Introduction
Meat-restructuring technology is a method that partially or completely disassembles and reforms meat in a different form (Sun, 2009). This technology can improve the quality characteristics (e.g., structure, texture, appearance, and nutritional value) of meat products and has been applied to low-quality cuts of meat to produce value-added meat products (Gadekar et al., 2014). Therefore, the manufacture of restructured meat products using low-quality meat cuts has economic advantages in the meat industry (Gurikar et al., 2014).
Semi-dried restructured sausages, one of the restructured meat products, have higher protein content and lower moisture content than regular sausages (Choi et al., 2016). However, the structural complexity of semi-dried restructured sausages, composed of meat and other functional ingredients, has been reported to reduce thermal conductivity and permeability during the drying process (Han et al., 2011). Therefore, the manufacture of semi-dried restructured sausages requires a long time and high energy consumption.
The drying process in semi-dried restructured sausage is an important procedure for determining quality and storage stability, and hot-air drying is a conventionally used in these products (Aykın Dinçer, 2021; Jin et al., 2017). During hot air drying, moisture in the internal muscle migrates to the surface of the product because of heat conduction from the air onto the surface of the meat, resulting in moisture evaporation (Shi et al., 2021b). Thus, increased drying time induces tough texture and off-flavor (Arnau et al., 2007), and deteriorates the quality of final products such as tenderness, color, flavor, and nutrients (Ran et al., 2019).
Moisture content is known to be highly associated with thermal diffusivity, mass transfer, and specific heat during the food drying process (Mariani et al., 2008; Modi et al., 2014; Razavi and Taghizadeh, 2007). In particular, the high initial moisture content of meat products attenuates the particle-particle interaction in materials, thereby resulting in increased porosity and shortened drying time (Deng et al., 2018; Jerwanska et al., 1995). A previous study reported that the high porosity of products improves heat and mass transfer during the drying process (Datta, 2007). In addition, the increase in porosity through the use of various processing methods, such as vacuum, freeze, microwave, and infrared radiation treatments, improved the drying characteristics and quality properties (Aykın Dinçer, 2021; Kumar and Karim, 2019; Riadh et al., 2015). Furthermore, the formation of a porous structure in dried meat products inhibits the shrinkage and tough texture of jerky (Kim et al., 2021a).
We hypothesized that increase of initial moisture contents could improve the drying rates and physicochemical properties of semi-dried restructured sausages by increasing porosity and moisture diffusivity. Therefore, this study aimed to investigate the effects of different initial moisture contents on the drying characteristics and physicochemical properties of semi-dried restructured sausages.
Materials and Methods
Fresh pork ham was purchased from a local market (Incheon, Korea), and the visible connective tissue from the meat was trimmed. The lean meat was ground using a meat grinder (MGB-32, Hankook Fujee Industries, Suwon, Korea) through a 3 mm plate. Six different formulations and the initial moisture contents of the semi-dried restructured sausages are listed in Table 1. Briefly, sausage samples were prepared using combinations of pork/water as follows: 100/0%, 90/10%, 80/20%, 70/30%, 60/40%, and 50/50% (w/w) with 1% salt based on the pork weight (w/w). Ground lean meat and salt were homogenized for 1 min in a mixer (K5SS, KitchenAid, St. Joseph, MI, USA) and then homogenized with ice. After homogenization, the meat batter was stuffed into a collagen casing (10 mm, COLFAN, Lodi, CA, USA) and the length of each sausage was set at 100 mm. All samples were frozen at −18°C until the core temperature of each sample reached −15°C, followed by removal of the casing.
The sausages were dried in a convection dry oven (HSC-150, AccuResearch Korea, Hanam, Korea). The constant air velocity in the dry oven was 0.5±0.1 m/s, being continuously measured over 3 min. All samples were dried equally at 85°C for different time periods (10, 20, 30, 40, 50, 60, 80, 100, 120, 150, 180, 240, 300, 360, and 580 min). The samples were removed from the oven at each time point, and the moisture contents were determined according to the official AOAC method (AOAC, 2000). The drying kinetics of semi-dried restructured sausage were illustrated using moisture content (g/g, dry basis), moisture ratio (g/g), drying rate (g/(g·h)), and effective moisture diffusivity (m2/s) (Xie et al., 2020). All treatment groups had six replicates and each group weighed approximately 4 kg.
