Introduction
Since consumers tend to select high quality of food rather than a high quantity of food with increased economic development, the development of processed meat with natural ingredients has been focused on functional meat products (Cardenia et al., 2011; Choe et al., 2013). These functional properties include antioxidant and antimicrobial activities as well as other bioactive actions such as reducing blood pressure and increasing immunity. It is known that lipid oxidation of meat products caused by autoxidation and hydrolysis deteriorates the quality of food. The autocatalytic chain reaction of active oxygen and free radicals can produce peroxides such as wide range of carbonyl compounds, furans, and hydrocarbons (Kanner, 1994). These secondary oxidative products have undesirable effects on the quality and nutritional value of products such as odor, texture, color, and flavor (Fernández et al., 1997).
Therefore, antioxidants should be used to delay lipid oxidation in many foods since antioxidants can interfere with the formation of free fatty acids and thus inhibit oxidation reactions (Kelleher et al., 1992). Many synthetic antioxidants such as butylated hydroxyanisole (BHA), butylated hydroxytoluene (BHT), and propyl gallate (PG) have been used as antioxidants. However, they might have been found that these synthetic antioxidants induce toxicity and carcinogenic (Branen, 1975; Hirose et al., 1998). In particular, Festjens et al. (2006) reported that BHA shifts cell death from necrosis to apoptosis. Thus, application and research of natural antioxidants to meat products have been actively carried out (Biswas et al., 2012; García-Lomillo et al., 2017).
The color of meat or meat products is a specific visual appearance characteristic that affects consumer choice at the point of purchase of the meat (Ngapo et al., 2007). Consumers prefer pink in cured meat products, so color formation is one of the important sensory properties of meat products that affect consumption (Zhang et al., 2007). To these consumers’ preferences, colorants are added to meat products, but due to allergenic and intolerance reations to synthetic colorants, consumers have increased interest in natural colorant, which is associated with a healthy image (Østerlie and Lerfall, 2005).
Cudrania tricuspidata Bureau (CT) is a deciduous arboreous of the moraceae tree with blooms in May-June. The color of this fruit is red or purple. Its leaves have been used as food for silkworms, while its berries are used for food and medicinal purpose (Sol, 2006). Many studies have been performed on CT’s physiological activities such as cytotoxic (Kim et al., 2012), antibacterial (Choi et al., 2009), and antiallergic (Oh et al., 2009) activities. CT leaves extracted with ethanol and methanol were applied to pork meat patties to extend shelf life (Cuong and Chin, 2018). However, there was not much study on the antioxidant activity as affected by particle size of the CT leaves and fruit powder. The direct incorporation of natural powder from CT fruit to processed meat products may be an effective and economic strategy to control the oxidation of meat products. Furthermore, it is expected that the red color of meat products will be enhanced due to the color of CT fruits. This study was performed to evaluate the antioxidant activities of CT leaves (CTLP) and CT fruit powder (CTFP) as affected by different particle size, and determine antioxidant activity as determined by thiobarbituric acid reactive substance (TBARS) and peroxide values (POV), physicochemical properties and microbial counts of pork patties with different levels of CTFP during refrigerated storage.
Materials and Methods
CT fruits and leaves harvested in September 2018 were purchased at local commercial markets (Hampyeong, Korea). After removing discolored and damaged parts, the samples were washed with water before being dried. Leaves were dried with hot air in an oven at 60°C and fruits were dried using a freeze dryer under a pressure of 7 mm Torr at –50°C. These dried leaves and fruits were ground using a grinder (HMF Electric -3260s, Seoul, Korea). Then, some of the homogenized CT leaves and fruits were kept unfiltered in polyethylene bags (CTLP, CTFP). After the rest were sieved with the size of 500 μm mesh sieve (ChungGye Industrial Mfg., Seoul, Korea), some of the sieved powder was stored in a polyethylene bag (CTLP 500 μm, CTFP 500 μm). Another part of the sieved powder was sieved again to a size of 150 mesh sieve, and then the filtered powder was stored in a polyethylene bag (CTLP 150 μm, CTFP 150 μm). All powders were stored at –70°C and used in the experiment. 1,1-Diphenyl-2-picrylhydrazyl (DPPH), Folin-Ciocalteau reagent, ethylenediaminetetraacetic acid (EDTA), 2-thiobarbituric acid (TBA) and ammonium thiocyanate were all purchased from Sigma-Aldrich (Steinheim, Germany). L (+) Ascorbic acid, ferrous chloride, ferric chloride, gallic acid, trichloroacetic acid, petroleum ether and barium chloride anhydrous were purchased from Junsei Chemical (Tokyo, Japan). Ferrous sulfate heptahydrate was supplied by Duksan Pure Chemicals (Ansan, Korea). Potassium ferricyanide was purchased from Avocado Reserch Chemicals (Lancaster, UK). Methanol (99.5% purity) was obtained from OCI Company Ltd. (Seoul, Korea).
