Introduction
Extracellular vesicles (EVs) are nanosized, nonreplicating biological vesicles that are released from cells into extracellular space (Pegtel and Gould, 2019; van Niel et al., 2018). EVs contain cargoes of mRNAs, noncoding microRNAs (miRNAs), proteins, and lipids within a lipid bilayer, which are transported from donor cells to recipient cells and play an important role in intercellular interactions (Veziroglu and Mias, 2020). The structure and microscopic images of EVs are shown in Fig. 1. Recently, EVs have been classified into several subtypes, including ectosomes, exosomes, and apoptotic bodies, depending on their biogenesis, size, and release pathways (Zaborowski et al., 2015). However, there is no well-established separation method that clearly distinguishes among these subtypes, and with no consensus on specific markers, sufficient evidence is still needed for characterization and should be used with caution (Théry et al., 2018).
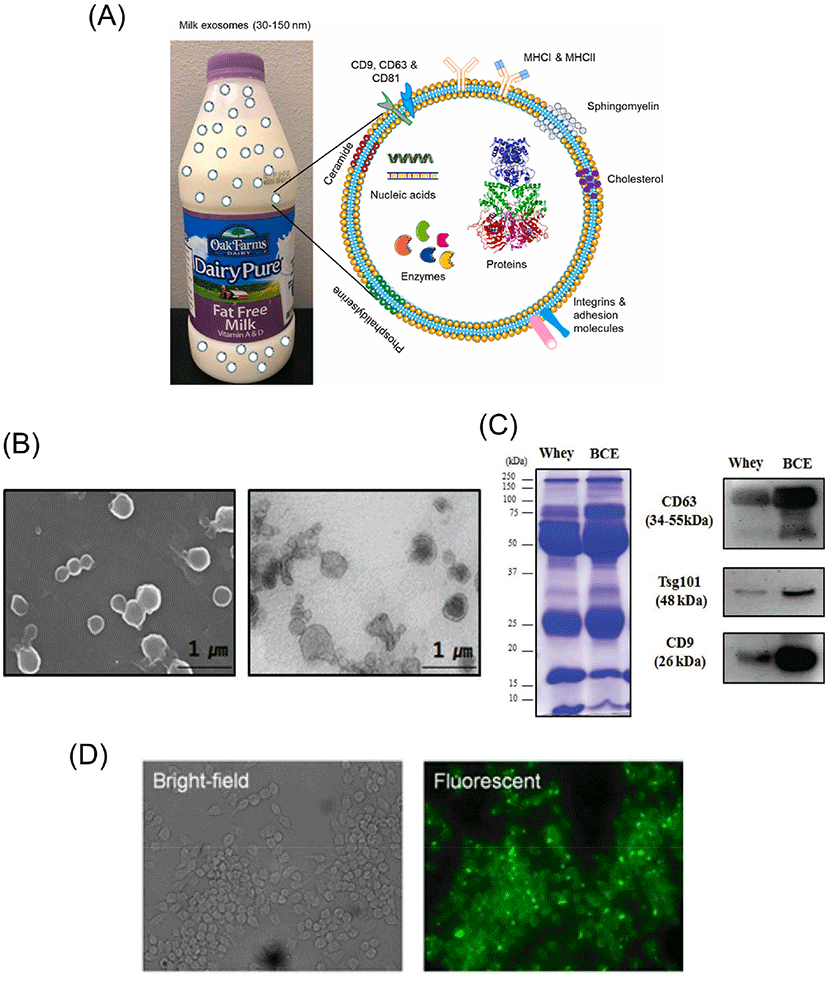
Bovine milk is one of the world’s most consumed foods, is high in nutritional value and contains a large amount of physiologically functional substances (Haug et al., 2007; Scholz-Ahrens et al., 2020). Most of high abundance nutrients, such as protein and fat, in milk have already been characterized, and research on EVs, which are minor components of milk, has rapidly increased over the past decade as the functionalities of EVs and their cargos are being revealed (Ong et al., 2021). In addition, since milk is a food that can be consumed by humans, it is safe and the production volume is abundant, so the mass production of EVs is possible (Somiya et al., 2018). Therefore, studies on the function of milk-derived EVs are being conducted in various research fields (Aarts et al., 2021; Adriano et al., 2021). The objective of this review is to summarize the recent findings in understanding the cargo, uptake and stability, potential use as a carrier, and therapeutic effects of bovine milk-derived EVs.