The moisture content of semi-dried restructured sausage at any time was calculated using the following Equation (1).
where Wt (g water/ g dry basis) and Wds (g) are the weight of sausage at t time of drying and the final dry weight of sausage which can be calculated by initial weight and moisture content.
The moisture ratio (MR) during the drying experiments can be expressed by Equation (2).
where M0, Mt, and Me are the initial moisture content (g/g), moisture content at time t (g/g), and equilibrium moisture content during the drying process (g/g), respectively. Equation (2) can be simplified to Equation (3).
where, during long drying times, the value of Me is regarded as zero compared to Mt and M0 (Aykın-Dinçer and Erbaş, 2018).
The drying rate (DR) can be calculated from the mass of water removed from the material per unit of time in units of mass. This can be expressed using Equation (4).
where t1 and t2 are the specific drying times (min). Mt1 and Mt2, calculated using Equation (1), are the moisture contents at times t1 and t2 on a dry basis (g/g), respectively.
The migration of moisture during the drying process is controlled by diffusion. According to Fick’s second law of diffusion, effective moisture diffusivity (m2/s) can be calculated when the moisture content of the semi-dried restructured sausage was reduced below 0.5 g/g (dry basis) using the following Equation (5).
Using an infinite slab geometry and consistent initial moisture distribution, Equation (5) can be calculated according to Equation (6) (Aykın-Dinçer and Erbaş, 2018).
where n is the number of experiments, t the drying time (s), and L the half thickness of the sausage (m). Equation (6) can be simplified to Equation (7) by taking the natural logarithms.
The effective moisture diffusivity was calculated by plotting the drying data at a given drying time using the slope of the graph acquired from ln(MR). This can be expressed by Equation (8).
Sausages with different water contents (0%, 10%, 20%, 30%, 40%, and 50%) were prepared under the same condition of below 0.5 g/g moisture content on a dry basis. Samples were dried at 85°C for 360, 350, 350, 300, 270, and 270 min using a convection dry oven (HSC-150). The moisture content of each sample was determined using the AOAC method (2000). The physicochemical characteristics of the sausages were determined by measuring the water activity, pH, color, porosity, volatile basic nitrogen, shear force, and microstructure.
A water activity meter (Humimeter RH2, Schaller, Austria) was used to measure the water activity of semi-dried restructured sausages at a temperature of 25±1°C.
Semi-dried restructured sausages (5 g) and distilled water (20 mL) were homogenized using a homogenizer (Daihan Scientific, Gangwon, Korea) for 2 min at 10,000 rpm. The pH of the homogenate was determined using a LAQUA pH meter (Horiba, Kyoto, Japan).
The color of the semi-dried restructured sausages was determined using a colorimeter (CR-210, Minolta Camera, Osaka, Japan). It is expressed using L* (lightness), a* (redness), and b* (yellowness) color values according to the CIE (International Commission on Illumination). The colorimeter was calibrated using a white plate (L*=97.27, a*=5.21, and b*=−3.40).
For the calculation of porosity (ε, %), the apparent density (ρa, g/cm) and real density (ρr, g/cm) were required (Silva-Espinoza et al., 2019). The apparent density refers to the weight (m, g) per volume (V, cm3) of the material, including water and pores. Real density also refers to the weight per volume but does not consider the pores in the material. The apparent density can be expressed by Equation (9).
where the real density is calculated based on the composition of the sample according to Equation (10).
where XW is the mass fraction of water, ρW the density of water (0.9976 g/cm3), XCH the mass fraction of carbohydrates, and ρCH the density of carbohydrates (1.4246 g/cm3). The porosity was calculated using Equation (11).
The shear force of the semi-dried restructured sausage was obtained using a texture analyzer (TA-XT2i, Stable Micro Systems, Surry, UK) with a V-slot blade (Warner-Bratzler, Hamilton, MA, USA). The texture analyzer was operated at pre-test speed 2.0 mm/s, test speed 2.0 mm/s and post-test speed 1.0 mm/s at 25±1°C. The shear force was expressed in kilograms (kg).
