Introduction
Over the past few decades, consumers’ health concerns have increased leading to growth in the health food and nutraceuticals markets (Sun, 2008; Utama et al., 2018). In the muscle food sector, the demand for healthy meat products, including low-fat, low-calorie, low-salt, and functional ingredient-added (e.g. fiber-fortified) meat products have also increased (Olmedilla-Alonso et al., 2013).
The intake of dietary fiber-rich foods reduces the incidence of conditions such as obesity, cardiovascular, and coronary heart disease (Johnson and Southgate 1994; Lairon et al., 2005; Pereira et al., 2004). In meat products, fiber plays an important role that enhances cooking yield and emulsion stability due to its water and fat binding abilities, as well as its textural properties (Choe et al., 2013; Cofrades et al., 2000). A number of studies have reported that the addition of fiber sources, including grains, vegetables, and plants, improves the functional properties various of meat products (Kim, 2013; Kim and Kim, 2017; Lee et al., 2008; Mansour and Khalil, 1997).
Buckwheat (Fagopyrum esculentum) contains 30% total dietary fiber (approximately 26.5% insoluble and 3.0% soluble dietary fiber), significant levels of essential amino acids, polyunsaturated fatty acids, and vitamins B and E (Lee et al., 1995; Lin et al., 2009). In addition, buckwheat possesses functional properties that include anti-oxidant, anti-inflammatory, and anti-carcinogenic properties due to the high levels of rutin and other flavonoids that are key components of buckwheat (Baumgertel et al., 2003; Lin et al., 2009). In particular, the anti-obesity properties of buckwheat are well known to reduce low-density lipoprotein (LDL) and cholesterol levels (Choy et al., 2013). However, to the best of our knowledge, only a limited number of studies on the application of buckwheat as a functional material in meat products have been published (Bejosano and Corke, 1998). Therefore, the objective of this study was to determine the effect of buckwheat powder on the physicochemical characteristics of sausages including proximate composition, pH values, instrumental color, cooking yield, viscosity, texture profile analysis (TPA), and sensory evaluation.
Materials and Methods
Ham (M. semitendinosus and M. semimembranosus) muscle from fresh pork and back fat were purchased from a local market (Hongjumeat, Chungnam, Korea), 48 h postmortem. The subcutaneous and intramuscular fat and visible connective tissue were removed from the fresh ham muscle. The sausages were produced to the following formulation: 50% pork meat, 30% pork back fat, 20% ice, 1.2% nitrite pickled salt (salt:nitrite=99.4:0.6), ground 1% sugar, 0.6% mixed spice, and buckwheat powder [0 (control), 1, 2, and 3%, respectively]. The sausage manufacture for three batches was performed in the different days. For each batch, lean pork meat and fat were ground through a 3-mm ground plate (PA-82, Mainca, Spain). The fat and additives were emulsified using a bowl cutter (K-30, Talsa, Spain). In addition, buckwheat powder was added to all samples, excluding the control. The meat batter was stuffed into natural casings and the meat samples were cooked in a chamber (10.10ESI/SK, Alto Shaam, USA) at 85±1°C until their core temperatures reached 75°C. The cooked sausages were rested at 10°C for 30 min and then stored at 5°C until analyzed.
The proximate composition of each sample was analyzed according to the AOAC (2012) standard. Moisture content (AOAC method 950.46B) was determined by weight loss after 12 h at 105°C in a drying oven (SW-90D, Sang Woo Scientific Co., Bucheon, Korea). Fat content (AOAC method 960.69) was determined by the Soxhlet method using a solvent extraction system (Soxtec® Avanti 2050 Auto System, Foss Tecator AB, Sweden), and protein content (AOAC method 981.10) was determined with an automatic Kjeldahl nitrogen analyzer (Kjeltec® 2300Analyzer Unit, Foss Tecator AB, Sweden). Ash was determined using a muffle furnace according to AOAC method 920.153.
Cooking yield was determined for individual samples by calculating the weight before and after cooking as follows:
Meat batter viscosity was measured in triplicate with a rotational viscometer (MerlinVR, Rheosys, USA) at 20 rpm. A 2.0 mm gap was set between the 30 mm parallel plates that were then rotated at a constant shear rate (s-1) for 60 s prior to each reading to give apparent viscosity (cP). The temperature of each sample (25±1°C) during testing was also recorded.
