Introduction
Salt is a major ingredient in processed meat products owing to its functional capacities, including improving the emulsion stability, water holding capacity, textural properties, flavor, and shelf-life (Bowen et al., 1974; Seman et al., 1980; Watts, 1954). In particular, the protein extracted by salt in raw meat plays an important role in forming a stable gel matrix comprising insoluble fat and other components, resulting in a final product of high quality (Choe et al., 2017).
As an alternative source of salt, seawater has been utilized for the processing of various foods, including meat, for the curing of Baechu (Chinese cabbage), and in the formation of soybean curd (tofu), and has been shown to improve the quality characteristics (Ko et al, 2013; Ryu et al., 2014; Uttaro, 2011). Seawater contains sodium chloride (NaCl; about 80%), sulfates, and various minerals, including zinc, magnesium, calcium, and arsenic (Moon et al., 2004). The pH of seawater is largely dependent on the salt component and is generally around 8.3, which can improve the water holding capacity of meat products (Kim et al., 2016). As the number of negative ions increases as pH high, empty space creates water molecules to settle. However, to our knowledge, there has been no previous attempt at using seawater to improve the technological properties of emulsified meat products. Therefore, the objective of this study was to compare the effects of seawater and salt addition at different addition levels on the technological properties of a chicken sausage model system.
Materials and Methods
The seawater was provided by Yeorumul (Incheon, Korea), comprising 20% NaCl, 0.007% zinc, 3.3% sodium, 1.8% potassium, and 0.0004% arsenic. The chicken breast meat was obtained from a local butcher (Jungwoo-food, Pocheon, Korea). All of the subcutaneous and intramuscular fat, and visible connective tissues were removed from the chicken meat muscle. The meat was cut and ground using a grinder (PA-82, Mainca, Barcelona, Spain) equipped with 3 mm plates. The ground chicken meat (60%) was mixed with pork backfat (20%), iced water (20%), salt (1.2%), sugar (1%), and mixed spice (0.6%) in a bowl-cutter (K-30, Talsa, Valencia, Spain).
The samples were treated with seawater at three levels (10%, 15%, and 20%, respectively) in iced water (10%, 5%, and 0%, respectively) or with iced water and salt as the conventional method. The control is manufactured by adding 1.2% of NaCl and 20% of iced water, and the treatments were treated with seawater at three levels (10%, 15%, and 20%, respectively) in iced water (10%, 5%, and 0%, respectively). The meat emulsion samples were divided into two parts. One portion was used for measurement of pH values, protein solubility, cooking yield, emulsion stability, and viscosity, and the other port ion was stuffed into hog casings (Woosing Foodtec, Seoul, Korea) using a stuffer (EM-12, Mainca, Barcelona, Spain) and heated using a chamber (10.10ESI/SK, Alto Shaham, Menomonee Falls, USA) at 85°C until the internal temperature of the samples reached 75°C. The cooked sausage samples were subsequently cooled in iced water for 20 min, and then stored at 5°C until analysis (proximate composition, pH, and textural properties).
The proximate composition of each sample was analyzed as described by Choe and Kim (2019) following standard AOAC (2012) methods.
Each sample (4 g) was homogenized with 16 mL distilled water using a homogenizer (HMZ-20DN, Pooglim Tech, Seongnam, Korea). The pH value of the homogenate was then measured with a pH meter (Model S220, Mettler-Toledo International, Greifensee, Switzerland).
