Introduction
Recently, due to its high nutritional value and flavor, duck meat consumption has increased (Arias-Sosa and Rojas, 2021; Biswas et al., 2019). Simultaneously, as the duck meat industry grows, the production of blood generated during slaughter is expected to increase (Cho et al., 2020; Kim et al., 2020a). Due to its high solid matter content and chemical oxygen demand, the disposal of blood can cause environmental problems (Ofori and Hsieh, 2014). Treating blood in an environmentally friendly manner can be expensive (Liu, 2002). Therefore, methods to prevent environmental issues caused by blood are being considered (Adhikari et al., 2018; Wang et al., 2018).
Rich protein (18 g/100 g) and iron (30 mg/100 g) contents of duck blood make it an advantageous source of nutrients (Silva and Silvestre, 2003). The essential amino acid content of duck blood meets the amino acid requirements of adults as recommended by WHO et al. (2007), except isoleucine and methionine. Heme iron is contained in the blood and has a relatively high bioavailability compared to non-heme iron. Dried duck blood contains 833.05 μg/g of heme iron, which is higher than that contained in dried blood of pigs and chickens (Sorapukdee and Narunatsopanon, 2017). Blood from pigs, cows, and chickens have been studied as food additives or as sources of protein and iron (Ofori and Hsieh, 2011; Zou et al., 2019). Nevertheless, few studies have evaluated duck blood as a source of nutrients.
Blood contains high-quality nutrients, so there is a risk of perishability due to contact with surface organisms on the carcass and excreta during blood collection (Ofori and Hsieh, 2014). The drying process of blood can eliminate disadvantages such as spoilage (Hou et al., 2019; Wang et al., 2018). In addition, dry form of blood powder has many commercial merits, such as low storage costs, enhancement of storage and transportation, and the ease of mixing with other materials (Jin and Choi, 2021; Niu et al., 2019). However, depending on the drying conditions and methods, the biochemical characteristics and functional properties of the product may vary (Lewicki, 2006).
Drying methods such as spray drying (SD), freeze drying (FD), vacuum drying (VD), and hot air drying (HD) are used in the food industry. Each drying method has their own characteristics, depending on the drying efficiency, processing cost, and environmental influence (Hou et al., 2019; Liu et al., 2020). SD has the advantage of continuous and fast drying at a low cost. FD maintains a low temperature throughout the drying process, which is suitable for drying thermally sensitive ingredients (Niu et al., 2019). VD can reduce protein denaturation due to contact with oxygen and has been investigated as a method to overcome some of the disadvantages of FD (Parikh, 2015). HD has the advantage of easy process control and low processing cost (Liu et al., 2020). During drying, dehydration or high temperatures can cause protein denaturation, which can affect processing properties (Schmidt et al., 2019). Information on the effects of various drying methods on the quality of duck blood is still limited.
Therefore, the objective of this study was to investigate the effects of SD, FD, VD, and HD on the physicochemical and functional properties of duck blood gel. The results of this experiment can be used as a reference with basic data for product development using duck blood gel.
Materials and Methods
Duck blood contained 1% of trisodium citrate was obtained from slaughterhouse (Jangheung, Korea). Inlet temperature and feeding speed of a spray dryer (B-290, Buchi, Flawil, Switzerland) were as followed; 120°C and 125 mL/h. Lyophilization was conducted at –60°C of cold trap temperature, –70°C of material temperature, and 5 mTorr of vacuum for 72 h by freeze-drier (VTFD, Ilshin, Siheung, Korea). Duck blood also dried at 40°C for 48 h under vacuum condition (100 kPa) by vacuum drier (DRV622DA, Advantec, Saijo, Japan) and hot-air dried treatment was after drying at 40°C for 72 h by hot-air drier (HK-DO1000F, HANKUK S&I, Hwaseong, Korea).
