Introduction
Currently, there is an increasing trend in the consumption of processed meat products in Korea due to an increase in consumer income level and changes in dietary culture (Korea Meat Industries Association, 2018). According to a report by the Korea Rural Economic Institute (2015), over 40% of consumers believe that excessive use of additives negatively affects the nutrient value of processed meat products and consumer health, while 27.5% of consumers plan to reduce the purchase of processed meat products in the future in response to the perceived negative effects on health and safety concerns. Thus, consumers prefer processed meat products, including sausages (Cho, 2002), without artificial additives, and this trend in consumer preference depends on palatability, convenience, and guaranteed safety upon use of less food additives (Choe et al., 2008; Chung, 2014). Therefore, recent studies have been conducted to develop functional sausages using natural components with biological activity as additives in place of traditional food additives (Hyon et al., 2003).
The most well-known palm cactus (Opuntia ficus-indica var. saboten Makino), commonly referred to as Baeknyuncho, is a tropical plant in the family Cactaceae that is naturally found in the Jeju Island. The other known palm cactus naturally found in Korea is Chunnyuncho (Opuntia humifusa), which is a perennial plant that can survive at a temperature of −20°C (Yoon and Son, 2009). Wanggasi-Chunnyuncho (O. humifusa f. jeollaensis), in contrast to Baeknyuncho and Chunnyuncho, has a red color in the center of a yellow flower and large thorns of 3 cm in length (Kim, 2016). Otherwise, the characteristics of Wanggasi-Chunnyuncho, excluding the flower color and large thorns, are similar to those of Chunnyuncho. The fruit of Wanggasi-Chunnyuncho is of a similar color and composition with those of the Chunnyuncho fruit (Kim, 2006) consisting of high dietary fiber content and a viscous material comprised of arabinose, galactose, xylose, galacturonic acid, and rhamnose, with pectin fiber as the primary component (Kim et al., 2007; Park et al., 2010). The dietary fiber contributes to the binding capacity of processed meat products for moisture and fat, affects the cooking loss and emulsion stability (Cofrades et al., 2000). The red fruit pigment of Wanggasi-Chunnyuncho is created by betacyanin and brown betaxanthin, which are water-soluble pigments with stable pH values, and antioxidant activity, which increase their commercial value (Georgiev et al., 2010; Nemzer et al., 2011). Betalain, the red pigment in the Wanggasi-Chunnyuncho fruit (WCF), has been approved by the Food and Drug Administration (USA) for use as a food coloring agent in meat products, yogurts, cold drinks, and frozen desserts (Spears, 1988; Von Elbe et al., 1975). Research on the new Wanggasi-Chunnyuncho species as a food material is lacking, with only one published study that focused on its DNA sequence (Kim, 2006).
The viscous substance in cactus fruit contains dietary fiber and polysaccharide, consisting of approximately 30,000 monosaccharides (Paulsen and Lund, 2006). This viscous dietary fiber is stable when heated and contributes to the stability of the red pigment and maintenance of the physical properties under acidic conditions, and thus, has the potential to serve as an additive to promote functionality and quality of food in the food industry (Lee et al., 1998). The fruit is regarded as a high value crop due to the recognition of the properties of its natural red pigment and viscous dietary fiber, which has led to increased production output. However, the reputation of this fruit has been damaged by a lack of utilization method and reduced consumption. The viscosity of the dietary fiber of the fruit and the stability of its red pigment as an additive have not been rated highly, resulting in its limited use in the food processing industry (Choi, 2011).
Therefore, in this study, we produced a WCF powder sausage and evaluated its quality and sensory characteristics to determine the effects of WCF components on emulsified sausages and to assess the potential of this fruit to be used as a food additive.
