Introduction
In the last few decades, tremendous improvements have been achieved in growth rate and breast yield of poultry birds, to meet the growing demand for poultry meat (Mudalal et al., 2015). Globally, the productivity of poultry meat has been enhanced by intentional genetic selection using traditional quantitative techniques (Zuidhof et al., 2014). The genetic selection was companied by histological and biochemical modifications in the muscular tissues of growing birds (Petracci and Cavani, 2012). It was found that genetically selected birds had low blood capillary vessels’ density which led to some disorders metabolism (Soglia et al., 2018a). Accordingly, this was companied by emergence of several muscle abnormalities such as Pale Soft exudative (PSE) (Petracci et al., 2017), Deep pectoralis myopathy (DPM). Recently, new muscle abnormalities have been observed such as white striping (WS) and hardening of the breast muscle known as ‘wooden breast’ (Kuttappan et al., 2017). Moreover, intramuscular connective tissue defects characterized by a loose structure of muscle fiber bundles called ‘spaghetti meat’, has been recently observed (Baldi et al., 2018; Maiorano, 2017). The previous poultry meat defects were attributed due to a consequence of substantial improvement towards increasing growth rate and breast yield (Petracci et al., 2015).
All previously mentioned defects in turkey and chicken meat (in particular breast meat) are considered as a serious problems to poultry industries because they affected adversely the quality traits of premium cuts (Soglia et al., 2018b). These defects impaired visual appearance as well as reduced technological properties such as water holding capacity, texture, and color. Accordingly, this was negatively reflected on consumer acceptance (Kuttappan et al., 2017). The classification systems for affected meat by muscle abnormalities are still based on aesthetic criteria (variations in the color of the meat, whether the meat is too pale or too red, and/or excessive fluid accumulation), couldn’t make an exact judgment to deal with meat quality issues (Barbut, 2009). The affected meat should be culled out from the processing line and transformed them for further processed meat (such as nuggets and sausages) while the rest of the carcass is suitable for human consumption (Brambila et al., 2017).
Differences in meat composition due to increase muscle abnormalities have imposed more pressure on the meat industries to guarantee good meat quality. Concerning production and meat evaluation, there is a need to look for rapid, non-destructive and non-expensive techniques.
Over the last years, the use of near-infrared spectroscopy (NIRS) as instrumental technique with spectrum wavelength (800–2,500 nm) has increased enormously. Near-infrared spectroscopy (NIR) has the ability to estimate and predict different quality traits in food products by measuring the amount of NIR radiation that is reflected, absorbed, transmitted, and/ or scattered at different wavelengths (Gardner, 2018).
NIR spectroscopy technique was employed to evaluate the chemical composition of meat and meat products (Van Kempen, 2001). It has unique advantages if compared with classical methods such as quick and frequent measurements, and the ease of sample preparation. Moreover, it is fit for on-line applications in the agriculture field (Abu-Khalaf, 2015; Beghi et al., 2018), pharmaceutical industries (Guillemain et al., 2017), as well as medical sectors (Monteyne et al., 2018) to assess different quality traits. On another hand, NIRS has still some limitations where there is a necessity for reference method, low sensitivity to minor constituents, as well as complexity in the calibration (Buning-Pfaue, 2003).
The ability of NIRS to predict several quality traits of meat such as chemical composition (protein, moisture, fat, and collagen), pH, water holding capacity, etc have been investigated (Brondum et al., 2000; Meulemans et al., 2002; Moran et al., 2018; Yang et al., 2018). Moreover, it was found that there was a possibility to classify meat based on feeding regimes (Cozzolino et al., 2002), strains (McDevitt et al., 2005), and tenderness (Yancey et al., 2010) by using NIR spectroscopy.
There are no available studies that used visible-near infrared (VIS/NIR) spectroscopy to predict the quality traits of turkey breast meat affected by different levels of WS. Therefore, the main objective of this research is to employ VIS/NIR spectroscopy to differentiate different levels of WS defects.
