Introduction
Various kinds of diseases are increasing due to westernization of food intake habits and lifestyle of modern people (Carrera-Bastos, 2011). As aging accelerates, interest in health has increased. Additionally, as living standards increase, food is no longer being eaten for hunger, but to improve health and lifestyle (Nordstrom et al., 2013; Schneider and Davis, 2010). Therefore, products with improved functionality in the dairy market are being developed and demanded.
On the other hand, the spread of westernized diet, high fat and protein but low vegetables and fruits, is associated with incidence of inflammatory bowel diseases (IBD) (Hou et al., 2011). IBD is divided into two major types, including ulcerative colitis and Crohn’s disease and have been characterized by chronic and recurring intestinal inflammation. (Lichtenstein and McGovern, 2016). The most symptoms of IBD are known to diarrhea, fatigue (Singh et al., 2011), palindrimic inflammation and injury of epithelial tissue (de Moreno de LeBlanc et al., 2015), which leads to reduce of life quality (Simren et al., 2002).
Yogurt is one of major dairy products produced by the fermentation of milk. Its lactic acid bacteria (LAB) ferment lactose and produce lactic acid, and it affect milk protein, and give yogurt textural and its own properties (Kim and Oh, 2013). The desirable effects of yogurt are nutritional benefits, prevention of diarrheal disease, immune regulation, prevention of osteoporosis, cholesterol reduction, and cancer prevention (Kim and Oh, 2013). Especially, according to many reports (Baroja et al., 2007; Campieri et al., 2000; Dotan and Rachmilewitz, 2005; Matsumoto et al., 2001), yogurt contained probiotics is effective to prevent IBD and to modulate immune system in IBD patients.
There are various types of yogurt products, such as plain or flavored yogurt, strained yogurt, concentrated yogurt, and so on (Chandan and O’Rell, 2006). However, all of these are fermented in approximately at 37°C.
Fermentation at low temperature has been studied in many fermented foods, for instance, kimchi and wine (Hong et al., 2016; Redon et al., 2011; Torija et al., 2003; Tronchoni et al., 2012). It was reported that the degree of lipid unsaturation and lipid composition were affected by fermentation temperature and medium-chain fatty acids were enlarged by low fermentation temperature in manufacturing of wine (Torija et al., 2003; Tronchoni et al., 2012). In addition, volatile composition and pungent odor were different by low temperature fermentation in Kimchi (Hong et al., 2016).
In yogurt fermentation, Gjorgievski et al. (2014) reported that the antioxidant activity of milk increased after fermentation with various probiotics. Nonetheless, it is hard to find out the associated reports regarding yogurt fermentation in low temperature. Therefore, this study was conducted to evaluate the effect of fermentation at low temperature on the antioxidant activity of yogurt, and on its anti-inflammatory effect using a mouse model.
Materials and Methods
Market milk and skim milk powder were purchased from Maeil Dairies Co., Ltd. (Seoul, Korea) and Seoul Dairy Coop. (Seoul, Korea). Commercial yogurt starter culture, YAB 472 EC, containing Lactobacillus acidophilus, Bifidobacterium lactis, Streptococcus thermophilus, and Lactobacillus delbrueckii subsp. bulgaricus was purchased from Sacco system Co. (Cadorago, Italy).
Homogenized and pasteurized commercial milk was mixed with 2.7% (w/v) skim milk powder. Next, it was pasteurized at 80°C for 15 min and cooled down to 22°C and 37°C for low temperature-fermented yogurt (YFL) and general temperature-fermented yogurt (YFG), respectively. Commercial yogurt starter YAB 472 EC (0.04%, w/w) was inoculated and fermented. Each yogurt sample was fermented until pH 4.6 and later stored at 4°C.
pH was measured with pH meter (PP-15, Sartorius AG, Germany), and titratable acidity (TA) assayed as % lactic acid by titration with 0.1 N NaOH (F=1.022) and phenolphthalein as an indicator in each sample (10 g) with 10 mL DW. Next, the amount of 0.1 N NaOH added was used in the following equation:
One gram of yogurt was diluted in 95% ethanol. After mixing thoroughly, the sample was centrifuged at 440×g for 20 min at 4°C, and the supernatant fraction was separated for further assays. We prepared 100 μM of DPPH solution (Sigma Chemical Co., St. Louis, MO, USA) in methanol and added 100 μL of samples (1:1 ratio) in a 96-well plate. After incubation for 30 min, DPPH radical scavenging activity of each yogurt was assessed spectrophotometrically by the DPPH cation de-colorization assay at 517 nm (Blois MS, 1958).