Materials and Methods
To prepare sausages, pork back fat and a hind leg were purchased from Happy Pork (Asan, Korea). WCF, cultivated in Iksan, Jeollabuk-do, were purchased in October 2018. The fruits were washed and freeze-dried to be used in sausage production. The sausage was prepared by grinding the pork back fat and hind leg using a grinder (5KSM150, KitchenAid, St. Joseph, MI, USA) with a 7-mm plate. The pork hind leg, back fat, and ice were placed in a food mixer (SF-100, Samwoo Industry Co., Deagu, Korea) to create a batter. Subsequently, nitrite pickling salt, sugar, mixed spices, and the WGF were added to produce emulsified sausages (Table 1). The sausage emulsion was packaged in natural pork casing (28 mm, HS Newtech, Deagu, Korea) using a filling machine (5KSM150, USA). The stuffed sausage was heated in a water bath at 80°C for 30 min and was heated until the core temperature of the sausage reached 70°C. It was then cooled at room temperature for 20 min prior to further analysis.
Variable | Ingredients (%) | |||
---|---|---|---|---|
Meat | 60 | 60 | 60 | 60 |
Back fat | 20 | 20 | 20 | 20 |
Ice | 20 | 20 | 20 | 20 |
NPS | 1.2 | 1.2 | 1.2 | 1.2 |
Sugar | 1 | 1 | 1 | 1 |
Mixed spice | 0.6 | 0.6 | 0.6 | 0.6 |
WCF powder | - | 1 | 5 | 10 |
General and dietary fiber content analyses were conducted using AOAC (2000) as the standard. Moisture was measured with a moisture meter (MB45, Ohaus Corporation, Zurich, Switzerland) using a 105°C normal pressure and high-temperature drying method. The Kjeldahl nitrogen method was used to measure crude protein, and Soxhlet's extraction method was used to measure crude fat. Further, a direct method was used to measure ash after heating at 550°C-600°C, and dietary fiber was measured using the enzymatic gravimetric method.
The weight of the sausage was measured before and after cooking to calculate the cooking loss according to Equation 1:
To measure emulsion stability, a sausage emulsion (20 g) was transferred to a centrifuge tube, avoiding gas bubbles, and the tube was placed in a water bath at 80°C for 30 min. After 20 min incubation at room temperature, the volumes of water and fat collected in the centrifuge tube were measured separately, and the water and fat losses were calculated according to Equation 2 and Equation 3, respectively:
A pH value of the sausage batter was measured before and after cooking using a pH meter (F-51; Horiba, Kyoto, Japan). The sausage batter was prepared by placing 5 g of sample in 45 mL of distilled water, followed by homogenization using a homogenizer (Polytron PR-MR 2100; Kinematic AG, Littau, Switzerland) at 15,000×g for 1 min and filtration through filter paper (Cat No. 1002 110, Whatman, Westborough, MA, USA). Sausage pH values were measured three times.
Surface color measurements of sausage batter samples were performed before and after cooking. The color of each sample was determined using a Hunter color reader (CR-300; Minolta Co., Osaka, Japan) on cross-sections selected from five random locations of each sample. Lightness (CIE L*), redness (CIE a*), and yellowness (CIE b*) values were recorded.
A white standard plate with a lightness of 97.26, redness of −0.07, and yellowness of +1.86 was used as reference.
The shear force was measured using a texture analyzer (TA-XT2, Stable Micro System, Ltd., Haslemere, UK). A cooked sample was prepared at a size of φ2.5×1.0 cm for measurement. The analysis conditions were set to a pretest speed of 5 mm/s, test speed of 2 mm/s, return speed of 5 mm/s, trigger force of 5 g, and distance of 30 mm using a v-blade probe, and the measurement was repeated five times.
Textural properties of the sausage were measured using a texture analyzer (TA-XT2, UK). A cooked sample was uniformly cut to a size of φ2.5×2.0 cm and measured at room temperature. The analysis conditions were set to a pretest speed of 2.0 mm/s, posttest speed of 5.0 mm/s, test speed of 2.0 mm/s, distance of 8.0 mm, and trigger force of 5 g using a 75 mm probe compression plate for measurement. Measurements for hardness, adhesiveness, springiness, gumminess, cohesiveness, and chewiness were repeated five times.
