-aldrich, USA) for 1 h. The bound primary antibodies were labeled with mouse IgG alkaline phosphatase-conjugated secondary antibody (diluted 1:2,500 in TTBS) (Sigma-aldrich, USA) for 1 h. The bound antibodies were visualized using an enhanced chemiluminescence (ECL) kit (Thermo Scientific, USA) and then images were acquired with a Versa Doc 3000 imaging system (Bio-Rad, USA).
Direct transesterification of fatty acid was performed with the procedure developed by Rule (1997). Gas chromatography (GC-2014, Simadzu, Japan) with Flame ionization detector (FID), was used to separate the fatty acid components in the samples. Chromatographic separation of components was carried out on a FAMEWAX Capillary column (30 m×0.32 mm×0.25 μm; Restek Corporation, USA). The flow rate was 0.7 mL/min and the split ratio used was 30:1. N2, H2 and air were used as carrier gases. The inlet temperature was 250°C and the oven temperature program was as follows: 50°C for 1 min, raise the temperature to 200°C at a rate of 25°C per min, further increase the temperature to 240°C at 3°C per min then the temperature was held at 240°C for 15 min. The detector temperature was 250°C. The individual fatty acids were identified by comparing their retention times with those obtained from fatty acid standards (Marine Oil FAME Mix, Bellefonte, USA), and finally expressed as a percentage of the total fatty acids.
The volatile compounds in the cooked samples were extracted using solid phase micro-extraction (SPME) and analyzed by GC/MS (6890N Network GC System, Agilent Technologies, USA) equipped with 5973 Network Mass Selective Detector (Agilent Technologies, USA) following the method of Ba et al. (2014). The samples were powdered in liquid nitrogen using a mini-grinder. A 1-g aliquot was placed in 40-mL headspace vials and sealed with a PTFE-faced silicone septum. The vials were then heated at 121°C for 20 min in an autoclave, cooled at room temperature and immediately used for the analysis. Samples were extracted using SPME. The volatile compounds were identified by comparing their mass spectra with the Wiley Registry of Mass Spectral Data (7th edition), and/or by running a number of commercial standards. The internal standard 2-methyl-3-heptanone was used for quantification.
The data were analyzed using a two-way analysis of variance. Using SAS PROC GLM (SAS Institute, USA), we assessed the main effects of the variables in the two experiments and injection treatments in regard to their objective quality traits and volatiles. The means of the measurements were compared using Duncan’s multiple-range test at a significance level of 0.05.
Results and Discussion
Meat quality traits and yield of the LL muscles injected with calcium, zinc and sodium chloride are shown in Table 1. There was no significant difference in injection yield. The mass of the injected samples was slightly lower after 7 d aging (by 1.86% for calcium and by 0.08% for zinc compared to their initial weight). These results were similar to those of Bunmee et al. (2014) who showed that injecting a 10% calcium salt solution did not affect drip loss; however, theoretically, the ions could promote moisture loss because of the change in ionic strength. Moreover, Jaturasitha et al. (2004) reported that drip loss was not affected by CaCl2 concentration.
Other researchers did not recommend pre-mortem injection of CaCl2 because of adverse effects on the flavor, appearance and texture of the meat, specifically, toughening (Rousset-Akrim et al., 1996). Pre-rigor enhancement with ionic compounds can have a dramatic effect on the rates of glycolysis and muscle contraction as well as proteolysis and the oxidative process, whereas post-rigor injection affects mainly proteolysis and oxidative processes (Hopkins, 2014). Accordingly, we injected LL muscles at 24 h postmortem and samples aged for 7 d. The current data was shown that both calcium and sodium chloride treatments resulted in more tender meat (p<0.05) than samples injected with zinc chloride. Zn2+-treated muscles had significantly higher (p<0.05) WBSF values (3.7 kgf) than those injected with CaCl2 (2.02 kgf) or the control (2.7 kgf). This result was similar to that of Ilian et al. (2004), who reported that the shear force values of Zn2+-treated lamb muscles were significantly higher than those of the control or water-treated meat, and infusion with 50 mM ZnCl2 inhibited the rate of tenderization. An introduction of exogenous solutions containing ions such as Ca2+ or Na+ has been found to impacts the tenderization of meat because the action of Ca2+ is strongly linked to the activation of the calpains, whereas, Na+-based solutions can lead to solubilization of proteins, thus improving tenderness (Hopkins, 2014).