Cargo of Bovine Milk-Derived Extracellular Vesicles
miRNAs are noncoding RNAs of approximately 18 to 24 nucleotides that bind to a specific region of the mRNA and regulate it translation. Chen et al. (2010) found for the first time that substantial amounts of miRNAs are present in bovine milk and identified 245 miRNAs in raw milk and found that they are altered during lactation. miRNAs known to be involved in immune regulation were confirmed to be expressed both in bovine colostrum and mature milk exosomes. When miRNAs from colostrum and mature milk exosomes were compared, the ratio of the 10 most highly expressed miRNAs was different (Yun et al., 2021). Izumi et al. (2012) published a study comparing miRNAs from bovine colostrum and mature milk using microarray and qPCR and found that there are more miRNAs related to immunity or development, including miR-15b, miR-27b, miR-106b, and miR-223, in colostrum than in mature milk. The same researchers also found that miRNAs present in milk exosomes could be taken up by human macrophages (Izumi et al., 2015). Benmoussa et al. (2020) reported that miRNAs in milk EVs isolated from differential ultracentrifugation exhibited distinct compositions. In particular, the general length (around 22 nt) of miRNA sequence was abundant at milk EVs isolated by ultracentrifugation at 100,000×g, but the heterogeneous iso-miRNA was more abundant in milk EVs separated by ultracentrifugation at 12,000×g and 35,000×g. Additionally, when milk EVs were incubated with HeLa cells, miR-223, known to regulate immunity, was increased. To examine the possibility of using miRNAs as biomarkers, miRNAs from cow milk infected with Staphylococcus aureus were analyzed, and 14 or 18 miRNAs, including bta-miR-142-5p and bta-miR-223, were found to be differentially expressed in infection conditions (Cai et al., 2018; Sun et al., 2015). The composition and ratio of exosomal miRNAs from cow milk of two different breeds were also different. In Holstein milk, miRNAs related to milk synthesis and Doğu Anadolu Kirmizisi’s milk were expressed in milk fat and milk protein metabolism, respectively (Özdemir, 2020). Changes in feed and differences in breed of cattle have also been shown to alter milk miRNAs. Four miRNAs were upregulated and 5 miRNAs were downregulated when a fiber-rich diet was replaced with a nonforage fiber source diet (Quan et al., 2020). Inducing stress by relocation of the cow group have been shown to affect the composition of milk exosomal miRNAs. In particular, whether miRNAs and target genes such as miR-142a, miR-135, and miR-320a would be useful as potential biomarkers on response of mild stress has been postulated (Colitti et al., 2019). The functions and target miRNAs from milk-derived EVs are summarized in Table 1.
Description | Targeted miRNAs | References |
---|---|---|
Identification of miRNA in bovine colostrum and mature milk and proposal of potential biomarkers for quality control of raw milk and dairy foods. | miR-26a, miR-26b, miR-200c, miR-21, miR-30d, miR-99a, and miR-148a could potentially be used as biomarkers for quality control in raw milk and dairy products | (Chen et al., 2010) |
Identification and comparison of exosomal miRNA composition in human, caprine, and bovine colostrum and mature milk. | miR-30a-5p, miR-22-3p, and miR-26a are highly conserved in colostrum and mature milk of three mammals, including humans, caprine, and bovines. | (Yun et al., 2021) |
Identification and comparing miRNAs in bovine colostrum and mature milk using microarray and qPCR analysis. | miR-15b, miR-27b, miR-34a, miR-106b, miR-130a, miR-155, and miR-223 are related to immunity or development and are more expressed in colostrum than mature milk. | (Izumi et al., 2012) |
Identification of miRNA and mRNA of bovine milk exosomes and confirmation of uptake into human macrophages. | Among the analyzed miRNAs, 14 bovine-specific miRNAs (miR-2478, miR-2412, miR-2305, miR-2881, miR-2328*, miR-2888, miR-2304, miR-2391, miR-2892, miR-2887, miR-2316, miR-2374, miR-2291, and miR-2284l) were recognized. | (Izumi et al., 2015) |