The heat-induced duck blood gel was prepared according to the method explained by Kim et al. (2020b) with some modifications. Protein concentrations in the fresh duck blood and duck blood powder solutions were measured using BCA assay. Duck blood powder solutions (protein concentration 30 mg/mL) were transferred into 50 mL conical tubes. The samples were heated with a water bath at 90°C for 30 min, and cooled off overnight at 4°C.
Carbonyl contents was measured to estimate protein oxidation of dried duck blood (Estévez, 2011). Dinitrophenylhydrazine was used to react duck blood protein and the absorbance at 370 nm was read with Optizen 2120 UV plus (Mecasys, Daejeon, Korea). The protein content was determined using a BCA assay (Thermo Fisher Scientific, Waltham, MA, USA) standardized by bovine serum albumin. The absorbance was measured to calculate protein contents at 562 nm. The carbonyl content was showed as nmol carbonyls mg−1 of protein (molar absorptivity of 21.0 nM−1 cm−1).
Lipid oxidation was examined using the TBARS method (Tarladgis et al., 1960). The raw sample (10 g) was mixed with distilled water (50 mL) and 50 μL of BHT and homogenized at 10,000 rpm for 1 min. After adding 47.5 mL of distilled water, the homogenate was transferred to a distillation flask with 2.5 mL of 4 N HCl and an antifoam agent. Then, 5 mL of distillate and 0.02 M 2-thiobarbituric acid (5 mL) reacted at 100°C for 35 min, and cooled at room temperature (25°C) for 10 min. The absorbance was read at 538 nm.
The fluorescence intensity of tryptophan was examined using the method of Wang et al. (2019). The fluorescence spectrum of the duck blood sample was dissolved in distilled water to obtain a protein concentration of 0.5 mg/mL. The sample was measured between 300 and 400 nm, at an excitation wavelength of 280 nm using a plate reader (Varioskan LUX, Thermo Fisher Scientific).
The thermal stability of duck blood was determined with DSC (DSC 4000, PerkinElmer, Waltham, MA, USA). A sealed aluminum pan (containing 30 mg sample, 30 mg/mL of protein concentration) was placed on one side and empty pan (used as a reference) was placed on the other side to detect caloric changes. The temperature range was set at 20°C–100°C with heating of 10°C per min. The peak and enthalpy (ΔH) were analyzed using the Pyris data analysis software (PerkinElmer).
The pH values of duck blood samples were measured by the method described by Kim et al. (2020c). The pH values of a homogenized set with 5 g of raw and cooked samples and distilled water (20 mL) were determined using a pH meter (Model 360, Mettler-Toledo GmbH, Schwerzenbach, Switzerland).
The color of the heat-induced gel sample was determined using a colorimeter (CR-410, Konica Minolta, Osaka, Japan; Illuminant C). The colorimeter parameters were calibrated with a white plate (L*=+97.83, a*=−0.43, b*=+1.98). L*, a*, and b* values were measured. The color difference (ΔE) was measured using the following formula:
To measure the cooking loss of heat-induced duck blood gel, the samples were weighted twice before heating and after cooling overnight at 4°C. Cooking loss was calculated as follows:
Press method was used to estimate WHC (Grau and Hamm, 1953). The 0.3 g of the sample was placed onto a Whatman No. 2 filter paper and pressed with two plexiglass plates for 3 min. The areas of pressed water and sample were measured using a planimeter, and WHC was obtained according to the following formula;
Hardness (kg), springiness, cohesiveness, gumminess (kg), and chewiness (kg) were examined in this study. The heat-induced gel samples were cut to a height of 10 mm and used for measurements. Texture analyzer (TA-XT2i, Stable Micro Systems, Godalming, UK) conditions were regulated as follows: Maximum load, 2 kg; pre-test speed, 2.0 mm/s; post-test speed, 5.0 mm/s; force, 5 g; strain, 50%; and test speed, 2.0 mm/s.