Total phenolic content was determined using the method described by Lin and Tang (2007). The sample solution was prepared at a concentration of 10 mg/mL. Approximately 2 mL of 2% (w/v) sodium carbonate was added to 0.1 mL of each sample in a test tube. Then, 1 mL of Folin-Ciocalteau reagent (previously diluted 2-fold with distilled water) were added and shaken. The mixture was allowed to stand at room temperature for 30 min. Absorbance was measured at a wavelength of 750 nm. Contents of phenolic compounds were then calculated using a standard curve of gallic acid at 0–200 mg/L concentrations and expressed as gallic acid equivalents / 100 g of dried powder weight.
DPPH radical scavenging activity was determined with the method of Huang et al. (2006). Briefly, 0.5 mL of DPPH (0.2 mM in methanol) was added to 2 mL of extract solution at different concentrations diluted with dd-water (0, 1, 2.5, 5.0, and 10 mg/mL). The mixture was left to stand for 30 min in darkness. Its absorbance was measured at a wavelength of 517 nm against a blank. Ascorbic acid was used as the reference (REF). DPPH-radical scavenging activity was calculated with the following equation: DPPH radical scavenging activity (%) = (1−A/B) × 100, where A was the absorbance of the sample and B was the absorbance of the control.
Iron chelating ability was determined using the method described by Le et al. (2007). After preparing CTLP and CTFP samples at different concentrations diluted with dd-water (0, 1, 2.5, 5.0, and 10 mg/mL), 0.5 mL of each sample, 0.1 mL of FeCl2 (0.6 mM in water), and 0.9 mL of methanol were mixed and reacted at room temperature for 5 min. After 0.1 mL of ferrozine (5 mM) was added, the mixture was kept at room temperature for 10 min. Absorbance was measured at 562 nm. EDTA was used as a positive control. Iron chelating ability was calculated with the following equation: Iron chelating ability (%) = (1−A/B) × 100, where A was the absorbance of the sample and B was the absorbance of the control.
Reducing power was determined using the method described by Huang et al. (2006). Briefly, 2.5 mL of K3Fe (CN)6 (1% (w/v)) and 2.5 mL of 200 mM sodium phosphate buffer (pH 6.6) were added to each sample (0, 1, 2.5, 5.0, and 10 mg/mL) dissolved in distilled water. Reaction mixtures were mixed well and incubated at 50°C in an oven (LDO-250F, Labtech, Namyangju, Korea) for 20 min. After 2.5 mL of 10% (w/v) trichloroacetic acid was added, mixtures were centrifuged at 1,476×g for 10 min. From the upper layer, 2.5 mL solution was mixed with 2.5 mL of distilled water and 0.5 mL of ferric chloride (0.1% (w/v)). The mixture was then incubated at room temperature for another 10 min. Absorbance was then measured spectrophotometrically at 700 nm. Ascorbic acid was used as a reference treatment.
The individual antioxidant components were determined by the method of Kwon et al. (2016) with slight modificaions. A 9 g of CTLP and CTFP were weighed each, extracted with 90 mL of 80% methanol on a magnetic stirrer for 24 h at room temperature and centrifuged (VS-5000N, Vision Scientific, Seoul, Korea) at 2,214×g for 10 min to collect the supernatant. This operation was repeated 3 times. The supernatants were pooled an condensed using a rotary evaporator (R-100, Buchi, Flawil, Switzerland) to remove methanol at 40°C. Then, the concentrate was dissolved in 5 mL with methanol. All samples were passed through a 0.5 μm filtermembrane for HPLC analysis Analytic HPLC was conducted on a LC-20AVP HPLC system (Shimadzu, Kyoto, Japan) fitted with a C18 reverse-phase (5 μm) column (250×4.6 mm). A mixture of water/acetonitrile, 90:10 (A), and 10: 90 (B) was used as a mobile phase. The 1.0 mL/ min flow rate was kept constant during the measurement and an injection volume of 20 μL in all experiments. The gradient elution was performed as following protocol: 0–2 min, 100% A, 6–31 min 10%–63% B, and 41–45 min 50%–100% A. The compounds were tested at the same wavelengths (nm) 280 for gallic acid, ferulic acid, chlorogenic acid, rutin, and isovanillic acid standards. These compounds were eluted from the column with retention time of 4.2, 10.8, 13.4, 15.3, and 17.0 respectively. The quantification of the phenolic and flavonoid compounds from CTLP and CTFP was performed by comparing standard spectra at each retention time.