One gram of yogurt was diluted in 95% ethanol. After mixing thoroughly, the sample was centrifuged at 440×g for 20 min at 4°C, and the supernatant fraction was separated for further assays. ABTS+ working solution was prepared with 5 mL of 7 mM ABTS solution, and 88 μL of 140 mM potassium persulfate, and incubated in the dark for 12–16 h. ABTS+ working solution was diluted in 95% ethanol in order to reach an OD of 0.70±0.02 at 734 nm, and was mixed with samples on a 1:1 ratio in a 96-well plate. After incubation for 30 min, ABTS radical scavenging activity of each yogurt was assessed spectrophotometrically by ABTS+ cation de-colorization assay at 734 nm (Re et al., 1999).
One gram of yogurt was diluted in distilled water. After mixing thoroughly, the sample was centrifuged at 440×g for 20 min at 4°C, and the supernatant fraction was separated for further assays. The FRAP assay was conducted according to the method of Benzie and Strain (1996) with some modifications. The fresh working solution was prepared by mixing 50 mL acetate buffer, 5 mL TPTZ (Sigma Chemical Co., St. Louis, MO, USA) solution, and 5 mL FeCl3 (Sigma Chemical Co., St. Louis, MO, USA) solution, and warmed at 37°C before assay. Readings of the colored product were then taken at 593 nm. The results are expressed in μM TE (trolox equivalent)/g fresh mass.
The experimental procedure of animal study was approved by Institutional Animal Care and Use Committee at Kangwon National University (KW-170615-1).
Balb/c mice (male, 6 weeks old) were purchased from Dae-Han bio link (Chungbuk, Korea) and kept in an air-conditioned room at 20±2°C, under a 12-hour light dark cycle. The mice were given ad libitum access to tap water and a standard diet. Acute colitis was induced in mice by adding 2.5% (w/v) dextran sodium sulfate (DSS; MP Bio-medicals, LLC, France) to their drinking water for 7 days.
Animals were randomly separated into 5 groups (n=6): Group 1 (normal control, NC) mice were treated with just drinking water during the colitis-inducing period, and treated with 300 μL distilled water during oral sample administration period; group 2 (negative control, DC); group 3 (positive control, SC) were treated with 2.5% DSS water and 25 mg/kg sulfasalazine; group 4 (DYG) mice were treated with 2.5% DSS water and 300 μL of water-diluted yogurt (1:1 ratio) fermented at 37°C; and group 5 (DYL) mice were treated with 2.5% DSS water and 300 μL of water-diluted yogurt (1:1 ratio) fermented at 22°C (Table 1).
Treatment | Group (μL) | ||||
---|---|---|---|---|---|
NC | DC | SC | DYG | DYL | |
DSS (2.5%) | − | + | + | + | + |
DW | 300 | 300 | − | − | − |
Sulfasalazine (250 mg/L) | − | − | 300 | − | − |
Yogurt sample | − | − | − | 300 | 300 |
Fermentation temperature (°C) | − | − | − | 37 | 22 |
Distal colon tissue (about 1 cm) was fixed in 10% formalin and embedded in paraffin, and was sectioned at 4 μm and stained with hematoxylin and eosin (H&E). Histological analysis was performed using light microscopy (Olympus CKX41, Olympus Co. Ltd., Tokyo, Japan).
To measure contents of cytokine IL-6 (interleukin 6), ELISA kit (R&D systems Inc., Minneapolis, USA) was used. Briefly, 50 μL of samples was added into the wells of the plate with diluent buffer, mixed well for 1 min and incubated at room temperature for 2 h. Next, 100 μL of mouse IL-6 conjugate was added into each well and incubated for 2 h. After discarding the supernatant in the wells, 100 μL of substrate solution was added and incubated at room temperature in the dark. After adding 100 μL of stop solution, the plate was read at 450 nm wavelength.