Cooking yield was determined as the percent weight of each meat sample after cooking. The samples were heated in a water bath at 85°C for 35 min until reaching an internal temperature of 72°C and then cooled in ice water. Cooking yield was determined using the following formula:
The manufactured emulsifiers were sealed a glass tube with the wire cut to 4×4 cm. After heating the glass tube in the chamber (10.10ESI/SK, Alto Shaam, Menomonee Falls, WI, USA) for 35 min at 85°C, it was cooled at 10°C for 1 h. After cooling down completely, the amounts of water and oil were measured, and the loss value was used to determine the emulsion stability according to the following formula:
Protein solubility (total, myofibrillar, and sarcoplasmic proteins) of the meat emulsion was determined as described by Choe et al. (2017). In brief, for assessment of the total protein content, 2 g of the raw sample was added to 20 mL of 1.1 M potassium iodide and 0.1 M potassium phosphate (pH 7.4). The sarcoplasmic protein was quantified by adding 2 g of the raw sample to 0.025 M potassium phosphate (pH 7.4). For both methods, the samples were homogenized for 2 min and incubated overnight at 2°C. The samples were then centrifuged at 4,032×g for 15 min at 4°C and filtered using Whatman No. 1 filter paper to prepare for quantification. The absorbance of the sample was measured at 540 nm to obtain the optical density (OD) value using the Spectra-photometer (SpectraMax iD3, Molecular devices, Sanjose, USA), and the protein content in each sample was determined by according to the following formula:
The flow behavior and time dependence of the batter for each sample were investigated at 20±1°C using a rotational viscometer (MerlinVR, Rheosys, Princeton, USA). The meat emulsion was allowed to equilibrate for 5 min at room temperature (25±1°C), and the time dependency of the meat emulsion viscosity was determined by measuring the apparent viscosity under a constant shear rate of 13.44×g for 60 s.
To determine the texture profile of the cooked sausage samples, the samples were cut into cubes of 2.5×2.5×2.0 cm (width×length×height) and placed in the Texture Analyzer system (TA 1, Lloyd, Largo, FL, USA) equipped with a 25 mm cylinder probe. The analysis conditions were set to a pre-test speed of 2.0 mm/s, post-test speed of 5.0 mm/s, head speed of 2.0 mm/s, distance of 8.0 mm, and force of 0.4 N. The measured hardness (N), springiness, and cohesiveness were recorded, and the gumminess (N) and chewiness (N) were calculated using these values.
Relevant protein levels were assessed with SDS-PAGE analysis using the method of Ossipow (1993) using a 12% separating gel and 4% stacking gel. The supernatants of the samples were mixed with 3 mM phosphate buffer and 5X sample buffer. Fifteen microliters of each sample was added to each well of the gel and processed for 1 h and 20 min. The gel was removed and fixed in a fixing solution, incubated overnight using a rocker, and stained with Coomassie brilliant blue for 20 min with gentle agitation. The dye was removed with a destaining solution for 1 h, and the gel was stored in a storage solution and scanned.
All data were analyzed using one-way ANOVA in SAS (version 9.3), and the results are expressed as mean values with standard deviations. Significant differences (p<0.05) among the mean values were determined using Duncan’s multiple range test. For pH values, the differences between raw and cooked samples between groups were tested with the independent samples t-test. Correlation coefficients between variables (proximate composition, pH cooking yield, emulsion stability, protein solubility, and TPA) were tested using the Pearson correlation coefficient.
Results and Discussion
The addition of seawater and the amount of seawater added affected the proximate composition of the emulsified sausage (Table 1). The moisture and fat contents were not significantly affected by the level or addition of seawater, but the crude protein and ash contents differed significantly according to the presence and the level of seawater addition. The seawater-treated groups showed significantly higher crude protein contents compared to those of the salt-treated control group. In addition, the ash content was significantly the highest in the group processed with 20% seawater. This could reflect the natural salt and ash content in the seawater.
The presence and level of seawater addition did not influence the pH in the samples either before or after cooking, but the cooking treatment significantly increased the pH (p<0.05 or <0.001) in all treatment groups (Table 1). This increase in pH from cooking is due to the non-reactive alkaline amino acids generated by the heat-induced denaturation of the proteins in the emulsion, thereby releasing large amounts of cations (Forrest et al., 1975).
The presence and level of seawater addition clearly affected the cooking yield of the sausage (Table 2). The group treated with 10% seawater showed a lower cooking yield compared to that of the other seawater groups and the salt-treated control group (p<0.05), whereas there was no significant difference in cooking yield between the control group and the 15% and 20% seawater groups. Therefore, the addition of 15% or more seawater resulted in similar retention ability as the addition of salt. Froning and Sackett (1985) reported that increasing the concentration of NaCl added to turkey breast muscle increased the protein solubility, which in turn increased the cooking yield, consistent with the present findings. In this study, as shown in Table 3, the protein solubility (total and myofibrillar protein) was increased (p<0.05) as increasing the addition level of seawater and the group with over 15% seawater induced higher (p<0.05) protein solubility compared to group with salt. However, the significant difference in protein solubility did not affect cooking yield (p>0.05). It could be speculated that salt and seawater have the different degree of myofibrillar protein swelling capacity affecting water holding capacity of meat emulsion, depending on content of chloride (Gordon and Barbut, 1992).