Materials and Methods
From a local Palestinian slaughterhouse near Tulkarm city (Palestine), more than 60 the pectoralis major muscles of 20-wk old tom turkey birds were randomly selected based on the appearance of white striations. The evaluation of the presence of WS was performed on the processing line at 1–2 h of post-mortem after the breast- deboning area. Out 60 pectoralis major muscles, 34 muscles were classified into three groups: normal (12 samples) (free of white striations), moderate (12 samples) (when white striations thickness <1 mm), and severe (10 samples) (when white striations thickness >1 mm) (Soglia et al., 2018b). Samples were subjectively pre-classified into categories, packed on ice, and transported to Palestine Technical University-Kadoori laboratory for VIS/NIR measurements then to An-Najah National University laboratories for other quality traits analysis. The pectoralis major muscles were excised from the whole breast muscle. Excessive fat, connective tissue, cartilage, and bone fragments were avoided to minimize sampling errors.
In each turkey breast meat sample (n=34), three spectra were collected (at a room with a temperature of 23±2ºC and relative humidity of 60%) directly on the skin side, radial section, and tangential section. A USB2000+ miniature fiber optic spectrometer (Ocean Optics, Largo, FL, USA) with a vivo light source and 50 μm fiber optics probe was used for spectra acquisition. The spectra were obtained at scans with a resolution of 0.35 nm full width at half maximum (FWHM), and spectra range 550–1,100 nm. It also has a 2048-element CCD-array detector, 2-MHz analog-to-digital (A/D) converter, in addition to a high-speed USB 2.0 port. The USB2000+ can be controlled by Spectra Suite software. This device is equipped with an active fan cooling to overcome the risk of sample overheating. The 4 halogen tungsten light sources make the vivo a high-powered VIS/NIR source, which allows a shorter integration time than conventional methods (Ocean Optics). The integration time used in this investigation was 1,340 μs. A total of 102 spectra were obtained for turkey samples, and then the average spectra were taken. The VIS/NIR analyses were performed in the diffuse reflectance mode and then recorded as absorbance (log 1/R). To ensure the stability of the measurements, a diffuse reflectance standard WS-1 (Ocean Optics) was used as the optical reference standard for the system every 5 minutes during the experiment. The dark reference was done once at the beginning of each experiment, by closing the entrance of incoming light from probe to the USB2000+ miniature fiber optic spectroscopy using a plastic cap. At the end of all spectral measurements, the acquired data were well stored for later analysis. The 34 samples were used for building PLS calibration models using their VIS/NIR spectra.
The Unscrambler program (version 9.7, CAMO Software AS, Oslo, Norway) was used for both principal component analysis (PCA) and PLS multivariate data analysis (Abu-Khalaf, 2015). In PCA, VIS/NIR spectra represented a bilinear model of the data matrix X. PCs represent in a pattern of observations in plots.
To investigate the possible differences in three types of turkey breast meat (normal, moderate and severe) at three ranges, i.e. VIS (550–700 nm), NIR (700–1,100 nm) and VIS/NIR (550–1,100 nm) wavelengths, a PCA model was carried out.
Results and Discussion
Typical mean spectral curves representing the three levels of WS fillets in the wavelength range 550–1,100 nm are shown in Fig. 1. The depressions and peaks in spectra showed the strong and weak absorbance characteristics of the samples, within the range of study. The spectra of normal, moderate (WS) and severe (WS) breast fillets showed similar absorption bands, which were in agreement with previous studies (Barbut, 1996; Fumiere et al., 2000).