Comparison of miRNA distribution in milk EVs according to different ultracentrifugation. | The top 4 miRNAs of P12K and P35K were bta-let7a, bta-miR-30a, bta-miR-21, and bta-let7b, and bta-mir-10a and bta-mir-10b were most expressed in P70K and P100K, respectively. | (Benmoussa et al., 2020) |
Identification and characterization of milk exosomal miRNAs in cows with mastitis. | Eighteen miRNAs, including four novel miRNAs (bta-let-7b, bta-miR-103, bta-miR-142-3p, bta-miR-142-5p, bta-miR-1468, bta-miR-146a, bta-miR-146b, bta-miR-147, bta-miR-221, bta-miR-223, bta-miR-2284w, bta-miR-2285b, bta-miR-23a, bta-miR-423-5p, etc.), had differential expression, and miR-223 and miR-142-5p were considered for use as potential biomarkers for the diagnosis of mastitis. | (Cai et al., 2018) |
Profiling of expression of milk exosomal miRNA in cows infected with Staphylococcus aureus. | According to the presence or absence of infection, 14 miRNAs (bta-miR-142-5p, bta-miR-296-5p, bta-miR-223, bta-miR-1246, bta-miR-183, bta-miR-502b, bta-miR-378b, bta-miR-2285 g-3p, bta-miR-99a-5p, bta-miR-181b, bta-miR-101, bta-miR-10a, bta-miR-99b, and bta-miR-2419-5p) were expressed differently, in particular bta-miR-142-5p, and -223 were suggested as potential biomarkers for detection of infection. | (Sun et al., 2015) |
Confirmation of changes in the expression of bovine milk EVs-miRNA as a result of changes in feed. | When fed on a nonforage fiber sources diet, 4 miRNAs (bta-miR-29c, bta-miR-760-3p, bta-miR-383, and bta-miR-11973) among milk EVs miRNAs were upregulated, and 5 miRNAs (bta -miR-328, bta-miR-10167-3p, bta-miR-103, bta-miR-885, and bta-miR-302d) were downregulated. | (Colitti et al., 2019) |
In milk-derived EVs, not only miRNAs but also proteins play a role in cell-to-cell communication and physiological activity (Fig. 1C). According to Reinhardt et al. (2012), a total of 2,107 proteins were identified in milk-derived EVs, of which 1,002 could be converted to David Resources. The same researchers also analyzed milk proteins from healthy cattle and from cattle infected with S. aureus. Interestingly, 2,350 proteins were identified in milk exosomes, and proteins related to host immunity and inflammation, including the heat shock protein family, protocadherin gamma family, and acute phase protein, were differentially expressed in S. aureus infection (Reinhardt et al., 2013). As a result of proteomics analysis of exosomes isolated from bovine colostrum and mature milk, a total of 9,430 proteins were detected in four samples, and 1,264, 1,404, 963, and 1,306 proteins were identified in colostrum and mature milk exosomes at 24 h, 48 h, and 72 h, respectively (Samuel et al., 2017). A total of 162 proteins were identified from exosomes and EV-containing pellets isolated through acid precipitation and ultracentrifugation, 43 of which were unique (Brown et al., 2020). When human and bovine milk exosomal proteins were analyzed and compared using the iTRAQ-coupled LC-MS/MS method, a total of 920 exosomal proteins were identified, and 575 proteins were differentially expressed. In particular, it was found that the proteins from human and bovine milk exosomes were clearly distinguishable (Yang et al., 2017). When the milk EV proteins from bovine leukemia virus-infected cattle were compared to that of uninfected cattle, a total of 1,330 proteins were detected in the milk EVs of infected cattle, of which 118 were uniquely expressed in the infected cattle. In addition, 26 proteins were expressed at significantly higher or lower levels than those of uninfected cattle. Through these studies, the possible use of proteins in EVs as a biomarker for specific diseases was revealed (Rahman et al., 2021). As a result of analyzing the lipids of EVs isolated by sequential ultracentrifugation and density gradient centrifugation through mass spectrometry-based lipid analysis, 8 major lipid classes were detected, and more than 200 fatty acid variations were identified (Grossen et al., 2021). Although it has been revealed through several previous studies that the lipids of EVs play an important role, there have been few lipidomics studies of bovine milk-derived EVs. This is because, due to the difficulty in reliably separating milk-derived EVs from milk lipids, there is still a limitation to the lipid analysis of milk EVs itself (Feng et al., 2021; Ong et al., 2021).