Contents of cytokine IL-4 and IL-10 in colon tissue were measured using ELISA kits (Koma biotech, Korea). Briefly, 100 μL of samples were added to each well and after 2 h, 100 μL of the diluted detection antibody (0.15 μg/mL) was added and incubated at room temperature for 2 h. The diluted color development enzyme was added and incubated for 30 min. Color development solution and stop solution were added to each well and the plate was read at 450 nm wavelength.
Contents of MPO in colon tissue were measured using an MPO kit (Origene, USA). Briefly, 100 μL of sample was added to each well, and after incubation for 2 h at 37°C, 100 μL of bio-anti mouse MPO was added and incubated at 37°C for 1 h. Afterwards, ABS working solution and TMB color developing agent were added and incubated at 37°C for 30 min in the dark. Color development solution and stop solution were added to each well and the plate was read at 450 nm wavelength.
Results and Discussion
Changes in pH and TA of yogurt during fermentation was indicated in Fig. 1. As shown, the pH of LFY gradually decreased, and it took approximately 27 h to reach a value of 4.6. Nontheless, that of GFY decreased rapidly, and it took approximately 12 h to reach pH 4.6. The time required for GFY to reach pH 4.6 was approximately half to that of LFY.
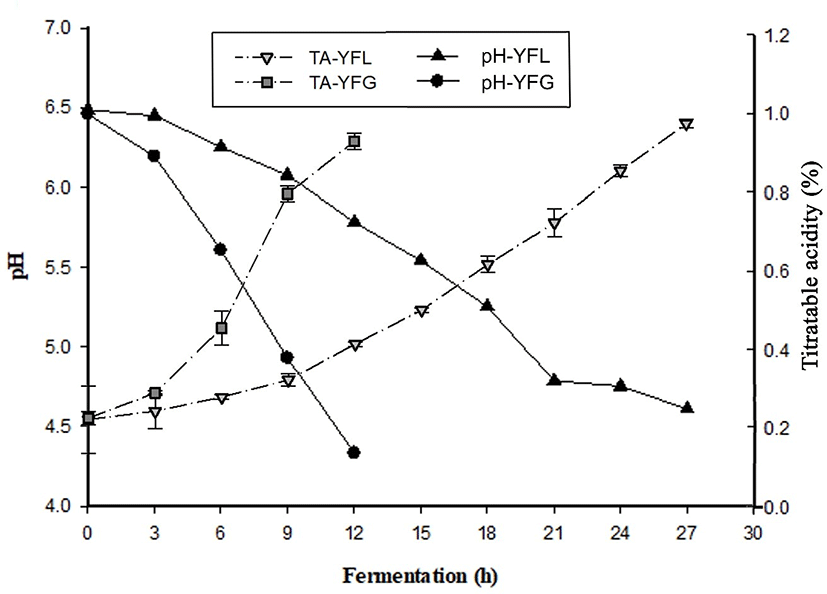
As shown in Fig 1b, GFY showed fast TA increase during fermentation, especially during hours 6 to 9 of the process. At the end of the fermentation, TA was 0.96±0.01. On the other hand, TA in LFY increased gradually. This aspect was similar to the changes in pH. It is considered that probiotics are not active in low temperature fermentation.
