Introduction
Phosphates are one of the most widely used food additives in poultry and meat products because of their multifunctional properties (Petracci et al., 2013; Sebranek, 2009, Sebranek, 2015). Phosphates have multiple functional abilities such as improving water-holding capacity, increasing pH, chelating on metal ions, improving the freeze-thaw drip and cooking stability, and emulsifying stability. These abilities can improve yield, texture, and sensory properties, as well as extend the shelf life of meat products (Long et al., 2011; McKee and Alvarado, 2004; Sebranek, 2015; Thorainsdottir et al., 2004; Xiong, 2005; Young and Lyon, 1997). Despite these advantages, the use of phosphates in the meat industry is under severe scrutiny due to increasing interest in healthier foods and the poor consumer perception of phosphates owing to health risks associated with synthetic phosphate (Petracci et al., 2013). However, a direct substitute for phosphates has not been discovered so far (Sindelar, 2015), as it is difficult to cover their multifunctional properties.
Many previous studies have attempted to improve the functionalities of meat products by using various functional ingredients, such as SavorPhos (blends of citrus flour and sodium carbonate) (Casco et al., 2013), dried plums (Jarvis et al., 2012, Jarvis et al., 2015), functional carbohydrates, including guar gum, carrageenan, alginic acid, and chitosan (Park et al., 2008), isolated soy protein (Rhee, 1994), collagen products (Prestes et al., 2013; Schilling et al., 2003), whey protein isolates (Youssef and Barbut, 2011), and modified tapioca starch, wheat bran, and sodium citrate (Ruusunen et al., 2003). Recently, new approaches for identifying phosphate alternatives have been attempted to satisfy the market demand for meat products with natural and clean labels. Casco et al. (2013) reported that marinated chicken breasts with SavorPos (blends of citrus flour and sodium carbonate) had higher injection yield, lower cooking loss, and higher tenderness than those with a commercial phosphate blend. Egg shell calcium and oyster shell calcium are obtained from by-products generated during the processing of egg and oyster products, and widely used as calcium supplements because of their low cost and sustainability (Jang et al., 2010; Kim et al., 2015; Shin and Kim, 1997). These natural calcium powders are generally prepared by pulverization after washing with water and drying or calcining at about 800℃ to 1,000℃. Thus, the calcium powders from natural sources have high calcium contents and their main compound is calcium carbonate, which can be distinguished from synthetic calcium products such as calcium carbonate, calcium lactate, and calcium citrate (Kim et al., 2015; Lee and Park, 2002; Shin and Kim, 1997). In previous studies, the utilization of egg shell calcium and oyster calcium has been investigated in meat products to replace a synthetic phosphate. Park (2011b) attempted to evaluate the effects of oyster calcium, as a natural source, and whey protein concentrate on the functional properties of emulsion pork sausages. They found that pork sausages with 0.3% or 0.5% oyster calcium powder had significantly higher water holding capacity and hardness and chewiness values; they suggested that oyster calcium powder could be a suitable substitute for phosphate in the presence of 0.5% whey protein, providing pork sausages of quality similar to those with 0.3% phosphate. Another study compared the efficacy of 4 types of natural calcium (eggshell calcium, oyster shell calcium, marine algae calcium, and milk calcium) as substitutes for synthetic phosphates in meat products (Bae et al., 2017). It was suggested that eggshell calcium could be an ideal phosphate replacer for minimizing cooking loss, likely because of an increase in pH of the products, and oyster shell calcium appeared to be the second-best candidate for a phosphate substitute. In order to determine the optimal ratio of oyster shell and egg shell calcium, Cho et al. (2017) compared the effects of using oyster shell and egg shell calcium (alone and in combination) on the qualities of cooked pork products. They concluded that a combination of 0.2% oyster shell calcium and 0.3% egg shell calcium could replace synthetic phosphate in pork products, but textural properties of the final product were less desirable than the control with synthetic phosphate. Further research has been required if a combination of natural calcium mixtures and food ingredients could be even more effective for improving the quality properties of cooked ground pork products.
Therefore, we investigated the effects of different combinations of premixed natural calcium powders and various binding ingredients on the quality properties of cooked pork products, with the aim of developing high-quality phosphate-free meat products.