Uptake and Stability of Bovine Milk-Derived Extracellular Vesicles
As the physiological effects of milk-derived EVs were revealed, various experiments were conducted to confirm whether they could be leveraged as therapies. In particular, it was confirmed that EVs and their cargo were not degraded in the harsh digestive environment encountered when milk EVs were ingested (Benmoussa et al., 2016; Izumi et al., 2012; Shandilya et al., 2017; Zeng et al., 2019). It was also confirmed in vitro (Hansen et al., 2020; Izumi et al., 2015; Maburutse et al., 2017; Rani et al., 2017; Roerig et al., 2021; Wolf et al., 2015), in vivo (Kirchner et al., 2020; Manca et al., 2018), or under both conditions (López de Las Hazas et al., 2022; Munagala et al., 2016) that these EVs and their cargo are taken up (Fig. 1D). Additionally, a study examining whether the integrity of EVs is affected during the processing of raw milk has also been reported (Kleinjan et al., 2021; Shome et al., 2021). A summary of these papers is shown in Table 2.
Description | References |
---|---|
Bovine milk-derived EVs are uptaken into the intestinal cell model (Caco-2) and have more beneficial features than liposomes. | (Roerig et al., 2021) |
EVs-encapsulated miRNA was stable in both in vitro and in vivo digestion, and it was confirmed that cellular uptake was possible and reached each organ upon oral administration to mice. | (López de Las Hazas et al., 2022) |
The drug-loaded bovine milk exosomes showed higher efficiency and targetability compared to the free drug in both cell and mouse tests. | (Munagala et al., 2016) |
Fluorophore-labeled bovine milk exosomes and miRNAs are uptaken by human colon carcinoma cells and rat small intestinal cells, which are mediated by endocytosis. | (Wolf et al., 2015) |
Milk exosomes crossed intestinal epithelial cells in in vitro culture, and milk exosomal miRNAs can resist in vitro digestion and cross the intestinal barrier. | (Rani et al., 2017) |
When fluorophore-labeled bovine milk exosomes and miRNA were orally administered, it was expressed in the organs of mice and pigs, confirming that interspecies transfer was possible. | (Manca et al., 2018) |
Postprandial blood analysis of newborn calves showed that bovine colostrum-derived EVs were transferred into the circulation but not miRNAs. | (Kirchner et al., 2020) |
Confirmation of uptake of bovine milk EVs into intestinal epithelial cells through fluorophore-conjugated lactadherin labeling. | (Hansen et al., 2020) |
Confirmation of bovine milk exosome uptake into differentiated THP-1 cells by flow cytometry and fluorescence microscopy. | (Izumi et al., 2015) |
Confirmation of uptake of PKH-labeled bovine colostrum-derived EVs into RAW 264.7 cells | (Maburutse et al., 2017) |
Resistance of miRNA and mRNA in milk with acidic conditions and RNase treatment. | (Izumi et al., 2012) |
Bovine milk exosomal long noncoding RNAs are protected from degradation during in vitro digestion system. | (Zeng et al., 2019) |
Bovine milk exosomal small interfering RNA resists in vitro digestive conditions and is uptaken into Caco-2 cells. | (Shandilya et al., 2017) |
bta-miR-223 and bta-miR-125b present in commercial cow milk are resistant in a computer-controlled gastrointestinal model (TIM-1). | (Benmoussa et al., 2016) |
The integrity and molecular structure of EVs in bovine milk are affected by Industrial processing. | (Kleinjan et al., 2021) |
Processing in commercial dairy plants have no statistical effect on the abundance value of miRNAs in dairy products. | (Shome et al., 2021) |
Drug Delivery as Biological Carrier of Bovine Milk-Derived Extracellular Vesicles
Milk EVs may play an important role in drug delivery because of their beneficial properties, such as mass production due to the industrial scale of milk, passage of biological barriers, resistance in the digestive tract, etc., and supporting studies have been reported (Adriano et al., 2021; Kandimalla et al., 2021b; Sedykh et al., 2020; Zhong et al., 2021). An approach to efficiently treat diseases such as cancer by loading small interfering RNA or drugs into bovine milk EVs is being performed. The related papers are shown in Table 3.