Pork ham used in the manufacture of pork patties added with CTFP was purchased from a local meat market (Samho, Gwangju, Korea). After removing connective tissue and fat, hams were ground through a grinder (Meat chopper, M-12S, Hankook Fujee Industries, Korea) using grinder plates of 5 mm hole diameter. After additives were added and mixed thoroughly with a mixer (EF20, Crypto Peerless, Birmingham, UK), the sample was re-homogenized using a grinder with 8 mm hole diameter grinder plate, divided into 65–70 g, and homogenized again. The sample was then shaped using a petri dish. The formulation of pork patties added with CTFP is shown in Table 1. Patties were made without (CTFP-0) or with 0.1 g/ 100 g of ascorbic acid (REF), 0.5 g/ 100 g of freeze-dried CTFP with size below 500 μm (CTFP-0.5), and 1.0 g/ 100 g of freeze-dried CTFP with size below 500 μm (CTFP-1.0). These prepared pork patties were stored in a polystyrene box at 4±1°C for 14 days. The different measurement was done initially and at days 3, 7, 10, and 14 of the storage. Patties were analyzed for color, pH, TBARS, and POV. All experiments were repeated three times.
The color of each pork patty sample was measured using a color meter (CR-10, Minolta, Tyoko, Japan) using an 8 mm measuring aperture size, with a CIE standard illuminant D65 and 10° observer.
The patty surface was repeatedly measured six times and the average value was calculated. Lightness (L*), redness (a*), and yellowness (b*) values were shown. The pH of pork patty sample was measured using a pH-meter (Model 120, Mettler-Toledo, Greifensee, Switzerland). The pH value of this experiment was obtained as an average value after repeating the measurement five times.
Levels of TBARS were determined using the method, according to Sinnhuber and Yu (1977). TBARS indicates fatty acid level by measuring O.D. values after adding TBA reagent, which reacts with malondialdehyde (MDA) and expresses red color. Briefly, 2 g of homogenized meat sample was blended with 3 mL of 1% (w/v) TBA and 17 mL of 2.5% (w/v) trichloroacetic acid in glass tubes. The blank sample consisted of 17 mL of 2.5% (w/v) trichloroacetic acid, 3 mL distilled water. The mixture was then heated at 100°C for 30 min. After mixing 5 mL of chloroform and 5 mL of the reacted sample in a conical glass tube by vortexing, the mixture was centrifuged at 1,476×g for 5 min. Then 3 mL of the supernatant from each a conical glass tube and 3 mL of petroleum ether were mixed and centrifuged again at 1,476×g for 10 min. The absorbance of the supernatant was then read at a wavelength of 532 nm. TBARS values were calculated using the following equation:
9.48 was a constant derived from the sample dilution factor and the absorption coefficient (152,000 M–1 cm–1) of the red TBA reaction product.
POV was determined according to the method of Shantha and Decker (1994). Briefly, 0.3 g of pork patty sample was homogenized with a mixture of 5 mL of chloroform and methanol (ratio of 1:1 v/v). After mixing 3.08 mL of 5 (mg/mL) sodium chloride and the homogenized sample in a tube, the mixture was vortexed for 30 seconds and centrifuged at 1,476×g for 5 min. After centrifugation, 2 mL of the supernatant was added to the bottom of a tube and mixed with 1.33 mL of chloroform and methanol (1:1, v/v). Then 25 μL of ammonium thiocyanate reagent (30 g / 100 mL) and iron (II) chloride were added to the sample, respectively. The mixture was mixed for 5 s and incubated at room temperature for 20 min. The absorbance of the mixture was then measured at a wavelength of 500 nm against a blank that contained all the reagents except the sample. A standard calibration curve was used with a concentration range of 0.1–1.0 μg/ mL. POV was determined as milliequivalents per kilogram of fat.