The microstructure of the heat-induced duck blood gels was investigated using scanning electron microscopy (SEM). The heat-induced gel samples were freeze-dried and sputter coated by the method of Pankyamma et al. (2019). Samples were coated with a vacuum ion coater (KIC-IA, Coxem, Daejeon, Korea; Widyastuti et al., 2020). Each of the specimens were observed in a SEM (EM-30SX, Coxem, Dajeon, Korea) at a voltage of 15 kV.
The data of this study were acquired from triplicate experiments and analyzed using the general linear model. The least square means and SE of the least square means was presented and the significance of the main effect was tested by Tukey’s multiple tests (p<0.05). Statistical analysis was performed using SPSS Statistics 20.0 (SPSS, Chicago, IL, USA).
Results and Discussion
Fig. 1 shows the effect of various drying methods on the carbonyl and malondialdehyde (MDA) contents of duck blood. The carbonyl content of protein is a commonly used metric for estimating oxidative stress-mediated protein oxidation (Nyaisaba et al., 2019). The highest carbonyl content was observed in HD, followed by SD and VD. The lowest carbonyl content was observed in FD (p<0.05). The results showed that the heating process had a significant effect on the increase in the carbonyl content, consistent with the results of previous studies which found that heating of proteins can increase carbonyl content (Lu et al., 2017). Duck blood contains abundant metal ions (Sorapukdee and Narunatsopanon, 2017). When oxygen and electron donors are present, the oxygen free radical generation system may catalyze oxidative modification of the protein (Stadtman, 1990). Contact with oxygen can create highly oxidative conditions for proteins during the drying process, resulting in an increase in the carbonyl content (Traore et al., 2012). This may cause an increase in the carbonyl content of HD, suggesting that vacuum conditions in VD and FD treatment inhibited an increase in the carbonyl content.