Description | References |
---|---|
Confirmation of cellular and intestinal uptake of milk exosomes loaded with curcumin, which is poorly absorbed. | (Komine-Aizawa et al., 2020) |
By attaching hyaluronan, a CD44-specific ligand, to doxorubicin-loaded milk exosome, it enables specific transport of CD44 overexpressing cancer cells and induces tumor cell death. | (Li et al., 2020) |
Development of a pH- and light-sensitive drug delivery system based on milk exosomes for highly active anticancer treatment of oral squamous carcinoma. | (Zhang et al., 2020) |
Ultrasonically loaded bcl-2 siRNA into bovine milk exosomes crossed the cell membrane and inhibited tumor growth. | (Tao et al., 2020) |
Development of efficient and nontoxic lactoferrin-poly-l-lysine mediated loading method for siRNA into bovine milk exosome | (Shandilya et al., 2020) |
The therapeutic efficacy of Paclitaxel was significantly increased by loading Paclitaxel, an anticancer drug with low water solubility and strong toxicity, in bovine colostrum-derived exosomes. | (Kandimalla et al., 2021a) |
Oral administration and confirmation of uptake of bovine milk EVs loaded with locked nucleic acid antisense oligonucleotides. | (Grossen et al., 2021) |
Epicatechin gallate-loaded bovine milk exosomes have a neuroprotective role in rotenone-induced Parkinson’s disease. | (Luo et al., 2021) |
Developed efficient oral delivery platform of siRNA by coating hydrophilic polyethylene glycol (PEG) on the surface of bovine milk exosomes and loading siRNA with cationic chemical transfection. | (Warren et al., 2021) |
Evaluation of uptake in hepatic and intestinal cell lines of milk exosomes loaded with exogenous hsa-miR148a-3p. | (del Pozo-Acebo et al., 2021) |
Therapeutic Effects of Bovine Milk-Derived Extracellular Vesicles
Milk and milk-derived EVs are important for the development of the immune system of calves, and EVs express a large number of immune-related miRNAs and proteins and exert immunomodulatory functions. Matic et al. (2020) reported that bovine milk exosomes promoted the proliferation of RAW 264.7 cells, decreased cisplatin-induced cytotoxicity, and regulated the expression of proteins related to the cell cycle and proliferation. Additionally, a previous study reported that the pretreatment with milk EVs in LPS-treated RAW 264.7 cells alleviated the production of inflammatory cytokines and decreased NK-κB activity (Ascanius et al., 2021). According to Pieters et al. (2015), commercial cow milk contains exosomes that are stable under low pH and boiling-freezing conditions and can carry bioactive immunoregulatory TGF-β. In addition, cow milk exosomes alone could not regulate immune cells but enhanced the production of interferon-γ cells by NK cells and γδT cells in inflammatory conditions (Komine-Aizawa et al., 2020). A previous study examined whether agricultural dust-derived inflammatory responses were modulated by bovine milk-derived EVs in a mouse model. When mice were fed a diet containing milk EVs and then exposed to organic dust, the inflammatory response was improved, and M1 polarization of macrophages was promoted, suggesting that milk-derived exosomes promote immune responses in inflammatory conditions (Nordgren et al., 2019).
In addition to regulating the immune system, milk-derived EVs are absorbed in the intestine and exert beneficial effects on intestinal health. In particular, they not only affect intestinal epithelial cells and the microbiota but may also have a protective effect in diseases that occur in the intestine. When milk EVs were fed to mice for 8 weeks, expression levels of genes important for the integrity of the mucus layer, including Muc2, RegIIIγ, and Myd88, were increased (Tong et al., 2020). Additionally, when oxidative stress was induced in intestinal crypt epithelial cells pretreated with bovine exosomes, superoxide dismutase, glutathione peroxidase activity and heme-oxygenase 1 (HO-1) protein levels was increased. In addition, ROS levels and the activity of adenosine deaminase and xanthine oxidase were decreased, suggesting that milk EVs could enhance the energy state of damaged cells to exert a protective effect against oxidative stress (Wang et al., 2021a; Wang et al., 2021b). Maghraby et al. (2021) reported that malnutrition-associated intestinal dysfunction was significantly alleviated by milk EV-induced intestinal stem cell proliferation and villus architecture restoration. Necrotizing enterocolitis (NEC), a type of colitis that can occur in premature infants, is characterized by significant intestinal damage and decreased mucin production. Treatment of milk exosomes with experimental NEC pups can alleviate the symptoms of NEC by increasing the expression of goblet cells and regulating genes involved in protein synthesis (Li et al., 2019). In addition, previous studies reported that treatment using cow milk-derived EVs alleviated colitis in both a DSS-induced colitis model and a genetic mouse model of ulcerative colitis (Benmoussa et al., 2019; Reif et al., 2020; Stremmel et al., 2020; Tong et al., 2021).