Homogenates were prepared using 4 g meat samples and distilled water (16 mL). The pH of each homogenate was measured with a pH meter (Model S220, Mettler-Toledo, Switzerland). All measurements were performed in triplicate.
The color of uncooked and cooked meat samples were determined using a colorimeter (CR-10, Minolta, Tokyo, Japan; illuminate C, calibrated with a white plate, CIE L*=+97.83, CIE a*=–0.43, CIE b*=+1.98). Lightness (CIE L* value), redness (CIE a* value), and yellowness (CIE b* value) values were recorded.
TPA was performed in triplicate for each sample at room temperature using a texture analyzer (TA 1, Lloyd Co., USA). Samples (Ø 25×50 mm) were cut from the central region of each sausage. Prior to analysis, each sample was allowed to equilibrate to room temperature (25°C, 1 h). The TPA conditions were as follows: pre-test speed, 2.0 mm/s; post-test speed, 5.0 mm/s; maximum load, 2 kg; head speed, 2.0 mm/s; distance, 8.0 mm; and force, 5.0 g. TPA values were measured using a cylinder probe (Ø 25 mm) and were calculated from force and time plots. Values of hardness (kg), springiness, cohesiveness, gumminess (kg), and chewiness (kg) were determined for each sample.
A semi-trained group of ten panelists were engaged to evaluate the sensory qualities of each sausage sample in terms of color, flavor, tenderness, juiciness, and overall acceptability. Sausage samples were cooked to a core temperature of 75°C in a water bath (Model 10-101, Dae Han Co., Korea), cooled, cut into quarters (Ø 25×20 mm), and served randomly to the panelists. Panelists were instructed to cleanse their palates between samples with water. The color (1=extremely undesirable, 10=extremely desirable), flavor (1=extremely undesirable, 10=extremely desirable), tenderness (1=extremely tough, 10= extremely tender), juiciness (1=extremely dry, 10=extremely juicy), and overall acceptability (1=extremely undesirable, 10=extremely desirable) of the cooked sausage samples were evaluated using a ten-point descriptive scale.
All analyses were conducted at least three times under each set of experimental conditions, and the mean value±standard deviation of each parameter were determined. Variance analyses were performed on all the measured variables using the general linear model (GLM) procedure within the SAS statistical package (SAS, 2011). Duncan's multiple range test (p<0.05) was used to determine statistical differences among the treatment means. The procedure CORR of the SAS package was used to calculate correlations between viscosity and cooking yield.
Results and Discussion
The proximate compositions of sausages depending on the levels of added buckwheat powder are shown in (Table 1). While the moisture contents of the sausage samples were numerically increased with increasing levels of added buckwheat powder, sausages sample with 3% added buckwheat powder was the highest moisture content (p<0.05). Significant decreases in the protein (p<0.05) and fat (p<0.05) contents of the sausage samples were observed with increasing levels of added buckwheat powder, while the sausages sample with 3% added buckwheat powder presented the highest (p<0.05) ash content among the treated samples. These results are attributable to the high dietary fiber content of buckwheat powder that led to high moisture and ash, and low protein and fat contents in the sausage samples. Shin et al. (2017) reported that moisture contents of the emulsion-type chicken sausage samples were dramatically increased with increasing levels of added buckwheat powder. Grain powders such as buckwheat, black rice powder and red rice powder improved water holding capacity between meat proteins and water molecular (Park and Kim, 2016; Shin et al., 2017). In this study, the addition of buckwheat powder caused an increase in moisture content and resulted in a relative decrease in protein and fat content.