The cooked sausage was cut to samples of a uniform size for evaluation by a panel consisting of 15 experts in sensory evaluation. For each sample, the color, flavor, tenderness, juiciness, and overall acceptability were evaluated; and the average scores were calculated. For each sample, a 7 point-scale method was used, and a score closer to 7 point reflected higher acceptability in terms of the color, flavor, tenderness, juiciness, and overall acceptability. This sensory evaluation was conducted after obtaining the approval from the institutional review board (IRB) of Sookmyung Women’s University (Approval Number: SMWU-1906-HR-037).
Statistical analysis of all data was conducted using SAS version 9.4 (SAS Institute, Inc., Cary, NC, USA). Results were presented as the mean±SD. To evaluate statistical significance, one-way analysis of variance (ANOVA) was conducted, followed by post-hoc analysis using Tukey's multiple-range test for significant results (p<0.05).
Results and Discussion
The general composition and total dietary fiber content in the WCFs are shown in Table 2. A freeze-dried WCF powder contained 7.34% moisture, 2.67% crude protein, 1.93% crude fat, and 6.90% ash. The total dietary fiber content was 21.28%.
The general composition of the WCF powder sausage is shown in Table 3. The moisture content increased in the sausage with the addition of WCF powder, compared to that in the control group (p<0.05). The crude protein content showed a decreasing trend with increasing concentrations of WCF powder and was significantly lower (p<0.05) than that in the control group. Additionally, the crude fat content decreased with increasing concentrations of WCF powder. Alternatively, the total dietary fiber content exhibited an increasing trend with increasing concentrations of WCF powder (p<0.05). Similarly, a previous study (Lee et al., 2018) reported that the addition of buckwheat at increased concentrations resulted in increased moisture and ash contents of sausage, while protein and fat contents showed decreasing trends. Ham et al. (2017) also reported that the addition of lotus rhizome at increased concentrations led to increased moisture and ash contents and decreased protein and fat contents. Furthermore, Kim et al. (2009) reported that the dietary fiber content affected moisture retention and the water-binding capacity. Further, Lee et al. (2008) reported that the binding capacity between meat tissue and added dietary fiber increased during sausage heat processing, serving to increase the moisture content. The results of this study showed that the addition of WCF powder increased the moisture content in sausages, compared to that in the control group, which could be due to a high total dietary fiber content in WCF. The total dietary fiber content in the WCF powder is 21.28%, of which soluble dietary fiber accounts for 8.38% and insoluble dietary fiber 12.90%. It is known that dietary fiber binds water molecules, thus increasing the water-binding capacity and water content (Han and Han, 1994). In turn, the water and dietary fiber contents of the WCF powder sausage could improve the cooking loss, emulsion stability, and texture of the emulsified sausage.
The results from the cooking loss analysis in WCF powder sausage are shown in Fig. 1. Cooking loss is caused by denaturation of the meat protein upon heat treatment, leading to a reduced binding capacity between water molecules and the protein. Further, cooking loss is associated with the meat pH (Choi et al., 2007). Within our study, increased concentrations of WCF powder led to a significant decrease in the cooking loss of the sausage (p<0.05). Moreover, the 10% treatment group exhibited the lowest cooking loss (11.04%), while the control group showed the highest (32.92%). These results may be due to the high dietary fiber content (viscous polysaccharide) in the WCF powder. Plant dietary fibers have a strong binding capacity for water molecules and can increase the retention capacity of water molecules by protein during heat denaturation of proteins, thereby reducing the cooking loss and increasing the emulsion stability (Kim et al., 2009). Lee et al. (2008) reported that the addition of dietary fiber to emulsified meat products can reduce the cooking, water, and fat loss. Ham et al. (2016) also showed that the addition of a Gaeddongssuk powder, with a high dietary fiber content, reduced the cooking loss of sausages. Similarly, increased concentrations of a black rice powder in sausages has been reported to reduce its cooking loss (Park and Kim, 2016); while Jeon and Choi (2012) have reported that an increased concentration of a seaweed powder, such as that of green algae, sea mustard, or hijiki, led to decreased cooking losses in processed meat products, similar to results observed in this study. Based on the study of insoluble polysaccharides in the Chunnyuncho fruit and stem (Yang, 2013), viscous fruit polysaccharides have appropriate physical properties to act as emulsion stabilizers in food processing. This study showed similar results to those of previous studies, which suggests that the viscous dietary fiber in the WCF affects the water retention and water-binding capacity of a sausage emulsion.