Fig. 2A shows antioxidant activity of yogurt fermented at low and general temperatures. Regarding the DPPH radical scavenging activity evaluation, in YFG it was 17.9±1.33, 28.36±1.22, 38.9 5± 1.13, 49.64±2.10, and 59.35±1.59% at 5, 10, 15, 20, and 25 mg/mL of yogurt sample concentration, respectively, with significant differences (p<0.05). The result of DPPH in YFL was 33.21±6.16, 53.00±3.32, 59.61±2.45, 64.82±2.22, and 74.23±3.23% at 5, 10, 15, 20, and 25 mg/mL of yogurt sample concentration, respectively, with significant differences (p<0.05). The result of ABTS+ radical scavenging activity in YFG (Fig. 2B) was 31.08±3.69, 55.08±4.94, and 79.23±4.32% at 10, 20, and 30 mg/mL of yogurt sample concentration, respectively, with significant differences (p<0.05). The results of ABTS+ in YFL was 50.72±1.88, 85.28±1.46, and 86.77±0.29% at 10, 20 and 30 mg/mL of yogurt sample concentration, respectively, with significant differences (p<0.05). As shown in Fig. 2C, the FRAP activity in YFG was 5.54±0.15, 9.61±0.31, 13.34±0.31, 16.55±0.37, and 18.97±0.24 TE μM/mL at 5, 10, 15, 20, and 25 mg/mL of yogurt sample concentration, respectively, with significant differences (p<0.05). In YFL, FRAP activity was 5.80±0.53, 10.40±0.15, 15.27±0.26, 20.17±0.39, and 24.02±1.04 TE μM/mL at 5, 10, 15, 20, and 25 mg/mL of yogurt sample concentration, respectively, with significant differences (p<0.05). In addition, YFL indicated significantly higher antioxidant activity compared to YFG in most of samples.
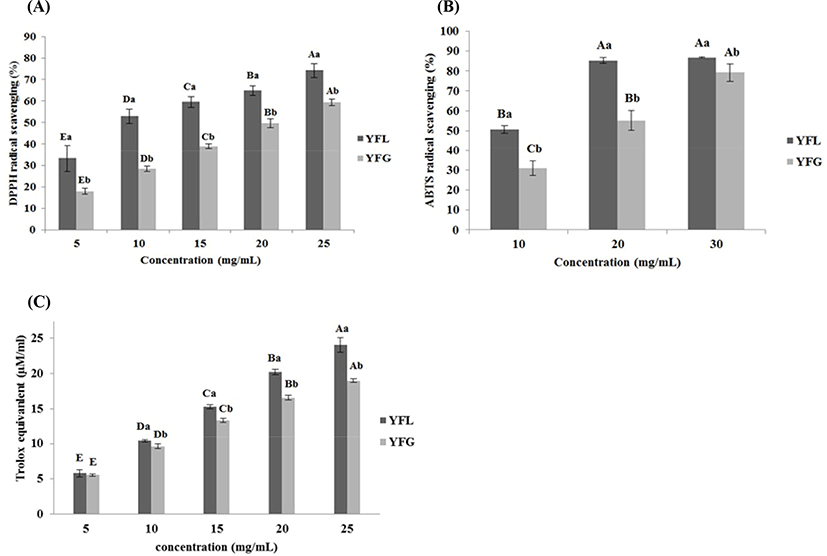
The difference of radical scavenging activity between DPPH and ABTS+ was caused by its solubility and diffusivity in the substrate. In the case of DPPH, it has “weakness” when the radical scavenger is hydrophilic since DPPH is soluble in organic solvents. However, ABTS+ dissolves well in both solvents, water and organic solvents. Thus, the radical scavenging effect derived from hydrophilic and lipophilic antioxidants can be measured by it (Tang et al., 2010). Hur et al. (2014) described that antioxidants are stable in low pH environments. Additionally, hydrolysis and release of cell wall components by fermentation leads to the phenolic compounds release in food, and this affects antioxidant capacity. According to Virtanen et al. (2007), the antioxidant activity of yogurt is derived from the hydrolysis of milk components by LAB; in particular, the hydrolysis products weighing 4–20 kDa showed remarkably high antioxidant activity in the antioxidant effect assay. In addition, Sah et al. (2014) reported that as the degree of hydrolysis of milk proteins increased, the antioxidant effect, such as DPPH, ABTS, and FRAP radical scavenging activity, also increased. Taking this into account, the high antioxidant activity of low-temperature fermented yogurt is thought to be due to the higher degree of hydrolysis of milk proteins than that in general fermented yogurt.