MDA is an oxidizing substance of polyunsaturated fatty acids and can be used as an index to evaluate lipid oxidation (Wood et al., 2008). SD had the lowest levels of MDA, followed by FD, VD, and HD (p<0.05). Factors that significantly influence the increase in TBARS during drying are drying time and temperature (Fu et al., 2015). During drying, with the evaporation of moisture, the fat exudates to the surface, which can enhance the contact of fat with oxygen and promote lipid oxidation. FD is carried out by freezing, a primary drying, and then a secondary drying. The material frozen for drying contains approximately 15%–20% unfrozen water, and the temperature rises to dry the unfrozen water during the secondary drying process. This process can adversely affect product stability (Patel et al., 2010). SD is expected to be relatively less affected by lipid oxidation due to a short drying time.
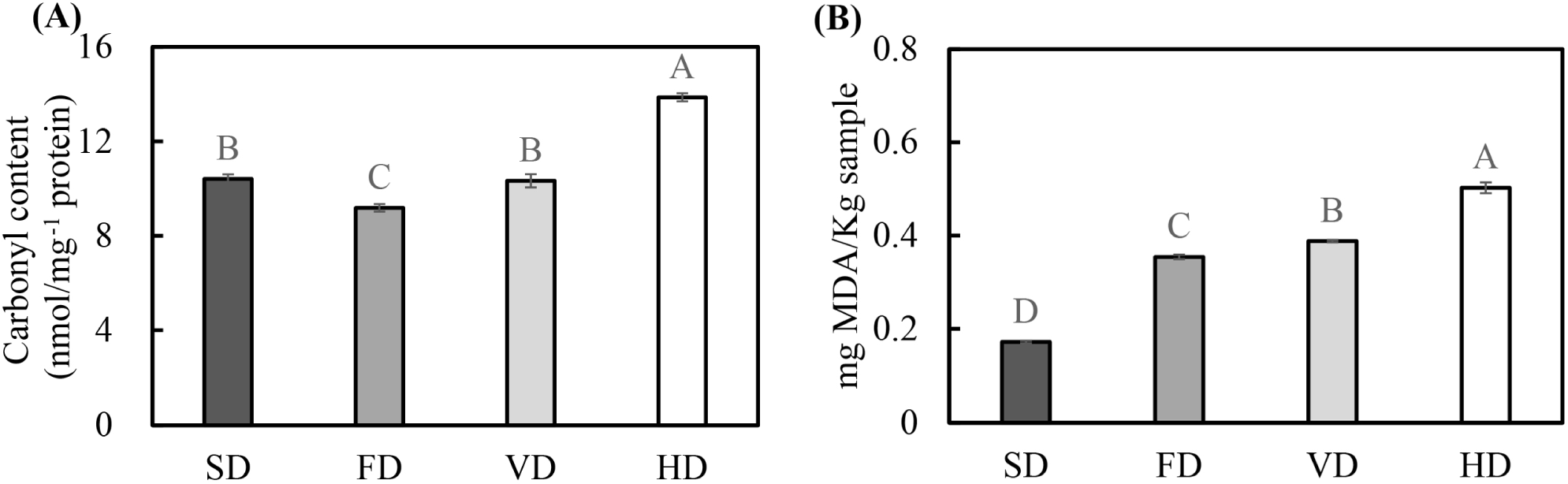
The effects of various drying methods on the endogenous tryptophan fluorescence intensity of duck blood are shown in Fig. 2. Endogenous tryptophan fluorescence intensity analysis was conducted to measure the effect of various drying methods on the tertiary structure of duck blood proteins. Since hydrophobic interactions constitute a protein tertiary structure, and amino acids such as tryptophan are usually buried in the protein core, changes in the tertiary structure can be confirmed through analysis of the internal tryptophan fluorescence intensity (Lee et al., 2021). The tryptophan fluorescence intensity in all treatments showed λmax fluorescence emission at 328 nm, indicating that the microenvironment of tryptophan residues remained unchanged. The intensity was the lowest in HD, with a peak wavelength of 328 nm, followed by VD (p<0.05), and there was no significant difference between FD and SD (p>0.05). These results indicated that the prolonged heat-drying process affected protein exposure to tryptophan residues and were consistent with the results of a previous study using gelatin (Feng et al., 2021). Another prior study noted that the fluorescence intensity of silver carp (Hypophthalmichthys molitrix) myofibrillar protein increased significantly after FD and vacuum SD (Niu et al., 2019). Exposure of amino acids with fluorescent properties, such as tyrosine and tryptophan, can result in an increase in fluorescence intensity (Jiang et al., 2017).