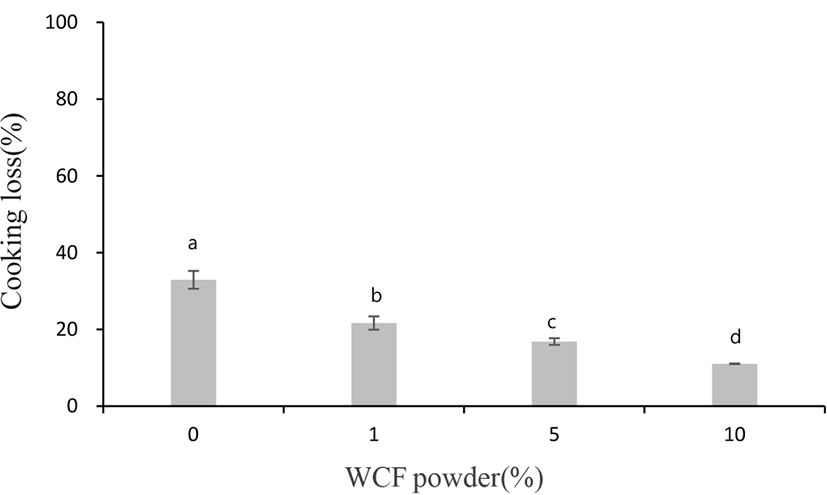
The emulsion stability results are shown in Table 4. Emulsion stability is the ability to maintain moisture, fat, and added moisture, which the meat originally had during the process of cutting and heating. Emulsion stability affects the meat color, texture, and succulence (Lee et al., 2010; Wu and Smith, 1987). The increase in WCF powder concentration led to a significant decrease in the water and fat loss of the sausage (p<0.05), with the group containing 10% WCF powder exhibiting the lowest water and fat loss. Previous studies (Ham et al., 2016; Lee et al., 2008; Park and Kim, 2016; Shin et al., 2017) have also reported decreased moisture loss with the increase in additives. Our findings suggest that the viscous dietary fiber present in the WCF binds moisture and fat to effectively reduce the moisture and fat loss from the emulsion.
Emulsion stability | WCF powder (%) | |||
---|---|---|---|---|
0 | 1 | 5 | 10 | |
Water loss (%) | 17.50±3.54a | 17.00±4.24a | 2.00±0.00b | 0.20±0.00b |
Fat loss (%) | 6.00±1.41a | 3.50±0.70a | 1.00±0.00b | 0.10±0.00b |
The results from the pH measurements of the WCF powder sausage before and after cooking are presented in Table 5. The pH value of the meat batter increased after cooking. In general, pH changes in food with protein substances are due to thermal denaturation of proteins (Shin et al., 2017). Lee et al. (2008) reported that hydrogen bonds in proteins are weakened by heat, which in turn leads to the release of a large amounts of ions from amino acid residues due to the thermal denaturalization of proteins.
pH values obtained before and after cooking showed a decreasing trend with increasing concentrations of WCF powder in the sausage (p<0.05). According to an alternate study on pork sausages (Ha, 2016), pH decreased with an increase in the added amount of a red beet powder, which contained the betalain pigment. Jin et al. (2011) also showed a decrease in the pH with an increase in the amount of a Baeknyuncho powder. Shin (2013) has reported that the presence of organic acids, including citric acid in the Chunnyuncho fruit powder caused a low pH value (5.07). The WCF powder used in this study had a pH of 5.16, which could lead to a decrease in the sausage pH following addition of increasing concentrations of WCF powder. Further, Devatkal (2010) showed that plant extracts with higher contents of phenolic compounds reduced pH of meat products to a larger extent.