There were no significant differences in pH, color and cooking loss among the injected treatments. This is likely associated with the low concentration of salt solutions (i.e., 50 mM). Previous studies found that a low level of ion supplementation had limited effects on meat quality (Morris et al. 1997; Rousset-Akrim et al., 1996), while 150 mM CaCl2 increased juiciness and 200 mM CaCl2 improved sensory traits of meat (Dile et al., 1994). On the other hand, sour flavor and metallic off-flavor were detected with 300 mM and 400 mM CaCl2 injections (Dile et al., 1994; Morgan et al., 1991) respectively, it was probably due to the fact that calcium ions can stimulate lipoxygenase activity and the mitochondrial respiration process leading to a higher-rate oxidative process (Hopkins, 2014).
The postmortem proteolysis patterns in LL muscle as affected by the ions injection in the exp.1 and exp.2 are shown in Figs. 1-3. By using SDS-PAGE technique, it was observed that the protein degradation patterns of postmortem proteolysis differed depending on the treatments (e.g., activation or inhibition). In exp.1, though most protein bands appeared in all the injected samples (CaCl2 or ZnCl2 and NaCl), however, some bands with same molecular weight (as marked in Fig. 1) showed more density for the control samples (injected with NaCl) whereas, some bands showed less density (slightness) in the CaCl2 injected samples. This meant that the proteins were partly degraded by the muscle proteases as enhanced by the CaCl2 injection. Regarding this, previous researchers also have indicated that protein degradation can be partly attributed to the acid lysosomal cathepsins (Toldrá and Flores, 1998) and cytosolic neutral