Total plate count (TPC) agar (Difco, Becton, NJ, USA) and violet red bile (VRB) agar (Difco) were prepared for the determination of pork patties containing CT fruit of total bacterial counts and Enterobacteriaceae. A 10 g of each sample was transferred to sterile vacuum package pouches containing 90 mL of water. Then, the pork patties in the pouch were homogenized for 20 s using a laboratory stomacher Lab-Blender (Bagmixer 400, Interscience, St-Nom-la-Bretèche, France). Dilutions (10−1, 10−2, and 10−3) were prepared and spread onto TPC and VRB agar plates. Then, plates were incubated at 37±1°C for 48 h. Then, the number of colonies was counted. The result was represented by using log10 colony-forming units per gram (CFU/g).
All experiments were repeated three times. All statistical analyses were performed using the SPSS 22.0 software program (SPSS, Chicago, IL, USA). For antioxidant activities of CT leaves and fruit, the data were analyzed using 2-way ANOVA as factors for treatments [reference, CTLP, CT fruit powder (CTFP), CTLP 150 μm, CTFP 150 μm, CTLP 500 μm, CTFP 500 μm] and concentration (0, 1, 2.5, 5.0, and 10 mg/mL).
For pork patties added with CT fruit, the data were determined using two-way ANOVA by treatments (CTFP-0, REF, CTFP-0.5, CTFP-1.0) and storage time. Duncan’s multiple range test was carried out to calculate the significant difference between the means. Differences of p<0.05 were considered to be significant.
Results and Discussion
Total phenolic contents and antioxidant activities of oven-dried CTLP and freeze-dried CTFP were analyzed according to particle size (Table 2). Contents of total phenolic of all concentrations quantified ranged from 1.49 to 3.54 (g/100 g dry weight). CTLP was higher (p<0.05) than those in fruits regardless of particle size. Crude CTLP showed the highest total phenolic content among all samples (p<0.05). In the total phenolic content result of the CTFP, there was no difference in the according to the particle size (p<0.05). The smaller the particle size of plants with antioxidant properties, the larger the surface area, so the antioxidant capacity was well transferred to the solvent (Castiglioni et al., 2015). However, as the surface area of substances increases, O2 can affect the stability of the antioxidant material (Hu et al., 2012). Choi et al. (2009) have determined total phenolic content in methanol extracts of various parts of CT tree and observed higher content of total phenolic in leaves compared to fruit. Hong and Hong (2015) have also reported that the fruit of CT has lower levels of total phenolic than leaves in either ethanol or water extract. Memon et al. (2010) have also reported that levels of total phenolic compounds in fruits are lower than those in leaves regardless of mulberry species. They reported to the amount of antioxidants such as ferulic acid are present in leaves more than in fruits. However, in this study, the CTFP contained more ferulic aicd than the CTLP, whereas the CTLP had more rutin content than the CTFP (Table 3).
Treatments1) | ||||||
---|---|---|---|---|---|---|
CTLP | CTFP | CTLP 150 μm | CTFP 150 μm | CTLP 500 μm | CTFP 500 μm | |
Total phenolic contents (g/ 100 g dry matter) | 3.54±0.40a | 1.85±0.15c | 2.80±0.08b | 1.49±0.08 c | 2.80±0.21b | 1.91±0.29c |
Treatment | Individual antioxidant components1) | ||||
---|---|---|---|---|---|
Gallic aicd | Ferulic acid | Chlorogenic acid | Rutin | Isovanillic acid | |
CTLP | ND | 91.81b | ND | 191.56b | ND |
CTFP | ND | 185.45a | ND | 21.24a | ND |
In this study, we confirmed that the difference of antioxidative activities of the CT leaves and fruit, which was dried by the oven-drying method and freeze-drying method, respectively. As shown in Fig. 1A, DPPH radical scavenging activity of CTFP was higher than that of CTLP. Among samples of fruits with different particle sizes, those with a particle size of less than 150 μm (CTFP 150 μm) showed the highest radical scavenging activity, among the treatments (p<0.05). There was no significant (p>0.05) difference in DPPH radical scavenging activity between CTLP at a particle size of 500 μm or less (CTLP 500 μm) and CTLP at a particle size of 150 μm or less (CTLP 150 μm). However, the leaves powder sample with crude particle size had lower (p<0.05) DPPH radical scavenging ability than leaves powder sample with particle size less than 500 μm. Chon et al. (2009) have reported that DPPH radical scavenging activity of leaves powder sample was higher than those of fruit powder samples. These results present that even if the fruit has lower total phenolic content than leaves, DPPH radical scavenging activity might be higher. Csepregi et al. (2016) explained that different types of phenols might lead to different consequences due to different structure-activity relationships between polyphenol substituents and antioxidant activity results. Therefore, it seems that the difference in the types of phenol contained in leaves and fruit influenced the result of DPPH radical scavenging activity in this study (Natić et al., 2015).