Materials and Methods
Raw pork ham (M. biceps femoris, M. semitendinosus, and M. semimembranosus) used in this study was purchased from a local meat processor (Pukyung Pig Farmers Livestock Cooperatives, Korea) at 24–48 h postmortem. After removing subcutaneous and intermuscular fat and visible connective tissues from the fresh ham, lean pork meat was cut in squares of 4–5 cm, vacuum-packaged in nylon/PE film bags, and stored in a freezer at -18℃ until processing. Pork back fat was prepared in the same manner. The frozen pork ham and back fat were stored at 2℃–3℃ for 24–36 h before processing. The thawed raw materials were ground in a chopper (TC-22 Elegant plus, Tre Spade, Italy) with an 8-mm plate and then again ground with a 3-mm plate. A phosphate blend (mixtures of sodium tripolyphosphate, tetrasodium pyrophosphate, and sodium hexametaphosphate) (Polymix-CS, SDBNI Co. Ltd., Korea) was used as the synthetic phosphate. The egg shell calcium (egg shell calcium 50, Essentron Co. Ltd., Korea) and oyster shell calcium (oyster shell calcium 40, JK Biochem Co. Ltd., Korea) were purchased from commercial suppliers. The egg shell calcium and oyster calcium powders tested in this study contained 39.6% and 51.6% calcium, respectively. As binding ingredients, egg white powder (Egg white, TOF Co. Ltd., Korea), whey protein concentrate (WPC, TOF Co. Ltd., Korea), concentrated soybean protein (CSP, TOF Co. Ltd., Korea), isolated soybean protein (ISP, Yujin Co. Ltd., Korea), carrageenan (carrageenan WG-5, MSC Co. Ltd.), and collagen powder (collagen powder, Ikjin Co. Ltd., Korea) were also prepared. The formulation of the pork meat products with the calcium powder mixtures and binding ingredients is presented in Table 1.
1) Control, 0.3% phosphate blend added; Treatment 1, 0.5% natural calcium mixtures (NCM; 0.2% oyster shell calcium powder and 0.3% egg shell calcium powder) and 0.25% egg white powder added; Treatment 2, 0.5% NCM and 0.25% whey protein concentrate added; Treatment 3, 0.5% NCM and 0.25% concentrated soybean protein added; Treatment 4, 0.5% NCM and 0.25% isolated soybean protein added; Treatment 5, 0.5% NCM and 0.25% carrageenan added; Treatment 6, 0.5% NCM and 0.25% collagen powder added.
The control was processed by mixing ground pork meat and back fat with 1.5% NaCl, 0.3% phosphate blend, and 15% ice water in a mixer (KitchenAid 5K5SS, Whirlpool Corp., USA) for 5 min (Fig. 1). For treatments, 1.5% NaCl and 15% ice water were incorporated into the ground pork meat and back fat; samples were then produced by combining 0.5% natural calcium mixtures (NCM, comprising 0.2% oyster shell calcium and 0.3% egg shell calcium powder) with 0.25% egg white powder (treatment 1), whey protein concentrate (treatment 2), concentrated soybean protein (treatment 3), isolated soybean protein (treatment 4), carrageenan (treatment 5), or collagen powder (treatment 6). The meat mixtures from each batch were stuffed into conical tubes (50 g each) using a stuffer (MOD.5/V Deluxe, Tre Spade, Italy). The stuffed tubes were centrifuged at 2,000×g for 10 min in a centrifuge (FELTA5, Hanil Scientific Inc., Korea) to remove air pockets. The tubes were then transferred into a 90℃ water bath (CB60L, Dong Won Scientific System, Korea) and cooked to an internal temperature of 75℃. Changes in temperature were monitored by inserting a K-type thermocouple attached to a digital thermometer (TES-1384, Ketech Scientific Instrument Co., Ltd., Taiwan) into the center of the samples. After cooking, the samples were immediately cooled on ice slurry for 20 min and placed at 2℃–3℃ overnight, prior to analyzing dependent variables. All experiments were performed in triplicate.