calcium-activated calpains (Koohmaraie, 1996), both of which are known to play a key role in postmortem proteolysis and contribute to tenderness during meat aging (Geesink et al., 2006). On the other hand, the troponin-T degraded product (30 kDa) was not found in the ZnCl2 injected samples but appeared in the samples treated with CaCl2 or NaCl (Fig. 2). The results signified that the injected Zinc potently inhibited the troponin-T degradation by cysteine proteinases, which agreed with finding of (Whipple and Koohmaraie, 1991).
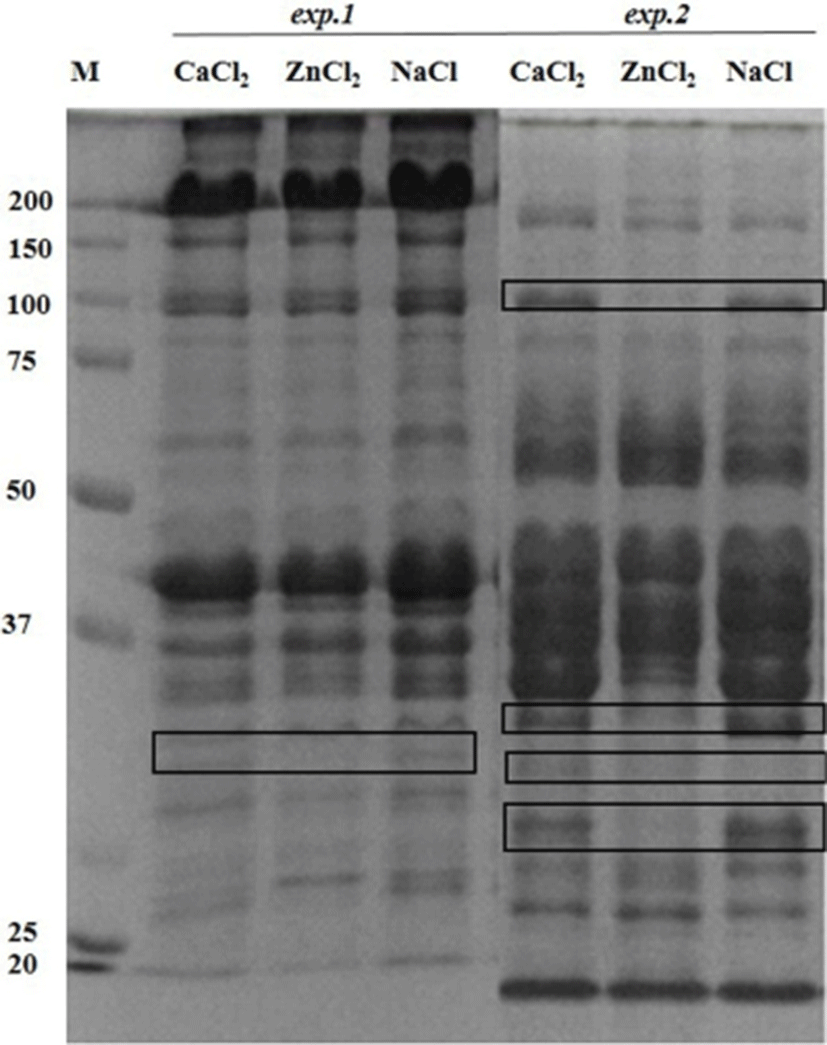
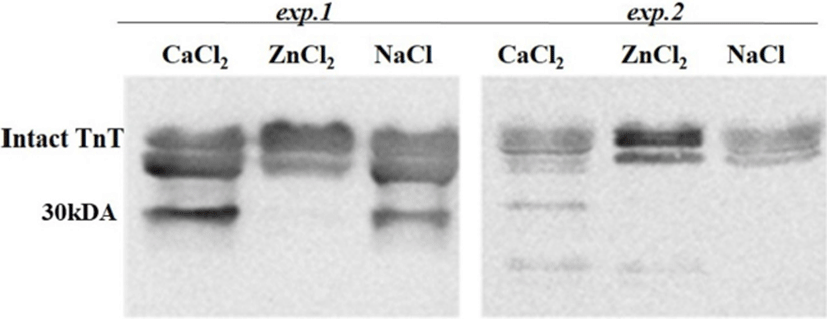
Regarding the exp.2 where the proteases inhibitors were used and the clear effects of the injected ions on the proteins degradation was observed. From the SDS-PAGE results, it was observed that some of the important changes consisted of a disappearance of numerous proteins in the ranges of 100 kDa and 30-34 kDa in Zn2+-treated muscle (Fig. 1). Moreover, around 80 kDa, a smear band was visible from an undegraded form of calpain in the ZnCl2 treated samples (Fig. 3). From these results it could be said that the proteolytic activities of proteases (e.g., calpain and capthepsins) were significantly inhibited by ZnCl2 and inhibitors treatment. It is well recognized that zinc is a strong inhibitor of both calpains and cathepsins and its availability maintains cell structure and delays oxidative processes, thus ZnCl2 injection into beef have also been proven to block post mortem proteolysis and the tenderization (Koohmaraie et al., 1990). The ion also inhibits troponin T degradation and the same concentration of ZnCl2 caused no detectable solubilization of myofibrillar proteins (Taylor et al., 1991).