Volatile basic nitrogen (VBN) was measured using microdiffusion analysis as previously described (Kim et al., 2019). Semi-dried restructured sausage (5 g) was homogenized with distilled water (20 mL) for 1 min at 12,000 rpm. The homogenates were mixed with distilled water (30 mL) and filtered through Whatman No.1 filter paper. The indicator solution was prepared by mixing 0.066% methyl red in ethanol and 0.066% bromocresol green in ethanol at a 1:1 ratio. In the inner part of the Conway diffusion cell, the indicator solution (100 μL) and 0.01N H3BO3 (1 mL) were added. The filtrate of the sample (1 mL) and 50% K2CO3 solution (1 mL) were added to the outer section of the cell. The cells were left at 37°C for 90 min and the 0.02N H2SO4 was titrated to the solution of inner part. The VBN value is expressed as mg%.
The microstructures of the semi-dried restructured sausages were evaluated using field emission scanning electron microscopy (FE-SEM) (SU8010, Hitachi, Tokyo, Japan). The samples were cut into small pieces and frozen for 12 h at −78°C. The frozen samples were lyophilized in a vacuum evaporator (MCFD 8508, Il Shin Bio, Yangju, Korea) for 24 h. Thereafter, the samples were sputter-coated with platinum using an ion sputter (MC1000, Hitachi) and observed at an accelerating voltage of 5 kV by FE-SEM. The magnification of all images was ×300.
Experimental data were analyzed using SPSS Statistics 24 software (IBM, Armonk, NY, USA). Data are presented as mean±SD, and one-way analysis of variance with Duncan’s multiple range test was performed to identify significant differences (p<0.05). Data were obtained from at least three replicates in all experiments.
Results and Discussion
The results of moisture content, moisture ratio, and drying rate are shown in Fig. 1. The moisture content and moisture ratio of all samples gradually decreased during the drying period (Fig. 1A and 1B). In addition, the moisture ratio of sausages with water addition (WAL10−50) was lower than that of the sausages without water addition (WAL0 group). The drying rate was also higher in sausages with added water during the initial 10 min of drying (Fig. 1C). Free water evaporates more easily than bound water in food products (Tunde-Akintunde et al., 2005). A previous study reported that a higher moisture content of ginger slices resulted in a higher drying rate during the preliminary stage of drying (Zeng et al., 2022). Thus, the increase of drying rate resulted from the increase of free water in the sausage. Moreover, the drying time required to reach a moisture content of 50% (dry basis) in sausages was shortened by the increase in the water addition level (Table 2). Shortening the drying time during the production of dried foods is important for minimizing energy consumption and improving quality properties (Riadh et al., 2015; Tunde-Akintunde and Ogunlakin, 2011). Our data indicate that an increase of initial moisture content significantly shortened the drying time and improved the drying characteristics of the sausages. A similar observation was reported, in which high initial moisture content using brine injection to beef jerky shortened the drying time (Kim et al., 2022). Collectively, our data demonstrate that an increase in the initial water content can accelerate the drying rate and shorten the drying time in semi-dried restructured sausages.