After sacrificing all mice in groups NC, DC, SC, DYG, and DYL, the colonic tissue length, from cecum to rectum, was dissected and measured. The results of colon length and organ weight on Balb/c mice with colitis induced with DSS are showed in Fig. 3 and Table 2, respectively. NC group showed the highest colon length with 8.53±0.40 cm. The colon length in DC, negative group, was 7.24±0.51 cm, which is the shortest significantly among treatments (p<0.05), and the colon length was 7.87±0.21 cm in SC, 7.89±0.32 cm in DYG, and 7.90±0.45 cm in DYL. Colon length, shortened by DSS treatment, was significantly longer with the administration of yogurt, which means that feeding of yogurt was significantly beneficial to the improvement of colitis. However, no significant difference was observed between DYL and DYG (p>0.05). Similarly, Jing et al. (2017) detected significant abbreviation of colon and decrease of body weight in the DSS-induced colitis model for 6 days. Similarly, feeding of Lactobacillus lactis S-SU2 improved the abbreviation of colon length in DSS-induced IBD mice (Kawahara et al., 2015).
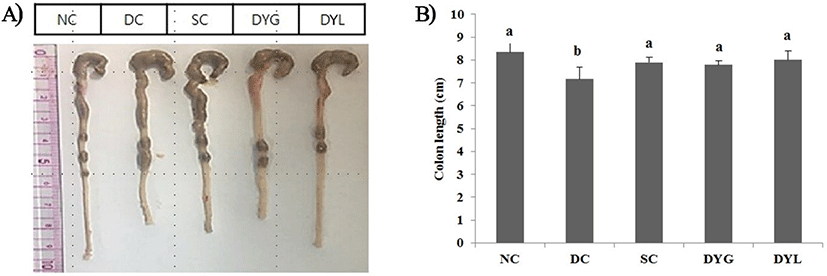
1 NC, normal control; DC, DSS, negative control; SC, DSS group with sulfasalazine (250 mg/L), positive control; DYG, DSS group with yogurt fermented at general temperature (37°C); DYL, DSS group with yogurt fermented at low temperature (22°C); DSS, dextran sodium sulfate. Each value represents mean±SD (n=6).
On the other side, significant difference was not found in weight of liver, testis, and kidney (p>0.05). However, the weight of the spleen was 0.07±0.00 g in NC, which weighs the least among the treatments, and the spleen weight in DC was 0.09±0.02 g, which weighs the most (p<0.05). The weight of the spleen significantly decreased in the yogurt administrated groups (p<0.05). Difference on weight of the spleen could lead us to guess the degree of inflammation, since the spleen might be bigger due to the immune response or inflammatory reaction (Nunes et al., 2018). According to another study, the spleen can be hypertrophied by the DSS response (Herias et al., 2005).
Recent studies indicate that DSS-induced intestinal injury and inflammation are alleviated by microbial metabolites, including proteins and polyphosphates (Segawa et al., 2011; Yan et al., 2011). Also, similar to this study, Yoda et al. (2014) reported that intake of Lactobacillus GG-fermented milk can significantly improve colonic inflammation, injury, and colon abbreviation in DSS-induced colitic mice. Furthermore, Geier et al. (2007) reported that yogurt with probiotics, such as Lactobacillus rhamnosus GG, Lactobacillus fermentum BR11, and Streptococcus thermophilus TH-4 were effective to reduce symptoms from colitis.
Fig. 4 shows histological observation of the colonic cells. Microscopically, histological damage in colonic mucosa was not detected in NC. However, inflammatory changes in colonic architecture was founded in DC. In contrast, histological analysis of the colons from yogurt-administrated mice, DYG and DYL, denoted greatly reduced cell infiltration, mucosal injury, and edema. These results were associated with Yoda et al. (2014), who reported that abnormalities by DSS treatment in C57BL/6 mice were significantly improved by LGG-fermented milk feeding. Similarly, according to Zaylaa et al. (2018), feeding of probiotics led to decrease of histological score and recovery of damage by DSS in microscopic observation. The mechanism of inflammation induction by DSS is unclear, however, the acute inflammatory response is more likely occur from the nonspecific disruption or injury of the basolateral intercellular space of the colonic epithelium (Koboziev et al., 2011), and it is considered that yogurt consumption is helpful to alleviate these cell injury.