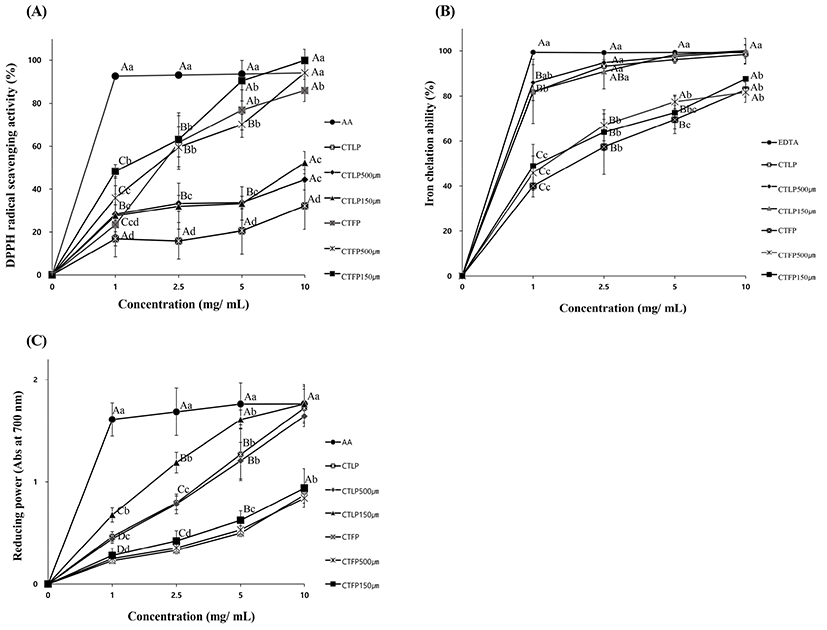
Iron chelating ability is one of the methods to confirm the antioxidant capacity. It is known that Fe (II) possesses the ability to speeds up the generation of hydroxyl radical through the Fenton reaction (Rastogi et al., 2009). Ferrozine combines with FeCl2 to form a complex, resulting in purple color. Chelating agents may serve interrupted complex formation, resulting in a reduction in the purple color of the complex. Measurement of absorbance of the purple color reduction, therefore, allows estimation of the iron chelating activity (Gülçin et al., 2011). In the present study, the chelating ability of the CT leaves and fruits powder toward ferrous ions was investigated. Results of iron chelating ability are shown in Fig. 1B. CTLP had higher (p<0.05) iron chelating ability than CTFP. All leave samples showed no significant (p>0.05) difference in iron chelating activity from EDTA at concentrations of higher than 0.1% (w/v). It has been reported that total phenolic and flavonoids contents in CTLP were higher than those in CT fruits (Choi et al., 2009). These contents can affect the iron chelating ability (Natić et al., 2015). At a concentration of 1.0% (w/v), CTFP samples showed more than 80% iron chelation ability. The result of iron chelation activity according to particle size showed no difference (p>0.05) in activity regardless of CT leaves or fruit powders. Our results suggest that the particle size does not affect iron chelating ability. Ganjewala et al. (2013) have determined the iron adsorption power of methanol extract leaf and fruit of Kadam. They found that leaf was higher than the fruit and the reason was that leaf has higher contents of total phenolics in these tissues.
Results of reducing power are presented in Fig. 1C, similar to the results of iron chelating ability CTLP had higher reducing power than CTFP (p<0.05). The reducing power of CTLP with particle size less than 150 μm was higher than that of other treatments but slightly less than that of ascorbic acid (p<0.05). However, at 1.0% (w/v), leaves powder had similar reducing power to fruit powder, regardless of particle size (p>0.05). In fruits, no difference in reducing power by particle size was observed, regardless of the concentration (p>0.05). The reducing power increased with increasing concentration for all particles (p<0.05) except for CTFP 150 μm (p>0.05). Natić et al. (2015) reported that reducing power had a positive correlation with total phenolic content. In this study, the total phenolic contents of CT leaves treatments showed higher than those of CT fruits treatments (p<0.05). Moreover, since CTLP contained more rutin (Table 3), the reducing ability of CTLP seems to higher than CTFP (Firuzi et al., 2005). Jeong et al. (2009) have reported that the reducing power of 100°C water extract from different parts of CT tree showed high results in the order of leaves, fruit, root, and stem. Among various parts of CT tree extracted with hot water, leaves had an excellent ability to react with and stabilize active oxygen, similar to the results of the present study.