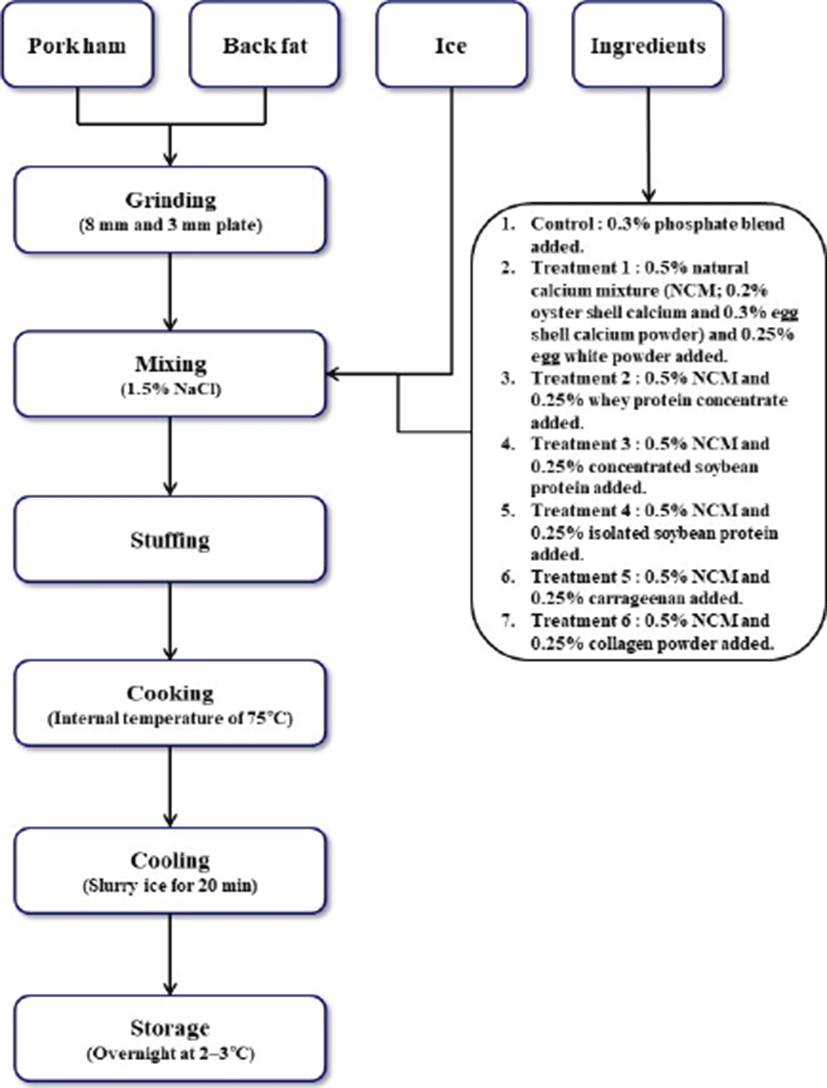
Five grams of the samples was weighed before and after cooking and homogenized with 20 mL of distilled water for 1 min in a homogenizer (DI 25 basic, IKA®-Werke GmbH & Co. KG, Germany). The pH was measured using a pH meter (Fisher Scientific Accumet AB150, Thermo Fisher Scientific, Inc., Singapore) equipped with a glass electrode.
Moisture content of the cooked products was measured using the drying method (AOAC, 2007). The samples were dried using a 105℃ dry oven (CB60L, Dongwon Scientific System, Korea) for 12 h, and the difference between the weight of each sample before and after drying was calculated.
As previously described, the weight of each sample in the conical tube was measured prior to cooking and then again after cooking and cooling to determine the cooking loss using the following equation:
Commission Internationale de l'Eclairage (CIE) measurements were used to determine lightness (CIE L*), redness (CIE a*), and yellowness (CIE b*). CIE L*a*b* values were measured on freshly cut surfaces of the cooked samples using a colorimeter (Chroma Meter CR-400, illuminant C; Konica Minolta Sensing Inc., Japan). Two slices from each cooked sample were cut in half (parallel to the longitudinal axis), and three CIE L*a*b* readings per slice were taken immediately after cutting. The colorimeter was standardized against a white calibration plate (CIE L*=+94.90, a*=-0.39, and b*=+3.88).
After cutting the samples to a width of 2.5 cm perpendicular to the longitudinal axis, their hardness, springiness, cohesiveness, gumminess, and chewiness were measured using a texture analyzer (TA-XT2i, Stable Micro System, UK) equipped with a 50-mm-diameter aluminum cylinder (Bourne, 1978). The cross-head speed for measurement was 5 mm/s.
All experiments were performed in triplicate. Statistical analysis of the results was performed using the generalized linear model (GLM) procedure of the SAS package program (SAS, 2012). When the analysis of variance showed a significant difference between the means of different samples, the significance of the differences was further analyzed by Duncan's multiple range test (p<0.05).