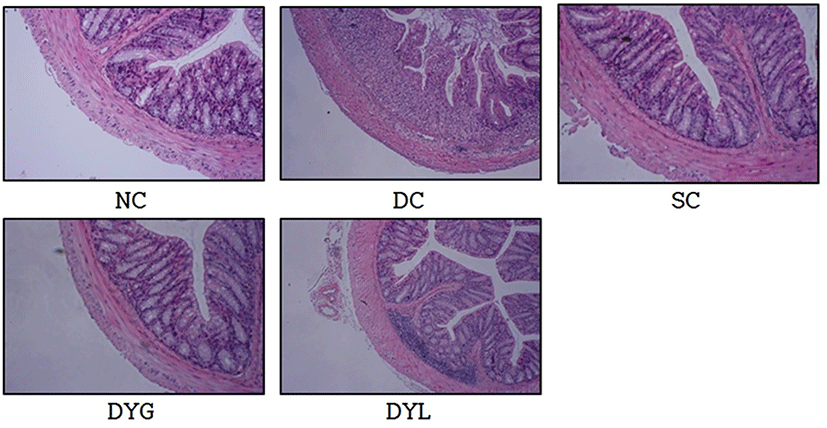
The result of IL-6 content in serum of Balb/c mice with DDS-induced colitis is shown in Fig. 5. IL-6 is an inflammatory cytokine that increases in serum, plasma, or tissue by inflammation. IL-6 content of serum in NC was the lowest with 1.40±0.49 pg/mL, and the content in DC was the highest with 5.09±1.42 pg/mL (p<0.05). Additionally, IL-6 content in other groups was 1.71±0.68 pg/mL in SC, 2.57±0.49 pg/mL in DYG, and 1.96±1.08 pg/mL in DYL. Sample groups treated with sulfasalazine and yogurt (SC, DYG, and DYL) showed decreasing IL-6 levels comparable to NC, which means yogurt had an effect on IL-6. Nevertheless, there was no significant difference between DYG and DYL, although IL-6 level was lower in DYL compared to that in DYG.
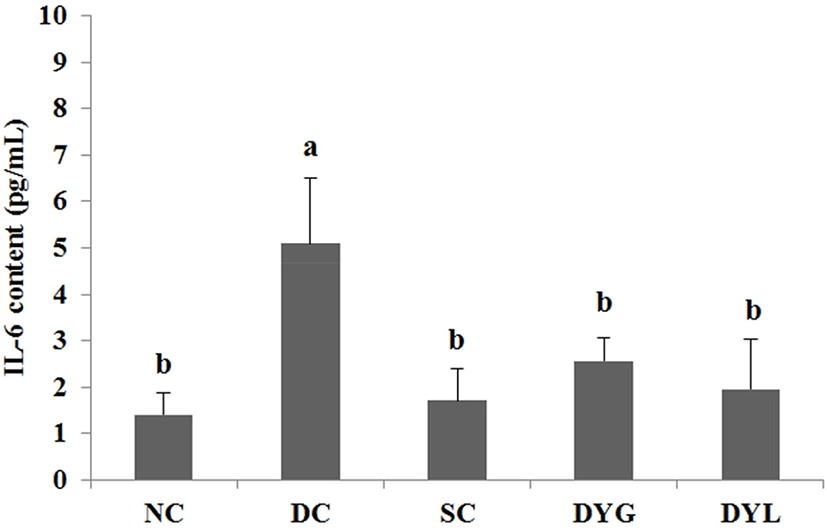
Yang and Sheu (2012) investigated immune response after ingestion of yogurt in Helicobacter pylori-infected children. In their study, after intake of yogurt, the level of IL-6 in serum significantly decreased. Additionally, the levels of other serum cytokines, such as TNF-α and IL-10, decreased, and serum IgA increased as immune protection. Miyauchi et al. (2013) reported that Bifidobacterium longum subsp. infantis suppresses DSS-induced colitis, in particular, IL-6 level was decreased in DSS-treated mice.