In order to establish CTLP and CLFP in terms of bioactive compounds and antioxidant activity in this study, they were analyzed by HPLC. The HPLC analysis of phenolic acids in CTLP and CTFP presented in Table 3. Comparison with individual antioxidant components including gallic aicd, ferulic acid, chlorogenic acid, rutin, isovanillic acid allowed the extracted compounds to be detected and quantified by HPLC. Rutin and ferulic acid were detected in CTLP and CTFP. Out of the two phenolic compounds, rutin was found in the highest concentration compared to ferulic acid amounting 191.56 mg / 100 g of dry sample in CTLP. In contrast, CTFP detected more ferulic acid than rutin. Both rutin and ferulic acid showed to contribute to the antioxidant activity of natural antioxidant resources and which have health benefits (Abarikwu et al., 2015). These results indicated that CTLP and CTFP could be a potential source of antioxidants.
Pork patties were prepared by adding 0.5 and 1.0 g/ 100 g of CTFP. Physicochemical properties and fatty acid compositions of pork patties were then measured during refrigerated storage. As shown in Table 4, the pH of pork patties with the addition of fruit powder did not interact between treatments and storage periods (p>0.05). There were no significant (p>0.05) differences in the pH between CTFP-0 and treatments added with CTFP. As the storage period increased, the pH also increased for all treatments. Lee and Kim (2015) have shown that the pH and total acid of CT fruit are 6.05 and 0.31 mg/mL, respectively. Thus, the addition of CTFP did not affect the pH of pork patties in this study.
As shown in Table 4, there was no interaction between the amount of CTFP added and the storage period. Pork patties with CTFP had lower lightness (L*) values than the CTFP-0 and reference. Redness (a*) and yellowness (b*) values were higher (p<0.05) as the amount of CTFP added was increased. On the other hand, there were no differences in lightness, redness, or yellowness during storage (p>0.05). According to Jo et al. (2017), when colors of ripe CT were measured, CIE values of L*, a*, and b* were 33.41, 26.80 and 16.57, respectively. Thus, the colors of pork patties were affected by the addition of CTFP. Yong (2015) has found that the red color of CT fruit was mainly induced by carotenoid pigment. The CT fruit was found to show the presence of four components. Among these components, antheraxanthin was identified. Sasaki et al. (2008) have investigated meat color changes after adding fucoxanthin, a significant carotenoid in algae, to patties of chicken breasts. They found that fucoxanthin decreased the lightness (L*), but increased redness (a*) and yellowness (b*). These results were similar to those of the present study.
As shown in Fig. 2A, interaction (p<0.05) between storage days and pork patty treatment was observed in the result of TBARS. From day 10, CTFP-0 had higher TBARS values than other treatments (p<0.05), and the pork patties containing ascorbic acid, CTFP-0.5 and CTFP-1.0 treatments were not different (p>0.05). TBARS of CTFP-0 increased as the storage period increased. However, after adding CTFP and ascorbic acid, TBARS did not show any significant change for 14 days, indicated a delayed lipid oxidation activity (p>0.05). Results of TBARS of pork patties containing CTFP-0.5 and 1.0 for all storage periods showed no significant difference. The phenolic compound such as ferulic acid and rutin in CTFP (Table 3) have the ability to decrease the value of TBARS (Vaisali et al., 2016). Also, there are various phenolic compounds in CTFP (Choi et al., 2009; Hong and Hong, 2015; Yong, 2015), that can lower lipid oxidation (Singleton et al., 1999). Tapp et al. (2012) have added noni (also called Indian mulberry) to beef patties at different concentrations and then measured TBARS during the storage period. As a result, it has been reported that the antioxidant capacity of noni delayed lipid oxidation, thereby the expiration date could be prolonged.