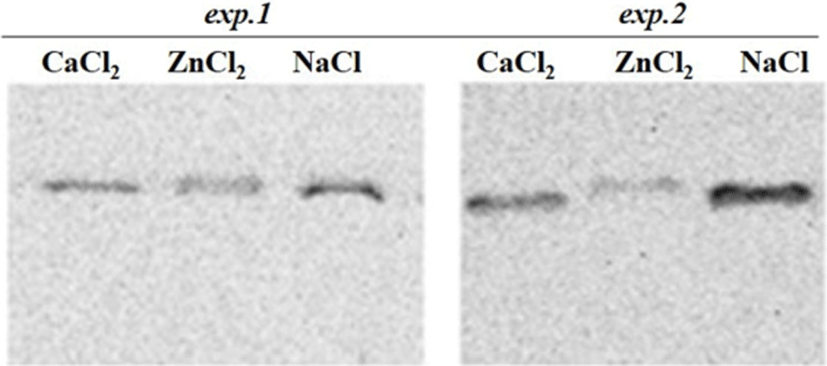
Unlike cathepsins, the calpains intensively degrade the majority of proteins into relatively few fragments (Huff-Lonergan, 2014). When calpain is activated, the 80-kDa catalytic subunit progressively autolyses to a 78 kDa protein, then the 76 kDa form (Goll et al., 2003). In the exp.2, calpain activity led to a greater concentration of troponin T degradation product (30 kDa) than the exp. 1 (Fig. 2). The troponin T breakdown product is a well-known indicator of tenderness (Lawrence et al., 2003). The appearance of the 30 kDa degradation fragment of the intact troponin-T has been demonstrated to be an indicator of muscle tenderization (Huff-Lonergan, 2014). In addition, a 28 kDa (actin) subunit of the enzyme is reduced to 18 kDa (myosin light chain) in exp.2 (Fig. 2).
Previous works showed that calpains are responsible for up to 95% of all proteolytically-induced postmortem tenderization that occurs during first 7–14 d of aging (Panduraman and Hwang, 2013). Even if the calpain system is responsible for most of the changes that occur during tenderization, the contribution of cathepsins cannot be ignored (Huff-Lonergan, 2014). Cathepsins were originally of interest as one of the major sites of protein degradation in lysosomes of living tissue, but time of postmortem aging has been shown to significantly affect cathepsin B+L activity (Panduraman and Hwang, 2013). Thus, from the protein degradation induced by calcium ions we expected it could result in formation of low molecular weight products such as small peptides and free amino acids, which are known precursors of flavor.
A list of volatile compounds identified in cooked Hanwoo beef using the SPME-GC method is reported in Table 3. All 51 volatile compounds identified in both experiments can be divided into two groups: those produced by Maillard reactions and those produced by lipid oxidation/degradation. Volatiles such as sulfur- and nitrogen-containing compounds, Strecker aldehydes and furans are derived from the Maillard reaction during heating; aldehydes, alcohols, ketones and hydrocarbons are derived from lipid oxidation/degradation (Ba et al., 2012; Elmore et al., 2002). Quantitatively, the most significant changes were observed for aldehydes, which were major volatiles in both experiments, followed by pyrazines and sulfuric compounds.
The highest total amount of volatile compounds was observed in the samples treated with NaCl (6.7 µg/g), followed by ZnCl2 (6.0 µg/g), and CaCl2 (5.5 µg/g), in exp.1, whereas amounts were slightly lower in exp.2 (4.5, 6.5 and 3.6 µg/g, respectively). Quantities of 12 volatile compounds significantly differed between the two experiments (Table 2). The total amount of volatiles derived from the Maillard reaction (pyrazines+furans+sulfuric) were greater (p<0.05) in exp.1, whereas, aldehydes (hexanal, benzaldehydes, benzeneacetaldehydes, decanal, undecanal, E,E-2,4-decadienal and 2-undecenal), hydrocarbons, decanes and alcohols (2-furanmethanol and 1-octen-3-ol) derived from lipid oxidation were greater (p<0.05) in samples with blocked cathepsin activity (exp.2). The results of the exp.1 also showed that amount of total furans (pentylfuran, hexylfuran, heptylfuran and octylfuran), heptanal and toluene were greatly (p<0.05) increased by endogenous proteinases (p<0.05). The furans with sweet, caramelic notes were produced generously from the Maillard reaction between reducing sugars and amino acids. Sugars and amino compounds react to form a Schiff base, which is eventually converted to an Amadori compound (Farmer, 1999).