Milk-derived EVs have been reported to exert a beneficial effect on intestinal health, which may be due to milk EVs bringing positive changes to the gut microbiota. Therefore, studies are being conducted to determine how milk EVs affect the intestinal microbiota. Yu et al. (2019) proposed that when exosomes isolated from milk were cocultured with bacteria, they promoted the growth of Escherichiacoli K-12 MG1655, a commensal bacterium, and Lactobacillus plantarum WCFS1, a probiotic, and influenced gene expression. Feeding C57BL/6 mice a diet containing milk exosomes and RNA was shown to affect the cecum microbiota composition in mice, a non-bovine species (Zhou et al., 2019). Similarly, when C57BL/6 mice were fed milk EVs containing 1.0×1010 particles per gram body weight, they had an effect on the gut microbiota and serum metabolites. In particular, among the intestinal microbiota, Akkermansia and Muribaculum, which are known to be beneficial, increased, and Desulfovibrio, which is known to be harmful, decreased. In addition, serum metabolite analysis revealed that milk EVs affected lipid and amino acid metabolism, especially anti-inflammatory factors such as lysophosphatidylcholines, deoxycholic acid, eicosapentaenoic acid, related to immune and metabolic diseases (Du et al., 2021). In addition to previous studies showing that milk EVs affect the intestinal microbiota and bacteria, studies have also investigated how these changes may affect intestinal immunity and colitis. When milk EVs were administered to mice, as in previous results, they had an effect on intestinal microbes and their metabolites, short-chain fatty acids, and the expression of genes related to intestinal immunity was increased (Tong et al., 2020). The same researchers reported that milk EVs alleviated ulcerative colitis by altering the gut microbiome and modulating gut immunity. Feeding milk EVs to an experimental mouse model of ulcerative colitis restricted the TLR4 signaling pathway and NLRP3 activation and restored DSS-induced intestinal dysbiosis (Tong et al., 2021).
Other Functions in Human Health
Previous studies have consistently reported that milk-derived EVs display beneficial biological activity in immune and intestinal health, as well as in various other conditions. Milk is a nutritionally rich source of calcium and protein that is known to support bone health (Pirilä et al., 2011; Uenishi et al., 2007). Several studies reported on the effect of EVs derived from milk on bone health. Milk EVs promote osteoblastogenesis, increase the number of osteocytes, and have been shown to increase bone mineral density (Oliveira et al., 2016; Oliveira et al., 2017; Oliveira et al., 2020; Yun et al., 2020). They exert beneficial effect on reproduction (Sadri et al., 2020), cardiac fibrosis (Zhang et al., 2021), arthritis (Arntz et al., 2015), cancer (Fonseka et al., 2021; Samuel et al., 2021), melanogenesis (Bae and Kim, 2021), and scar-free wound healing (Ahn et al., 2021). Milk EVs have been reported to exhibit biological effects in various case, but precise mechanistic studies and human trials are essential to define a strategy to leverage milk EVs as therapeutics.
Conclusion and Perspectives
Based on current studies, bovine milk-derived EVs are highly stable and absorbed in the body and are full of bioactive substances with multiple functions. Therefore, there are expectations for further therapeutic utilization. However, there are still goals that need to be achieved for success. Until now, due to the development of NGS and MS technology, the global investigation of cargo in milk EVs has been conducted in various ways. However, to administer it to the human body, it is necessary to analyze all components precisely whether there is any component to induce any adverse. To this end, it is necessary to develop more efficient technical methods to isolate EVs for clinical trial and a technology that can fully analyze their cargo. In particular, for the role of transporting drugs or nucleic acids for the treatment of diseases, a precise study on whether there is any problem in the long-term and high-dose application of milk EVs itself is required. In addition, it is necessary to investigate the detailed mechanisms of the biological effects revealed hitherto and to conduct further studies on whether therapeutic clinical application is possible.