The color measurement results are presented in Table 5. The sausage color after cooking showed that the CIE L* (lightness) and CIE b* (yellowness) values tended to increase, while the CIE a* (redness) decreased after cooking than before cooking. It has been reported that betanin is not affected by pH but is unstable when heated (Kim, 2006). According to the results of this study, the CIE a* of the sausage meat was measured to be higher before cooking than after. This may be attributed to the degradation of betacyanin due to heating and the expression of brown betaxanthin that caused an increased CIE b* after cooking. Additionally, depending on the amount of the fruit powder added, the CIE L* showed a decreasing trend (p<0.05), while the CIE a* increased (p<0.05) with the increasing concentrations of the powder. Meanwhile, the CIE b*, which was high before cooking, showed a decreasing trend (p<0.05), while there was an overall increase in the CIE b* accompanying the decrease in a value, caused by heat during cooking, and the CIE b* significantly increased upon fruit powder addition (p<0.05). Similarly, Jin et al. (2014) reported an increase in the b value following addition of red beet to sausage. Such changes in the color may be due to the presence of betaxanthin pigment from the betalain family (Lee et al., 2005).
The results of measuring the shearing force and evaluating the texture of the WCF powder sausage are shown in Table 6. The addition of the WCF powder led to a significant decrease in the shear force of the sausage (p<0.05). According to a study on Baeknyuncho powder sausage (Jin et al., 2011), an increase in the amount of the powder led to a decreased shearing force, similar to the results of this study. According to the reports by Virgili et al. (1995) and Monin et al. (1997), the shear force of raw ham decreased with the increased moisture content. Hence, the reduced shearing force observed in our study may be due to high moisture content in the treatment groups, which also affected the texture of the sausage.
The texture of meat products can be altered by thermal protein denaturation during processing. Further, it has been reported that the state of the source meat, addition of fat, and moisture, as well as the composition and form of additives, can change the texture of the product (Lee et al., 2004; Lee et al., 2008). We show that the WCF powder sausage also exhibited significantly decreased hardness with increasing concentrations of WCF (p<0.05). Moreover, the springiness, chewiness, and gumminess significantly decreased with an increase in the additive (p<0.05). Conversely, the data obtained by Lee et al. (2018) and Shin et al. (2017) showed that increasing the amount of additives led to an increased hardness, while Ham et al. (2017) observed decreased hardness in the treatment group, compared to that in the control; however, hardness of the sausage increased with an increased amount of the additive, which disagrees with the results of this study. Nevertheless, several studies (Ham et al., 2016; Park, 2016; Veram et al., 1984) have reported a decrease in sausage hardness with an increase in the amount of an additive, which is consistent with the results of this study. A softer sausage obtained in this study with the increase in the amount of the additive could be due to the ability of the dietary fiber and viscous material present in the WCF to increase the water binding capacity of the meat batter, thus decreasing the hardness of the sausage tissue.
The results of the sensory evaluation of the WCF powder sausage are presented in Table 7. In all categories, the WCF powder groups (1%, 5%, and 10%) received higher evaluation scores compared to the control group. The scores for color, flavor, tenderness, and juiciness were the highest in the 5% powder group (p<0.05), which may be due to the increased water binding capacity that in turn increased the juiciness. The 10% treatment group obtained a lower score in the tenderness category compared to the 5% treatment group due to its higher dietary fiber content. The 10% content in the WCF powder may have markedly decreased the hardness and led to the less pleasant texture of the sausage. The overall acceptability was significantly higher in the 5% powder group than in the other groups (p<0.05).
Conclusion
In this study, the effects of different concentrations of WCF powder were investigated on the quality parameters of an emulsified sausage. The moisture content and dietary fiber content the sausages were increased with the addition of WCF powder. Further, increasing the amount of the WCF powder served to decrease the cooking loss and emulsion stability, which increased the water binding capacity. Both before and after cooking, pH values and hardness of the sausage significantly decreased with addition of increased WCF powder. This result may be due to the presence of dietary fiber and viscous polysaccharides in the WCF, which prevents leakage of moisture and fat substances from the sausage and reduces hardness. Sensory evaluation resulted in higher scores in the groups with the addition of the WCF powder, compared to the control group, and the 5% powder group showed the best evaluation results. Therefore, the addition of the WCF to an emulsified sausage can result in an excellent meat product in terms of both physicochemical and sensory properties, with reduced moisture and fat losses from cooking. This study suggests the potential of the WCF as a natural additive in sausage processing.