The result of cytokines in colon tissue of DSS-induced colitic Balb/c mice is shown in Fig. 6 and Fig. 7. The IL-4 levels of colonic tissue were 61.09±12.81 pg/mL in NC which is the highest (p<0.05), 16.44±10.12 pg/mL in DC, which is the lowest (p<0.05), 44.85±7.13 pg/mL in SC, 27.45±7.07 pg/mL in DYG, and 46.30±11.51 pg/mL in DYL. The IL-10 levels of colonic tissue were 321.44±100.73 pg/mL in NC, which is the highest (p<0.05), 71.03±33.15 in DC which is the lowest (p<0.05), 179.09±76.55 pg/mL in SC, 209.75±67.38 pg/mL in DYG, and 265.21±62.66 pg/mL in DYL. Although there was no significant difference between DYG and DYL, the levels of IL-4 and IL-10 in DYL were higher compared to those in DYG.
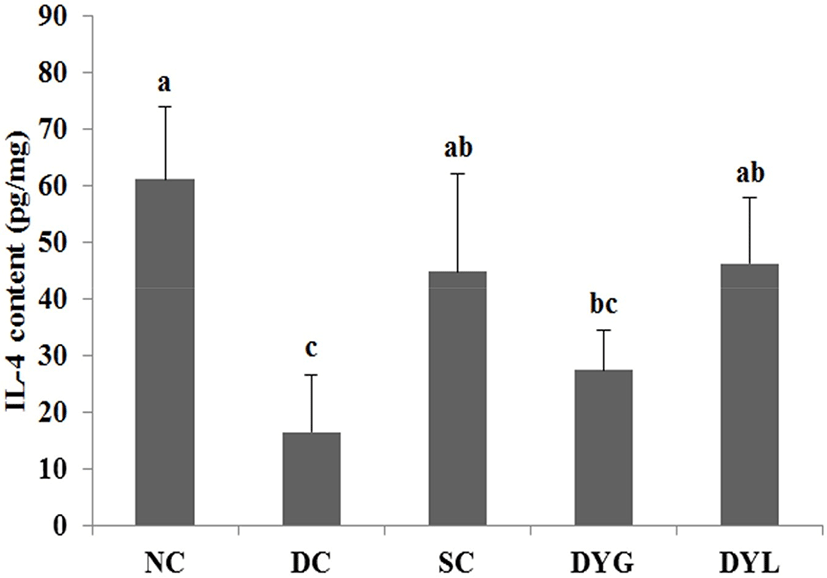
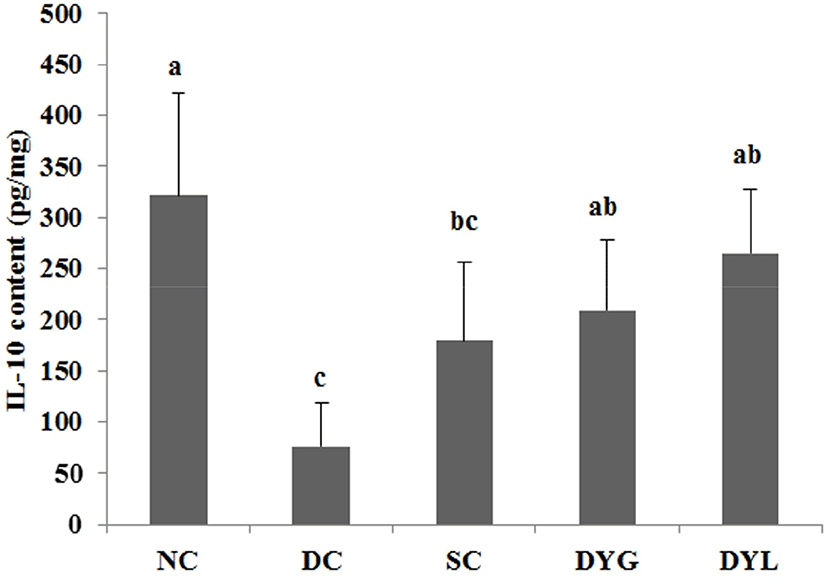
IL-4 and IL-10 are mainly produced by T-helper cells type 1 (Th1) (Adolfsson et al., 2004), and IL-10 is also a product of T-helper cells type 2 (Th2), cytokine synthesis inhibitor in Th1 cells and is hence termed a inhibitor of cytokine synthesis (Howard and O’Garra, 1992; Lalani et al., 1997; Opal et al., 1998). IL-10 deficiency is frequently observed in inflammatory bowel disease (de Moreno de Leblanc et al., 2011). Sheikhi et al. (2016) reported that probiotics, such as Bifidobacterum lactis BB-12 and Lactobacillus acidophilus LA-5 modulate cytokine secretion in patient with ulcerative colitis. In their research, these probiotics significantly enhanced secretion of IL-10 and the secretion more increased after 72 h. Thus, these results suggest that administration of yogurt contributed to improvement DSS-induced colitis in mice.