In this study, unlike the cathepsins, Ca2+-activated calpains degraded the majority of proteins into relatively low molecular fragments that involved the formation of volatiles. However, endogenous cathepsin proteolytic activity also increased the total amount of many volatiles including furans, acetaldehydes, 2-decenal, hexadecanal and 2-propanone. Moreover, the volatiles with lower linear retention indices (LRI, ≤800) such as; 2- and 3-methylbutanal, furfural, dimethylsulfide, 1-pentanol, 2,3-butanedione, 2-propanone, heptanes, 2,4-dimethylheptane and 3-thiophenemethanol, were not detected in exp.2, but were detected in exp.1. The differences could have stemmed from the activity of other enzyme systems, or synergistic action of the calpains and cathepsins. The lysosomal cysteine cathepsins have been implicated in the production of flavor peptides (Spanier et al., 1990), and this likely play an important role in the temporal generation of flavor in meat during aging. Although Calkins and Seidman (1988) reported that free cathepsin activity (B, H and L) is correlated with meat tenderness from day 1 until the end of the aging period, the cathepsin system functions optimally at an acidic pH, and longer postmortem aging involves some catheptic proteolysis (Panduraman and Hwang, 2013). On the other hand, other researchers have suggested that the structural changes of muscle protein cannot be explained by the action of one proteolytic system, a synergistic action of the proteases must be considered (Quali, 1992; Sentantreu et al., 2002). For example, Huff-Lonergen (2014) showed that serine proteases and the proteasomes may have a role in postmortem proteolysis.
The effect of calcium-induced calpain activity on the generation of volatile compounds is presented in Table 2. The results showed that the injection (50 mM CaCl2, 50 mM ZnCl2 and 154 mM NaCl) significantly (p<0.05) affected the concentration of 23 volatile compounds. Among these, pyrazines such as; methylpyrazine, dimethylpyrazine, 2-ethyl-2,5-dimethylpyrazine, and sulfuric compounds (e.g., dimethyldisulfide and 2-acetylthiazole) were greater (p<0.05) in the Ca2+-enhanced samples than the control samples in both experiments. However, these compounds were detected in higher amounts in the control (NaCl) samples than the ZnCl2 injected-samples. Strecker aldehyde 2-methylbutanal was detected in the calcium-injected samples and in the control but it was not detected in Zn2+-treated samples in exp.2.
Among the thousands of volatile compounds identified in meat (Shahidi et al., 1994), however, the sulfur-containing compounds and carbonyl compounds are principal contributors to the desirable meat flavor (Ba et al., 2013; Shahidi et al., 1994). The Strecker aldehydes 3-methylbutanal and 2-methylbutanal were with meaty, oily notes (Machiels et al., 2003). In this study, the greater extent of formation of these compounds was probably due to the greater levels of the free amino acids (e.g., isoleucine and leucine), which were released during protein degradation. The amino acids released during meat aging may produce intermediate products such as sulfur radicals, nitrogen radicals and carbonyls; these compounds undergo Strecker degradation, which can further react with each other or interact with other compounds to yield volatiles such as pyrazines and thiazoles via the Maillard reaction (Ba et al., 2013; Mottram, 1994). Sulfur-containing compounds with low odor detection threshold can be produced from the Maillard reaction between the reducing sugars (e.g., ribose) and the sulfur-containing amino acids methionine, cysteine and cystine (Koutsidis et al., 2008). Otherwise, non-sulfur containing amino acids react with sugars and produce nitrogen-containing products such as pyrazines with a roasted flavor (Mottram, 1994). Thus, this information implies that greater activity of calcium-activated enzymes leads to more extensive protein degradation, more free sulfur-containing amino acids, more Maillard reaction products and more volatiles.