Results and Discussion
The pH of meat products can affect their water holding capacity. The incorporation of alkaline phosphates and their blends in meat products can increase the pH, causing unequal positive and negative charges in meat proteins and resulting in higher water holding capacity (Glorieux et al., 2017; Lamkey, 1998; Long et al., 2011). The pH values of ground pork products treated with calcium powder mixtures and various binding ingredients before and after cooking are presented in Table 2. Before cooking, the treatments had higher (p<0.05) pH than the control, although no differences in pH were observed (p>0.05) among the pork products treated with the different combinations of NCM and binding ingredients. The increase in pH of the treatments was probably due to the NCM added. Cho et al. (2017) had found that the incorporation of egg shell calcium and oyster shell calcium powders to ground pork products can increase the pH. They had also reported that egg shell calcium could more significantly increase the pH than oyster calcium. After cooking, the pH of the control was lower (p<0.05) than that of all the treatments. Among the treatments, treatment 6 had the highest pH, whereas treatments 2 and 3 had the lowest. The pH of treatments 1 and 4 were in between that of the others. According to Prestes et al. (2013), the pH of collagen fiber powder and collagen fiber were 7.78 and 8.05, respectively. Therefore, the high pH of treatment 6 could be attributed to the combined effect of calcium powders and collagen powder. Generally, higher pH in meat results in more water retention (Sebranek, 2009, Sebranek, 2015; Trout and Schmidt, 1983).
A–E Means within the same row with different superscript letters are significantly different (p<0.05).
1) Control, 0.3% phosphate blend added; Treatment 1, 0.5% natural calcium mixtures (NCM; 0.2% oyster shell calcium powder and 0.3% egg shell calcium powder) and 0.25% egg white powder added; Treatment 2, 0.5% NCM and 0.25% whey protein concentrate added; Treatment 3, 0.5% NCM and 0.25% concentrated soybean protein added; Treatment 4, 0.5% NCM and 0.25% isolated soybean protein added; Treatment 5, 0.5% NCM and 0.25% carrageenan added; Treatment 6, 0.5% NCM and 0.25% collagen powder added.
Based on the observed pH of the cooked products, it is expected that water retention ability would vary in the different formulations. However, all treatments had similar (p>0.05) moisture contents, compared to the control (Table 2). It has been shown that phosphates and salt in meat products increase the meat protein solubility and ionic strength, thereby contributing to water retention ability (Petracci et al., 2013; Sebranek, 2015; Trout and Schmidt, 1983). Therefore, this result indicated that the absence of synthetic phosphate had no detrimental effect on moisture content, which might have been due to the variation in pH caused by the addition of natural calcium powder and binding ingredients.
The cooking loss of ground pork products treated with calcium powder mixtures and various binding ingredients are shown in Table 2. Interestingly, the cooking loss ranged from 1.15%–2.21% in products with calcium powder mixtures and binding ingredients, which was lower (p<0.05) than that observed in the control (2.79%). In particular, treatments 2, 3, and 6 had the lowest (p<0.05) cooking loss, indicating that calcium powder mixtures coupled with whey protein concentrate, concentrated soybean protein, or collagen powder could be potential phosphate replacers for industrial applications. Bae et al. (2017), after testing eggshell calcium, oyster shell calcium, marine algae calcium, and milk calcium in ground pork products, suggested that eggshell calcium could be an ideal phosphate replacer for minimizing cooking loss. Park et al. (2008) tested guar gum, ĸ-carrageenan, alginic acid, and chitosan to identify a suitable substitute for inorganic polyphosphate in the pork model system. They reported that pork samples treated with guar gum and ĸ-carrageenan showed lower cooking loss than those treated with polyphosphate.
Table 3 shows the instrumental color values of ground pork products treated with calcium powder mixtures and various binding ingredients. We found no differences (p>0.05) in CIE L* values between the control and the treatments. In addition, all treatments with NCM and binding ingredients had similar (p>0.05) CIE L* values. CIE a* values of treatments 1, 2, 5, and 6 were not different (p>0.05) from the control, but those of treatments 3 and 4 were lower (p<0.05) than that of the control. Generally, cooked meat products with higher pH are darker and redder in color (Barbut, 2009; Casco et al., 2013; Trout, 1989). However, treatment with 0.5% NCM and 0.25% binding ingredients had no effect on the CIE L* value in this study. The lighter color of the binding ingredients likely contributed to lighter products by reducing the effect of pH (Hughes et al., 1998; Youssef and Barbut, 2011). The low CIE a* values in treatments 3 and 4 could be attributed to the inherent color of the soybean products used in this study. This result is consistent with that of Park (2011a), who reported that redness decreased with the addition of concentrated soybean protein in cooked pork patties. We found no differences (p>0.05) in CIE b* values between the control and treatments. These findings are consistent with the results of Cho et al. (2017), who demonstrated that cooked pork products treated with a combination of oyster shell calcium and egg shell calcium powder had similar CIE b* values.