With respect to the emulsion stability, the addition of seawater affected the water loss of the sausage, but it did not affect fat loss (Table 2). The group treated with 10% seawater showed the highest water loss, whereas the group treated with 20% seawater showed the lowest water loss, and both effects were statistically significant. This suggests that seawater has similar salt-soluble protein extraction capacity, emulsion formation capacity, and protein-binding capacity to those of salt (Kim et al., 2010). Kwak et al. (2010) reported that the salt-soluble protein derived from anchovies could effectively separate fat and water during the production of emulsified sausage to produce a stable emulsion, which would influence the salt-soluble protein measurement as discussed further below in the next section. Surh et al. (2006) also reported that samples with high emulsion stability showed minimal separation of water and fat, which allowed for the production of a high-quality meat product.
Protein solubility is a key factor in sausage production for producing a high-quality product. During the production of emulsified sausage, improvement in the protein solubility allows for formation of a stable emulsion, which in turn enhances product characteristics such as moisture retention power, yield, and texture (Aminlari et al., 2009; Marcos et al., 2010). According to Briskey and Sayre (1964), enhancing the content of salt-soluble proteins increases the binding power during the production of meat products to ultimately improve the retention power and texture. The group treated with 10% seawater showed similar total protein and salt-soluble proteins to those of the control group (Table 3). However, groups treated with 15% or 20% seawater showed higher total protein and salt-soluble proteins. This difference in total protein solubility may be related to the difference in the salt-soluble proteins between treatment groups (Table 3; R2=0.72).
Xiong et al. (2000) found that the addition of increased concentrations of NaCl also increased the content of water-soluble proteins. Kim et al. (2003) reported that the addition of NaCl in DFD meat increased the total protein solubility, which improved the quality of the meat product. Therefore, addition of more than 15% seawater could improve the quality of the sausage.
The changes in apparent viscosity according to the presence and the level of seawater addition are shown in Fig. 1. The groups treated with 15% and 20% seawater showed significantly higher viscosity of 102.91 Pa·s and 128.34 Pa·s, respectively, at 60 s compared to that of the control group and the 10% seawater group (96.71 Pa·s and 101.33 Pa·s, respectively). In general, the control group showed the lowest viscosity values, and an increasing trend with increased seawater addition was observed. This suggests that the increased salt from seawater acted on the myofilaments of the meat protein to extract salt-soluble proteins, which further stabilized the water, protein, and fat (Park and Kim, 2016).
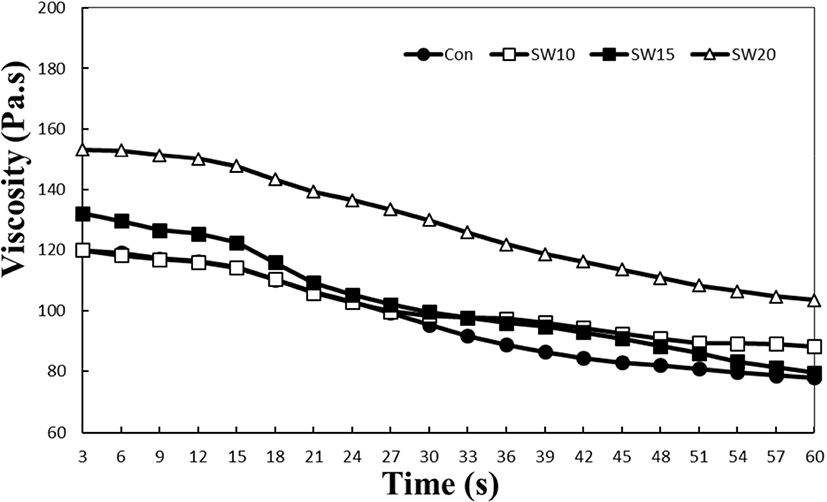
Excluding cohesiveness, the other physical properties (hardness, springiness, gumminess, and chewiness) of the control group were significantly lower than those of the seawater treatment groups (Table 4). Hardness, gumminess, and chewiness values were the lowest in the control group and the highest in the 20% seawater group. Samples treated with 10% and 15% seawater showed the lowest levels of cohesiveness (p<0.05), and samples treated with 20% seawater had the highest cohesiveness values (p<0.05).