The specific actions of each endogenous proteinase resulting in flavor formation in meat have not been characterized, though some mechanisms have been identified. For example, previous researchers noted that proteinases hydrolyze the muscle fibers, causing a disruption of muscle integrity (Alarcon-Rojo and Dransfield, 1995). The resulting intermediate-sized polypeptides and small peptides are subsequently degraded into free amino acids that can act as reactants in the Maillard reaction and Strecker degradation (Toldrá and Flores, 1998). As such, these reactants form volatiles in cooked meat. The specific proteinases have been identified as cathepsins B, D, H, and L and, to a lesser extent, calpains, as well as exopeptidases (i.e., peptidases and aminopeptidases) in dry cured ham (Toldrá and Flores, 1998).
Besides promoting Maillard compounds formation, CaCl2 also significantly increased (E,E)-2,4-decadienal, tridecanal, 1-pentanol and 2,4-dimethylheptane. (E,E)-2,4-decadienal with fatty note was likely formed during the thermal degradation of linoleic and linolenic acid (Ba et al., 2013; Elmore et al., 2002). With regard to alcohols, 1-pentanol and 2,4-dimethylheptane could have been formed from the thermal oxidation/degradation of lipids.
In addition, in the present study, 2-methylbutanal, octylfuran and sulfuric compounds were not detected in the ZnCl2-treated samples in exp.2. Interestingly, most of the aldehydes (i.e., hexanal, (E)-2-decenal, benzaldehyde, benzacetaldehyde, nonanal, octanal and undecenal) and the alcohols (furanmethanol, 1-heptanol, 1-octen-3-ol and octanol), formed as a result of lipid oxidation were detected in greater (p<0.05) amounts in exp.2 than in exp.1, especially in the Zn2+-treated samples. One possible explanation for this is that the use of chloride salts could affect the activity of lipolytic enzymes, changing the fatty acid profile and consequently affecting the flavor compound profile (Table 3). Ripollés et al. (2011) noted that inhibition of acid lipase was more pronounced with CaCl2 than with NaCl.
Like proteases, lipases are very important enzymes involved in meat flavor development. The unsaturated fatty acids are readily oxidized and they may initiate the free radical oxidation of more saturated acids and increased concentrations of breakdown products (Ba et al., 2013; Elmore et al., 2002). Free fatty acids are generated by the action of lipases and transform into volatiles as a result of oxidation/degradation during cooking (Toldrá and Flores, 1998; Vestergaard et al., 2000). Researchers also revealed that some by-products generated by lipid oxidation including conjugated dienes, carbonyls (ketones and aldehydes), alcohols and hydrocarbons can cause a rancid odor and off-flavors (Min and Ahn, 2005). These undesirable effects result from the decomposition of lipid oxidation products when further reactions with metal ions occur (Bekhit et al., 2013).
The present study also revealed that aldehydes were the most predominant among the detected flavor compounds in cooked Hanwoo beef. They presumably are the principle contributors to the sweet, green, roasty, fruity and especially fatty flavor notes characteristic of Hanwoo beef with high fat content. Hexanal and heptanal, which are associated with pleasant sweet, fatty flavors, come from oxidation of oleic acid; benzaldehyde is associated with the unpleasant bitter almond-burning odor notes is derived from oxidized linolenic acid (Ba et al., 2013; Elmore et al., 2002). Octanal, nonanal and (E)-2-decenal are produced from the oxidation of the oleic acid, which is the dominant fatty acid in Hanwoo beef (Ba et al., 2013).
Present study reports for the first time the effect of the calpain system on the formation of volatile compounds in cooked beef. These data imply that the endogenous proteolytic system in postmortem meats influences the flavor characteristics of cooked meat and, consequently, control of the calpain system is an important factor in the optimization of consumer acceptability of meat.
Conclusion
The current data demonstrate that Ca2+-induced calpain plays a major role in beef tenderization and involves formation of important favorable volatiles such as pyrazines and sulfuric volatile compounds of cooked beef. An increased level of some aldehydes likely generated from lipid oxidation occurred with enhancement by ZnCl2. In addition to the inhibition of postmortem calpain proteolysis, a ZnCl2 solution may also affect the activity of lipolytic enzymes.