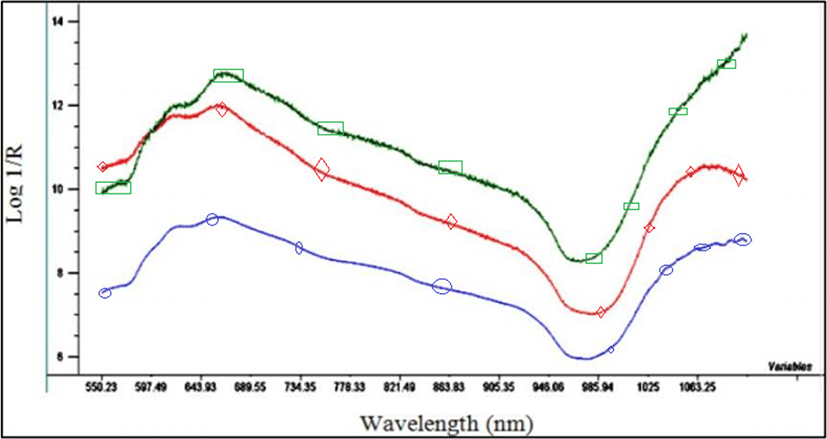
NIR spectra often contain undesired scattering variation due to heterogeneous content and sample surface amongst others. The scattering effect in NIR consists of a multiplicative effect and an additive effect. The additive effect is reflected as the baseline offset. The multiplicative effect is reflected as a slope that scales the entire spectrum. Data pre-treatment was employed to minimize these complex baseline variations and scattering effects. NIR spectra of the samples set were pre-processed using standard normal variate (SNV) to delete slope variation and to correct for scattering effects (Fig. 2). Light scattering in fresh meat samples does not always travel the same distance before it is detected. As a longer light traveling path corresponds to a lower relative reflectance value while more light is absorbed (Jens et al., 2019). This causes a parallel translation of the spectra. For that reason, multiplicative scatter correction (MSC) was used to eliminate these effects (Li and He, 2006) (Fig. 3). Savitzky-Golay first derivatives (1st D) was done to delete baseline flung in meat spectral data and small spectral differences were strengthened, this followed by Savitzky-Golay smoothing (Cunha et al., 2010) to prevent increasing the noise, which came from the derivative (Fig. 4; Li and He, 2006). The relative values of spectra may vary from sample to sample, which might be due to changes in surface texture and moisture content of three types of fillets (Mudalal, 2019; Soglia et al., 2018a; Soglia et al., 2018b).
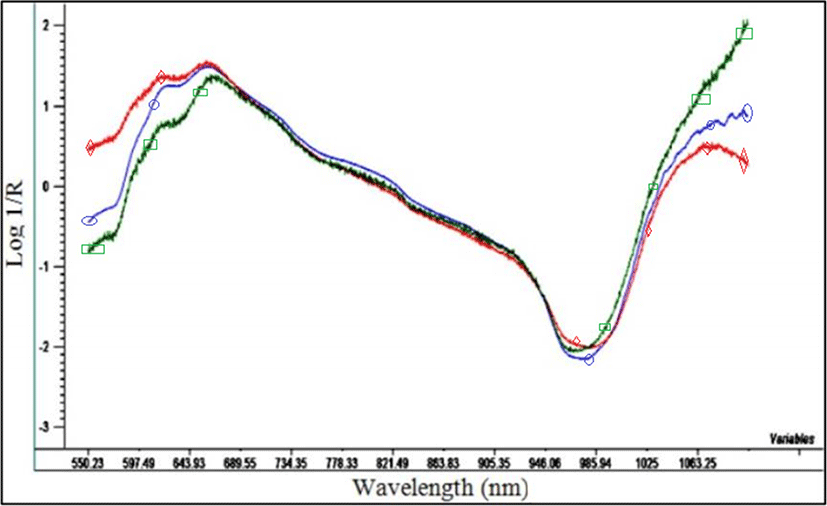
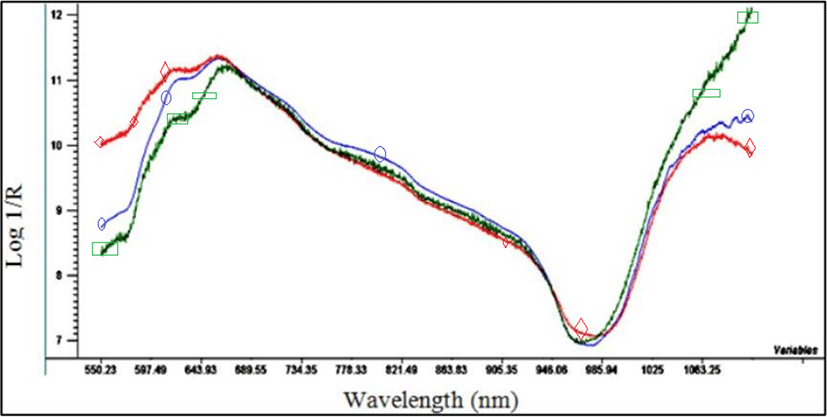