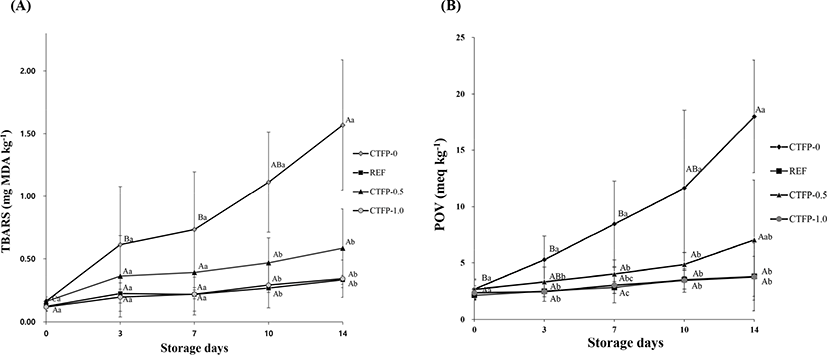
Fig. 2B shows the results of POV of pork patties added with 0.5 and 1.0 g/ 100 g of CTFP according to the storage period of pork patties. The POV showed an interaction between storage period and treatment (p<0.05). CTFP-0 showed no significant (p>0.05) difference in POV from the beginning of storage to 10 days after storage, however it showed higher TBA values than others thereafter. The patties with CTFP-0.5 increased POV up to 7 days after storage and showed no differences thereafter, however the addition of CTFP-1.0 did not show any significant difference in POV from the beginning of storage to the end of storage (p>0.05). CTFP-0 had significantly higher POV than other samples during all storage days (p<0.05). In contrast, no significant difference in POV was observed between pork patties added with the reference, CTFP-0.5 and CTFP-1.0 during the storage period (p>0.05). Thus, the ability of CTFP to inhibit peroxidation were determined. Yong (2015) reported that much chlorogenic acid among the phenolic compounds in CT powder was considered to have influenced the results of this study (Sasaki et al., 2010).
Table 4 presents results of microbial counts of pork patties added with CTFP during 14 days of storage under 4°C in a refrigerator. There was no interaction between the two factors (treatment and storage day) (p>0.05). During storage, TPC and VRB were increased (p<0.05). However, the addition of CTFP did not show any antimicrobial effect among all treatments (p>0.05). There was no significant change in TPC or VRB until seven days of storage (p>0.05). However, microbial counts were increased significantly at 10 and 14 days after storage (p<0.05). There was no significant difference in TPC and Enterobacteriaceae between CTFP-0 and patties added with CTFP, which had no antimicrobial effect. Choi et al. (2009) have reported that methanol extracts of CT fruit had antimicrobial activities against Staphylococcus aureus, Bacillus cereus, and Enterobacter aerogenes. The reason for no antimicrobial effect of CTFP added to pork patties in this study could be related to the use of dry powder instead of an extract with an organic solvent such as methanol and ethanol.
Conclusion
This study was carried out to evaluate the antioxidant activity of freeze-dried CT fruit, and oven-dried CT leaves according to different particle size (500 μm, 150 μm). Physicochemical properties and antioxidant activities of pork patties added with CT fruit were also determined. Total phenolic content was higher in leaves than in fruits (p<0.05). There was no significant difference in the content of total phenolic according to particle size (p>0.05). In the antioxidant activity, DPPH radical scavenging activity of CT fruit was higher than that of CT leaves (p<0.05). Iron chelating ability and reducing power of CT leaves were also higher than those of CT fruit (p<0.05). Based on pH and color values measured after applying CT fruit particles of 500 μm or less at a concentration of 0.5 or 1.0 g/ 100 g to pork patties, there was no interaction between treatment and storage period. Results of lightness (L*) showed that samples without adding CT fruit had lower L* values than those added with CT fruit, while redness (a*) and yellowness (b*) values were increased for pork patties added with CT fruit (p<0.05). Since the redness increased as CT fruit was added to meat products, it was confirmed that it could be used as a natural colorant that can be applied to other meat products. Lipid oxidation was lower on treatments using CT fruit during storage, and this result was confirmed by a low value of TBARS and POV (p<0.05). Consequently, the antioxidative activity of freeze-dried CT fruit was lower than that of oven-dried CT leaves. Although CT fruit was added to pork patties at a low concentration of only 0.5 g/ 100 g, it showed a positive effect in retarding lipid oxidation and color. Thus, CT fruit might be useful as a natural antioxidant and colorant, so it is considered that further research on the development of meat products using CT fruit should be conducted.