Moreover, the solubility of total, water-soluble, and salt-soluble proteins was closely correlated to the physical characteristics of the sausage. The solubility of total protein and salt-soluble protein was significantly correlated with hardness (R2=0.83 and R2=0.56, respectively), springiness (R2=0.99 and R2=0.25), cohesiveness (R2=0.30 and R2=0.84), gumminess (R2=0.77 and R2 =0.77), and chewiness (R2=0.94 and R2=0.62), which increased with addition of seawater, leading to formation of a stable emulsion. Fat loss and hardness were negatively correlated, which suggests that an increased fat content leads to reduced hardness (Table 5).
Myosin and actin are important proteins that contribute to the main functional properties of myofibrillar protein (Chan et al., 2011). Furthermore, myosin heavy chain (MHC) and tropomyosin in a protein-based gel or emulsion are associated with the gel-forming capacity of comminuted meat products (Benjakul et al., 2004; Kamath et al., 1992). The addition of seawater and the seawater level induced different protein patterns (Fig. 2). The band of MHC appeared in the 15% and 20% seawater-treated samples, whereas no such band appeared in the control and 10% seawater samples. This difference can explain the improvement of viscosity, protein solubility (total and myofibrillar protein), and some of the textural properties with seawater addition. C-protein, which is affected by actinidin and a component of myosin filaments (Aminlari et al., 2009; Asghar et al., 1985), was much more strongly expressed in the 15% and 20% seawater samples compared to the control and 10% seawater samples. There was no difference in the extent of actin expression among the treatments. In general, tropomyosin, which is related with protein solubility (Grossi et al., 2016), was not affected by the addition of seawater. However, for the upper band of tropomyosin, the control and 10% seawater groups showed a slightly stronger band density compared with those in the 15% and 20% seawater groups.
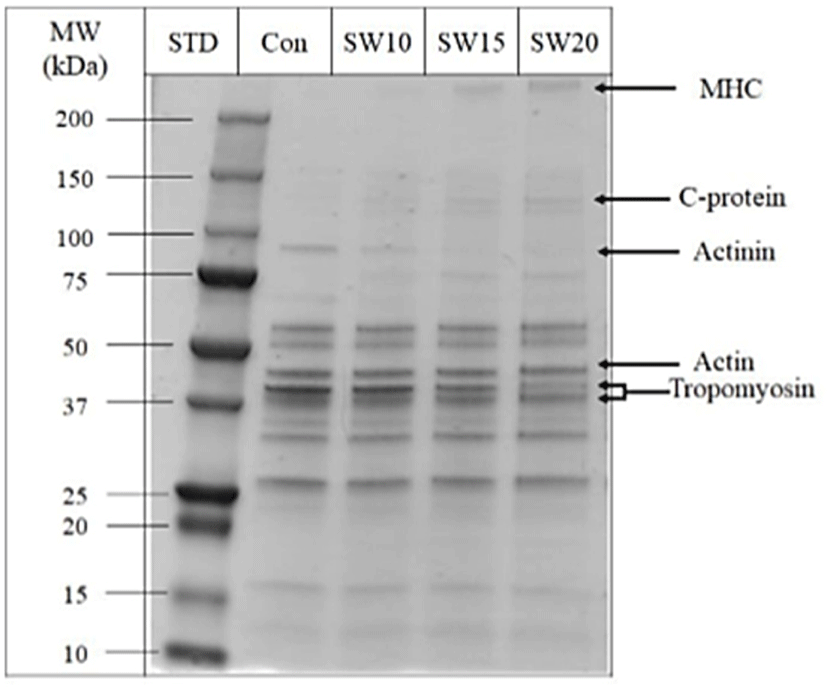
Conclusion
The results of this study suggest that seawater can be used as a source of salt in emulsion-type meat products when added at ≥15% without an adverse effect. Moreover, the addition of 20% seawater can enhance the technological properties of chicken emulsion sausages, including the total and myofibrillar protein solubility, viscosity, and textural properties compared to the conventional use of salt. Furthermore, seawater with chicken meat would probably result in better than those that use salt.