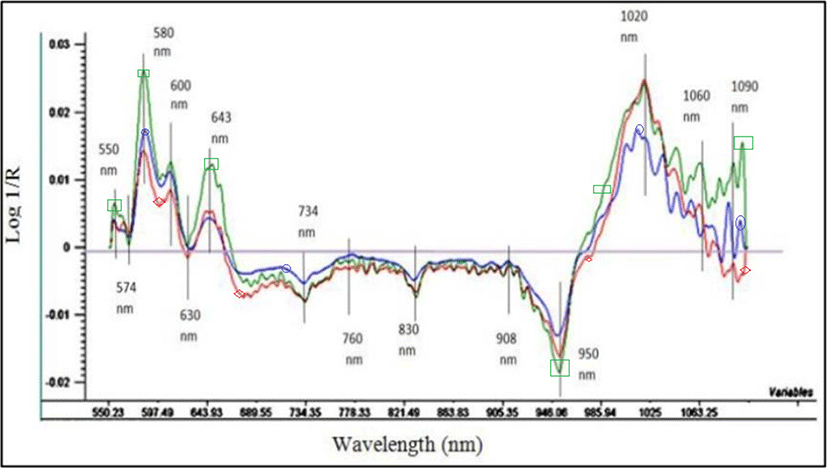
Six bands (peaks at 550, 574, 580, 600, 630, and 643 nm) in the visible region (550–700 nm) and eight bands in the NIR region have been observed (Fig. 4). Several researchers found similar bands and spectral features (Andres et al., 2008; Barlocco et al., 2006; De Marchi et al., 2012). Absorption bands at 550 to 580 nm were associated to the Soret band attributed to the traces of erythrocytes of myoglobin with both haemoglobin and oxyhaemoglobin absorption as well as to oxymyoglobin (Liu and Chen, 2000).
Our findings showed that severe and moderate WS fillets had higher absorption at 550, 574, and 580 nm than normal fillets. This result may be attributed due to discoloration over the surface of white striped fillets (Ellekjaer and Isaksson, 1992). The mean spectrum in the NIR region has absorption bands at 980 nm and it could be related to the second overtone of the OH- vibrational mode of water (Bowker et al., 2014). The absorption at 760 and 908 nm corresponds to the deoxhaemoglobin (Hollo et al., 1987) and the third overtones of C-H bonds, respectively. The identified band at 552 nm related to myoglobin (Cozzolino et al., 1996). Absorption band at 574 nm was associated with oxyhemoglobin (Mitsumoto et al., 1991). The absorption bands at 540 and 580 nm were associated with both myoglobin and oxymyoglobin, respectively (Cozzolino and Murray, 2004).
PCA has been carried for VIS/NIR regions spectrum considering the three levels of muscle abnormalities (normal, moderate and severe). PCA showed an ability to distinguish the three groups (normal, moderate WS and severe WS) from each other (Figs. 5–7).