The pH and color in the uncooked and cooked sausage samples were dependent on the levels of added buckwheat powder (Table 2). The pH values of the sausages increased significantly with increasing levels of added buckwheat powder, irrespective of cooking, which is due to the pH of buckwheat powder (6.1). Shin et al. (2017) observed that the addition of buckwheat powder to chicken sausages presented a higher pH than the control, which was due to the high pH of the buckwheat powder. In both uncooked and cooked sausage samples, the addition of 3% buckwheat powder resulted in the highest L* and b* values. However, interestingly, the opposite trend was observed for a*; increasing the levels of added buckwheat powder led to significantly higher a* values in uncooked sausages and lower values in cooked samples. These color results are attributable to the color of buckwheat powder (L*: 75.0, a*: 1.3, and b*: 8.7). Similar results were reported by Choi and Chung (2007). Shin et al. (2017) reported that redness of chicken sausage showed a increasing tendency as the amount of added buckwheat powder increased.
The cooking yields of the sausage samples were affected by the added buckwheat powder (p<0.05; Fig. 1). According to Lee and Sohn (1994), this observation is caused by the water-binding ability of fiber. Many authors reported that the addition of dietary fiber s, such as rice bran, black rice, and isolated soy bean led to increases in cooking yield by enhancing the water-holding capacity and protein-binding ability of the meat (Choe et al., 2011; Steenblock et al., 2001).
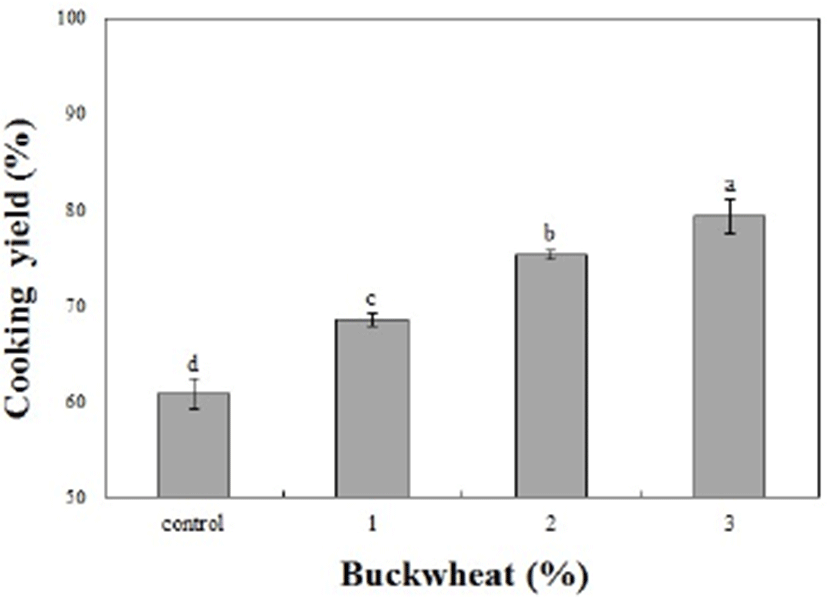
The viscosity of emulsified meat mince is closely related to its fat and water retaining capacity (Choe et al., 2011). As depicted in Fig. 2, higher levels of added buckwheat powder resulted in higher initial viscosities of the sausage samples (p<0.05). The sausage samples devoid of buckwheat powder exhibited the lowest initial (108.1 Pa.s) and final (58.9 Pa.s) viscosities. Among the treated samples, the highest final viscosity (p<0.05) was observed for the sausage sample containing 3% added buckwheat powder. This result is attributable to the fact that buckwheat-powder dietary fiber increases water retention in meat emulsions. Kim et al. (2009) reported that higher amounts of added wheat fiber and isolated soybean powder led to increases in viscosity and emulsion stability of emulsion-type sausages, resulting in decreases in cooking loss. Overall, improvements in the cooking yields and viscosities of emulsified meat products will help producing stable sausages.