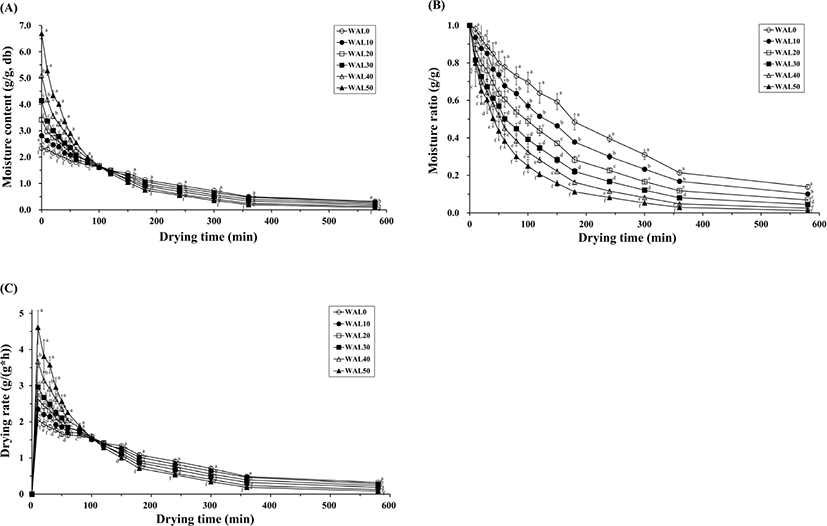
Deff represents the intrinsic mass transfer in food materials and has been used to provide information about moisture movement within foods during the drying process (Dadali et al., 2007). Deff values were calculated at the time of drying, and the moisture content of the sausages reached 50% (dry basis). The Deff value of the sausage increased with the amount of water added (p<0.05) (Table 2). Deff is influenced by various factors, such as drying temperature, pretreatment, and ingredients added to food materials (Sharma and Prasad, 2004; Tunde-Akintunde and Ogunlakin, 2011; Wang et al., 2019). In several previous studies, high moisture content was closely related to an increase in the Deff value (Chen et al., 2020; Wang et al., 2019). The Deff value is influenced by the thermal conductivity of water, which has a higher thermal conductivity than proteins or fats (Tavman and Tavman, 1999). Additionally, the pore network, which is affected by the higher water content, also influences the Deff value of foods (Chen, 2007). In a previous study, the porous structure of pumpkin slices was induced by blanching and freezing before hot air drying, which resulted in a higher moisture diffusivity during drying (Ando et al., 2019). Collectively, an increase of initial moisture content positively influenced the high thermal diffusivity in semi-dried restructured sausages, which consequently led to an increased drying rate and effective moisture diffusivity.
The pH values of the semi-dried restructured sausages are listed in Table 3. Overall, there were no significant differences between the groups, although an increase of initial moisture content may be associated with higher pH values. This may result from the shorter drying time in the groups with added water. According to previous studies, a relatively short drying time inhibited the lowering of pH in meat products by reducing protein denaturation during the drying process (Yang et al., 2009).
Color of semi-dried restructured sausages are presented as the L*, a*, and b* values in Table 3. The significant increase of L* and b* values were observed in the WAL40 and WAL50 group than WAL0 group, and increment of water addition levels induced the decrease of a* values (p<0.05). The increase in the L* values of sausages with the addition of water may result from the shortened drying time. During the drying process, Maillard browning reactions occur, which are associated with the darker color of the products (Akonor et al., 2016). A previous study showed that increased drying time induced lower L* and b* values in restructured jerky (Kim et al., 2021b). Taken together, our results show that the shortened drying time owing to the addition of water to the semi-dried restructured sausage blocked the excessive browning reactions.
The water activity of the semi-dried restructured sausages gradually decreased depending on the drying time (Fig. 2). As the amount of added water increased, the water activity of the sausages decreased markedly. Previous studies have reported that thermal conductivity increased with an increase in the moisture content of foods (Modi et al., 2014). In addition, our data show that the addition of water increased the drying rate (Fig. 1). Thus, the decrease in water activity with the addition of water to the sausage resulted from the increase in thermal conductivity, which accelerates the decrease in free water. Moreover, water activity is one of the most crucial factors related to the classification criteria for semi-dried restructured sausages (Jang et al., 2015), and the safety of microorganisms in semi-dried foods. Water activity between 0.60−0.90 is desirable for intermediate moisture foods (Aguilera and Gutiérrez-López, 2018). In our study, with water addition levels of 0%, 10%, 20%, 30%, 40%, and 50% of sausages, the time required for the water activities of sausages to reach below 0.90 were 300, 240, 180, 180, 180, and 180 min and the time to reach water activities below 0.80 were 580, 360, 360, 360, 300, and 300 min, respectively. Our data indicate that the addition of water to semi-dried restructured sausages positively influences microbiological safety by decreasing water activity.