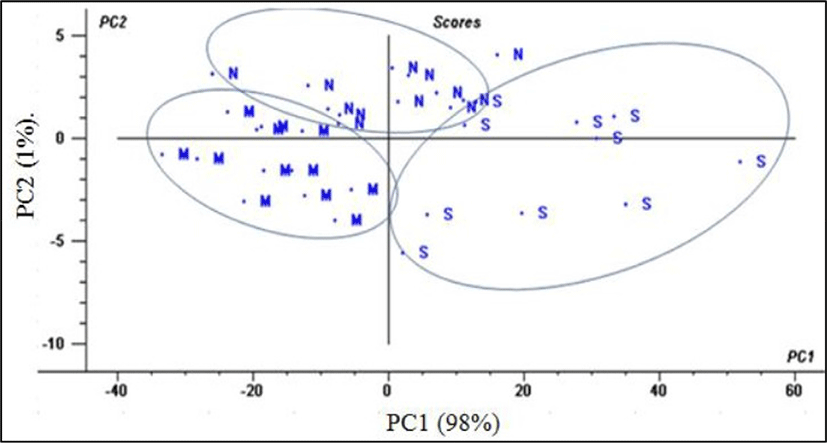
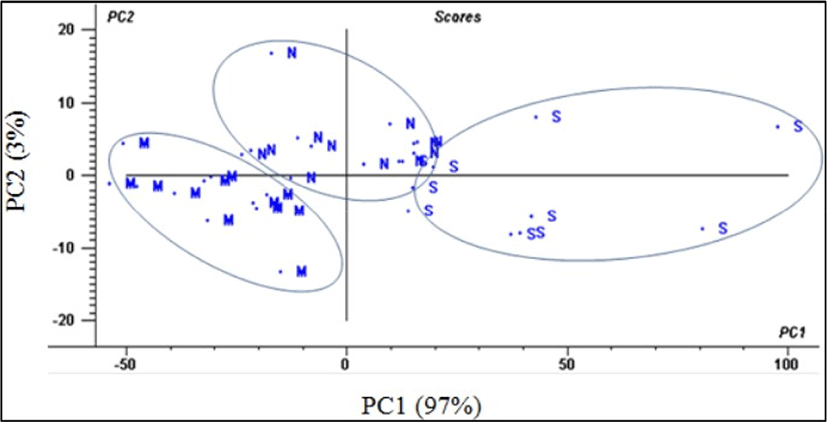
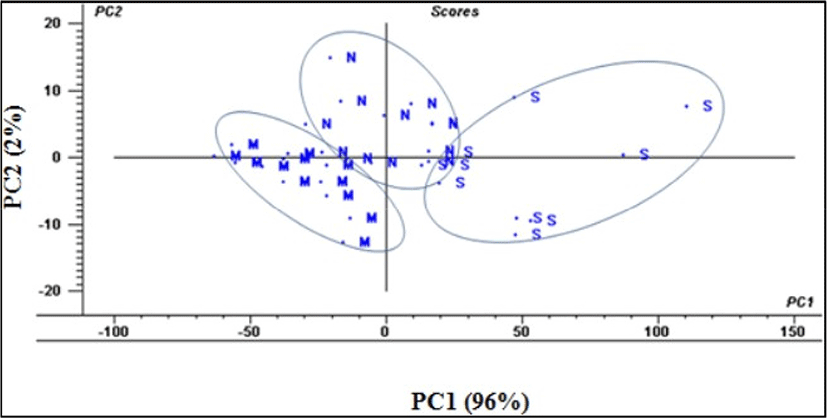
In this analysis, 2PCs for VIS, NIR and VIS/NIR region explained 99%, 100%, and 98% of the variance, respectively. Our findings showed that PCA had high performance in separating normal turkey breast meat from abnormal meat (moderate and severe). These results were in agreement with previous studies where VIS/NIR spectroscopy with PCA was used to separate poultry and meat products into different categories (Wold et al., 2017).
In conclusion, VIS/NIR spectroscopy is considered a quick, safe and nondestructive technique which is very suitable for online control for meat defects. The findings of this study showed that VIS/NIR spectroscopy was satisfactory to differentiate normal from severe WS turkey fillets. Moreover, the results open a wide door for using a portable VIS/NIR technique in the turkey industry. Further studies with a high number for samples are recommended to confirm the ability of VIS/NIR combined MVDA techniques to differentiate normal turkey breast meat samples from defect WS.