A,B Means within the same row with different superscript letters are significantly different (p<0.05).
1) Control, 0.3% phosphate blend added; Treatment 1, 0.5% natural calcium mixtures (NCM; 0.2% oyster shell calcium powder and 0.3% egg shell calcium powder) and 0.25% egg white powder added; Treatment 2, 0.5% NCM and 0.25% whey protein concentrate added; Treatment 3, 0.5% NCM and 0.25% concentrated soybean protein added; Treatment 4, 0.5% NCM and 0.25% isolated soybean protein added; Treatment 5, 0.5% NCM and 0.25% carrageenan added; Treatment 6, 0.5% NCM and 0.25% collagen powder added.
Table 4 shows the textural properties of ground pork products treated with calcium powder mixtures and various binding ingredients. Among the samples tested in this study, the control had hardness similar (p>0.05) to treatments 2 and 6. Choi et al. (2014) obtained similar results by adding 0.5% oyster calcium and 0.5% whey protein to restructured pork ham, and Meullenet et al. (1994) reported similar results for chicken frankfurter treated with collagen fibers. The hardness of treatments 1, 3, 4, and 5 was lower (p<0.05) than that of the control. However, springiness and cohesiveness were not different between the control and treatments (Table 4). Cho et al. (2017) reported that springiness and cohesiveness of pork products treated with 0.5% oyster shell calcium or a combination of 0.3% oyster shell calcium and 0.2% eggshell calcium powder did not differ significantly from those of the control treated with synthetic phosphate blend, which is consistent with our findings. The control had higher (p<0.05) gumminess than all the treatments except treatments 2 and 6, which had a similar (p>0.05) gumminess as the control. Chewiness was higher (p<0.05) in the control than in treatments 1, 2, 3, 4, and 5, but no differences (p>0.05) in chewiness were found between the control and treatment 6. Previous studies have reported higher gumminess and chewiness in meat products with phosphate. Bae et al. (2017) reported that hardness, gumminess, and chewiness were significantly higher in the control samples with synthetic phosphate than in samples treated with natural calcium powders. Cho et al. (2017) found that gumminess and chewiness were the highest in pork products containing a phosphate blend and that a combination of 0.2% oyster shell and 0.3% egg shell calcium improved gumminess and chewiness, which may complement the textural properties of pork products. However, in this study, the combined use of calcium powders and whey protein concentrate or collagen powder improved the textural properties of pork products (Table 4). Lee et al. (2011) had reported that the addition of oyster shell powder (0.15%, 0.3%, and 0.5%) and 0.5% whey protein to emulsion sausages significantly increased their hardness, springiness, and chewiness, compared with sausages with or without 0.3% sodium tripolyphosphate, which was partially consistent with the results of our study.
A–C Means within the same row with different superscript letters are significantly different (p<0.05).
1) Control, 0.3% phosphate blend added; Treatment 1, 0.5% natural calcium mixtures (NCM; 0.2% oyster shell calcium powder and 0.3% egg shell calcium powder) and 0.25% egg white powder added; Treatment 2, 0.5% NCM and 0.25% whey protein concentrate added; Treatment 3, 0.5% NCM and 0.25% concentrated soybean protein added; Treatment 4, 0.5% NCM and 0.25% isolated soybean protein added; Treatment 5, 0.5% NCM and 0.25% carrageenan added; Treatment 6, 0.5% NCM and 0.25% collagen powder added.
Conclusions
In this study, NCM and various non-meat ingredients were added to ground pork products to evaluate potential substitutes for synthetic phosphate. NCM and the tested ingredients increased the water holding capacity (lowering the cooking loss), possibly by increasing the pH of the pork products. In addition, no negative effects were observed regarding the moisture content and instrumental color of the final products, although treatments 3 and 4, which contained soybean protein, had lower CIE a* values than the control. Compared to the control (containing synthetic phosphate blend), treatments 2 and 6 had a notable effect on the textural properties of the ground pork products. Therefore, the combination of NCM and whey protein concentrate or collagen powder could be ideal for producing phosphate-free meat products. Further research is required for determining the optimal proportions of the combinations for industrial application.