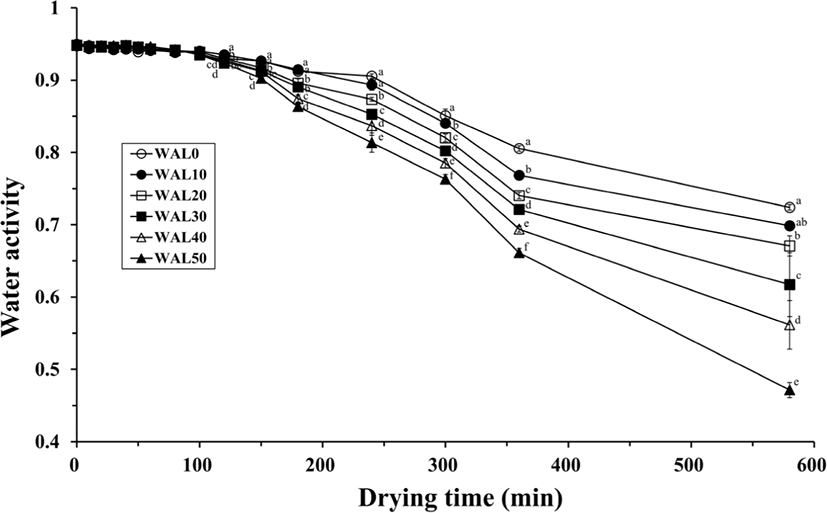
The porosity of semi-dried restructured sausages was significantly affected by the water addition levels in the samples (p<0.05) (Table 4), and the WAL50 group had the highest porosity and WAL20, WAL30, and WAL40 groups had significantly higher porosity than WAL0 group (p<0.05). This result could be explained by the increased number of water molecules, which might induce the formation of porous structures in the samples during the drying process (Kim et al., 2022). Different water contents in food materials affect the size and/or number of pores (Wang and Liapis, 2012). In addition, the high moisture content and porous structure of food materials shorten the drying time, as they accelerate heat and mass transfer during the drying process (Kim et al., 2022). Therefore, the high porosity from the increased amount of added water in the samples could explain the drying rate of the semi-dried restructured sausages.
An extended drying time causes excessive hardening in meat products, and the shear force is associated with the tenderness of meat products (Shi et al., 2021a). In the present study, the shear force of the sausages was significantly affected by the moisture content of the samples (p<0.05) (Table 4). The shear force of the sausages significantly decreased with increasing initial water content, except WAL10 group (p<0.05). These results can be explained by the reduced drying time of samples with added water. During drying, myofibrillar protein is denatured by heat and extended drying time, which causes shrinkage of myofibrillar protein (Shi et al., 2021a). This shrinkage induces tough texture of dried meat products, and the excessive hardness of dried meat negatively influences consumer preference (Kim et al., 2010). Collectively, our data suggest that increased moisture content leads to lower shear forces in semi-dried restructured sausages resulting from the increased formation of porous structures during the drying process.
The VBN values of the semi-dried restructured sausages are shown in Fig. 3. The increment of water addition levels in the sausages decreased the VBN values (p<0.05), although WAL10 group did not showed significant difference to WAL0 group (p>0.05). In the case of dried food, VBN values increase as the drying progresses because of the generation of volatile nitrogen compounds (Chen et al., 2004). Previous study reported that extended drying time significantly increases VBN values of dried yellow corvina (Gwak and Eun, 2010). Thus, the lower VBN values observed in the groups with more than 20% of moisture addition levels might result from the shortened drying time.