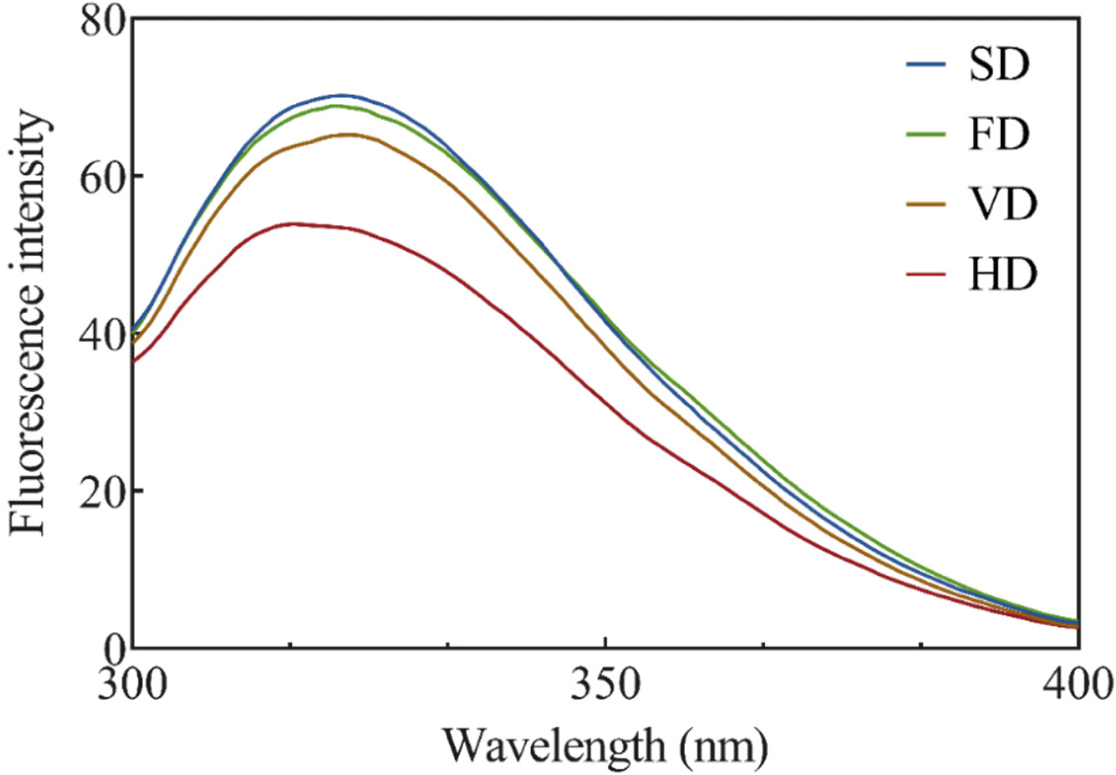
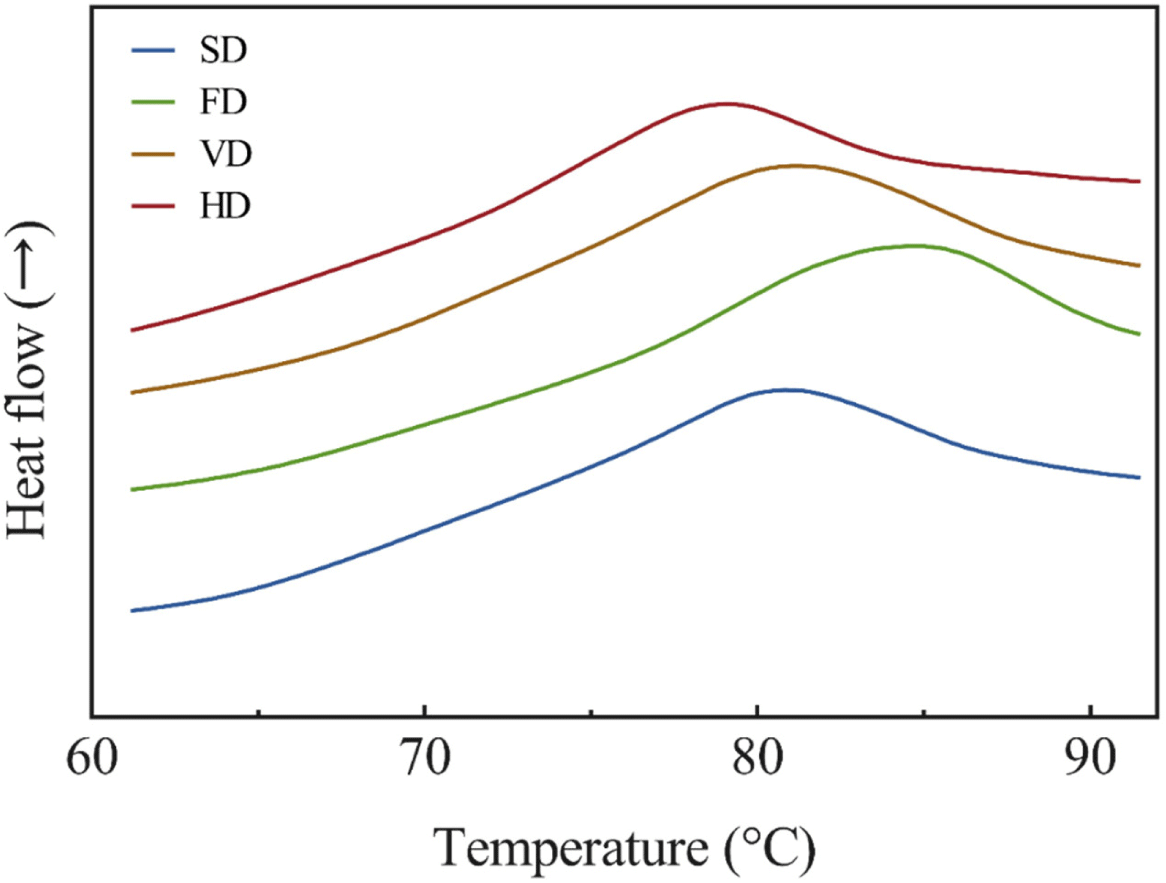
Our results of the thermal stability of the duck blood powder solutions are presented in Fig. 3. DSC is used to measure the properties of a protein, because the peak temperature and change in enthalpy may change depending on its stability, composition, and structure (Kim et al., 2020b). The peak denaturation temperature of a protein represents its thermal stability (Ghribi et al., 2015), and was significantly higher in FD and significantly lower in HD (p<0.05). From these results, it can be assumed that the thermal stability of FD is the highest and that of HD is the lowest. Oxidation of proteins can cause structural changes (Bischof and He, 2006), and thermal stability is associated with changes in protein structure (Hou et al., 2019). This result displayed the thermal stability according to the carbonyl content. The whole blood of ducks contains approximately 11.75 g/dL of hemoglobin, which is more than twice the plasma protein (Sorapukdee and Narunatsopanon, 2017). The peak is assumed to be due to the denaturation of hemoglobin (Cho and Choy, 1980). The peak of plasma protein is known to be 74°C–78°C, and it is presumed that the plasma proteins had been denatured by drying (Hou et al., 2019). It is assumed that the duck blood protein was denatured by the heating process, and it was consistent with the results of previous studies that measured the thermal stability of the myofibrillar protein by drying the golden pompano (Zhang et al., 2020). In addition, VD was shown to inhibit proteolysis more compared to HD, which was consistent with the results of Zhang et al. (2020).