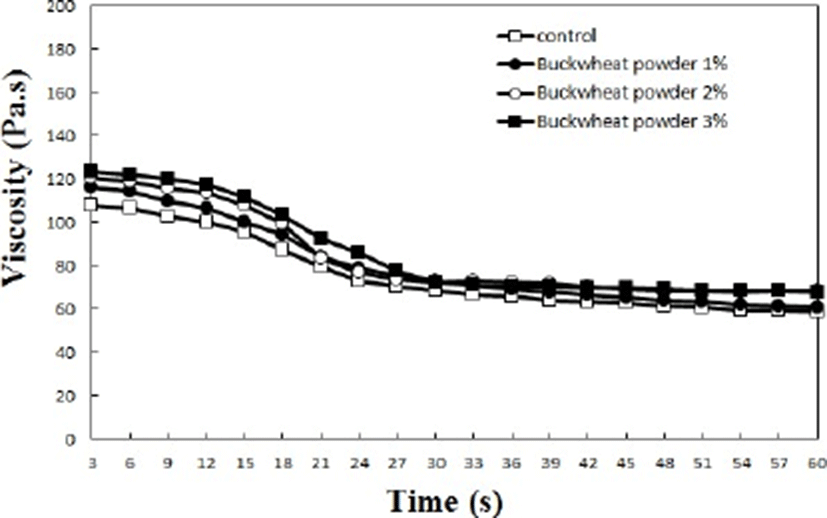
The relationship between the viscosity value and cooking yield of the meat batters prepared with various levels buckwheat powder is presented in Fig. 3. The viscosity value increased with increasing cooking yield in the batter (r2=0.7283) i.e. the correlation coefficient between the two measurements was high and positive. Kim et al. (2010) found that increases in the viscosity of a meat batter was primarily affected by water binding capacity and cooking yield. Also, Shand (2000) reported that increased viscosity led to increases in cooking yield and emulsion stability. This correlation demonstrates the relationship between emulsion viscosity and emulsion stability (Aktaş and Gençcelep, 2006).
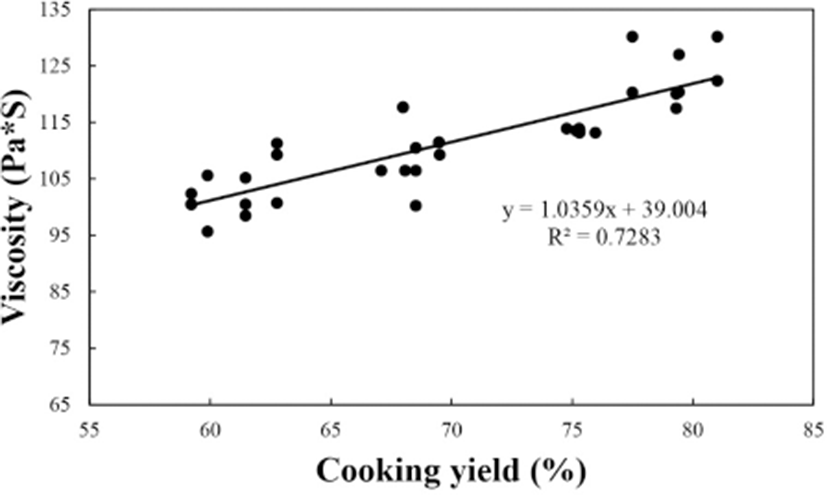
There were no significant differences in the texture profiles among the treated samples (Table 3). Previous studies found similar trendy result that the addition of dietary fiber, especially insoluble fiber, led to increases in the hardness of meat products (García et al., 2002; Steenblock et al., 2001). This result might be due to the formation of insoluble 3-dimensional structure in emulsion-type meat products, originated from insoluble fiber which has water-binding and swelling ability (Backers and Noli, 1997).
The panel detected sensory changes upon the addition of buckwheat powder to the emulsion-type sausages (Table 4). The additions of 2% or 3% buckwheat powder resulted in higher (p<0.05) color and flavor scores, but lower tenderness scores compared to the other samples. Panel detected the significant difference in tenderness between control and treated samples with buckwheat powder, exhibiting numerical difference in instrumental tenderness. The sausages with 3% buckwheat powder had the highest (p<0.05) juiciness and overall acceptability scores among the treated samples.
Conclusion
The results of this study demonstrate the application of buckwheat powder in emulsion-type sausages as exhibiting enhancement in physicochemical properties such as moisture retention, cooking yield, and viscosity and sensory traits. Especially, the addition of 3% buckwheat powder in emulsion-type sausage showed stable emulsion formation with a lower fat content and similar sensory property compared to no-added buckwheat counterpart. Therefore, the addition of buckwheat powder at level of up to 3% in emulsion-type should induce beneficial effect on quality property.