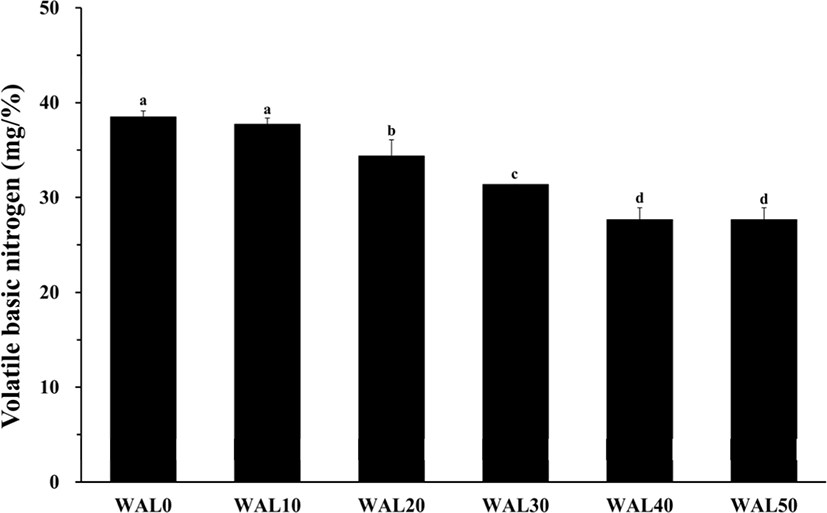
The microstructures of the semi-dried restructured sausages with different initial moisture contents were observed using FE-SEM (Fig. 4). The addition of water to the sausages formed pores, and the size and number of pores were markedly changed with the increase in initial moisture content. In WAL0 to WAL20 group, increase of water addition levels in semi-dried restructured sausages induced the increased number of small pores. In WAL30 to WAL50 group, the addition of water expanded the size of pores. During the drying process of meat products, heat treatment induces denaturation of myosin and decreases the water-binding capacity through the migration of water from the interior to the surface, resulting in the shrinkage of meat (Shi et al., 2021a). In this progress, water molecules were evaporated and formed a number of pores. The larger pore size was influenced by the increased rate of moisture migration (Labuza and Hyman, 1998). As observed in our data, the addition of water positively affected the porosity and pore size of the semi-dried restructured sausage by shortening the drying time. Indeed, the acceleration of moisture loss induces a higher number and size of pores in the semi-dried restructured jerky during the drying process (Kim et al., 2021b). Collectively, our data suggest that increasing the initial moisture content leads to a more porous structure, which improves the drying characteristics and physicochemical properties of semi-dried restructured sausages.
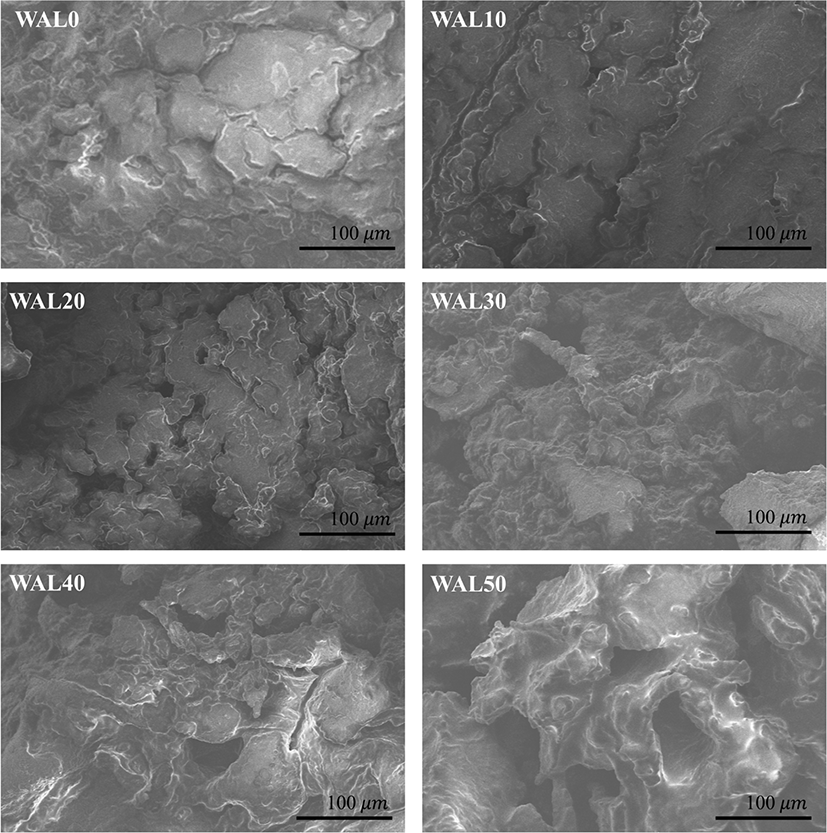
Conclusion
Our results demonstrated that increased initial moisture content in semi-dried restructured sausages shortened the drying time and increased thermal diffusivity. Particularly, WAL20, WAL30, WAL40, and WAL50 showed significantly improved physicochemical properties, including porosity, shear force, and VBN, compared with those of WAL0 (p<0.05). The structural difference in semi-dried restructured sausages was observed where the increment of initial moisture content resulted in improved drying characteristics. In conclusion, our data suggest that an increase in initial moisture content, especially more than 20% (w/w), is a good method for improving the drying characteristics and physicochemical properties of semi-dried restructured sausages.