The result of MPO levels in colon tissue of Balb/c mice with DSS-induced colitis is shown in Fig. 8. The MPO level of colonic tissue in NC was the lowest with 3.32±2.31 ng/mg (p<0.05), which means that inflammation in the colon was very low. The MPO levels of colonic tissue were 51.45±9.12 ng/mg in DC which is the lowest (p<0.05), and 25.85±12.24 ng/mg in SC, 26.84±10.37 ng/mg in DYG, and 26.20±11.62 ng/mg in DYL. There was no significant difference between the general temperature-fermented yogurt group and the low temperature-fermented yogurt group in MPO level (p<0.05).
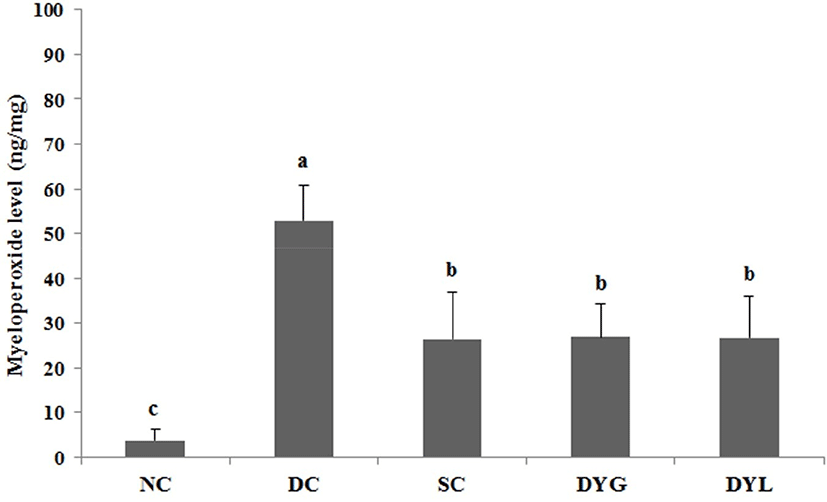
MPO is a peroxidase that is mostly expressed in neutrophil granulocytes (white blood cells) and catalyzes the production of hypohalous acids that aid the cells carry out their antimicrobial activity (Klebanoff, 2005). In the present study, DYG and DYL decreased the level of MPO as much as SC. Wasilewska et al. (2019) reported that overexpressed MPO causes normal tissue damage and inflammation, and decrease of MPO activity and extention of colon length were observed in yogurt starter culture fed DSS-induced colitis mice model. Similarly, Miyauchi et al. (2009), the level of MPO on distal colon was significantly decreased by administration of Lactobacillus rhamnosus OLL2838 in DSS-induced colitis mice.
Probiotics has attracted public attention as a new treatment strategy for IBD and have been used successfully in IBD patients (Orel and Kamhi Trop, 2014; Saez-Lara et al., 2015). Therefore, in view of these facts, it suggests that consumption of yogurt can improve inflammation in colon regardless of fermentation temperature.
Conclusion
This study was conducted to investigate the effect of fermentation temperature in yogurt on increased functionalities, such as antioxidant activity. An animal experiment using mice was performed to assess the effect of yogurt fermentation at low temperature. The antioxidant activity of yogurt fermented at low temperature was higher than that of yogurt fermented at general temperature (p<0.05). In animal experiments, yogurt fermented at low temperature had effects on the physiological condition, the histological analysis, the cytokine levels, or the myeloperoxidase level of the evaluated samples, although there was no significant difference between general temperature and low temperature. In conclusion, yogurt fermented at low temperature has anti-inflammatory activity on the DSS-induced colitis mouse model, Although there was no significant difference between these yogurts in anti-inflammatory effect, yogurt fermented at low temperature is considered to be sufficient as a functional food, and is necessary to further study.