Table 1 shows the effects of various drying methods on the pH and color of duck blood gel. Both raw and cooked samples showed high pH values in FD and SD, and the lowest value was observed in HD. The pH of the cooked treatments was significantly lower than that of the raw treatments (p<0.05). Despite not being indicated in the table, the pH of fresh blood was 8.05, which was considered to be due to the effect of trisodium citrate, which was added as an anticoagulant. There are abundant iron ions in the blood, which exist as Fe2+. This can promote autoxidation of O2 in the blood and lead to a decrease in pH (Gabay and Ginsburg, 1993). In addition, when blood is collected, it is converted to a Fe3+ due to binding with oxygen (Aycicek et al., 2014). It is presumed that pH changes due to contact with oxygen occurred during this drying and heating process.
Color is a visual appearance characteristic and is used as an indicator of quality by consumers (Font-i-Furnols and Guerrero, 2014). No significant difference in chromaticity was found in both raw and cooked samples (p>0.05). When comparing HD to other treatments, the color difference (ΔE) was less than 1. The ΔE of 1.0 is the smallest difference in color that the human eye can notice. If the ΔE is less than 1.0, it is not visually detected (Kuehni and Marcus, 1979). It is therefore assumed that the color change due to the difference in drying method cannot be distinguished by the consumer.
The results for cooking loss, WHC, and TPA of duck blood gel are shown in Table 2. Cooking loss and WHC can be used to determine the quality of food. Cooking loss of FD was the lowest, followed by HD, SD, and VD. WHC of FD and HD was the highest, and the other treatments followed (p<0.05). Among the heating methods, SD and VD samples had a low WHC, but HD had high values. This was consistent with the results of previous studies using separated peanut protein (Gong et al., 2016). However, the results were different from previous studies using pig plasma proteins (Hou et al., 2019). This is expected to vary due to differences in protein types and drying parameters. In addition, proper heating processes can cause particle size, surface hydrophobicity, and protein crosslinking, and it is speculated that they are mainly affected by interactions between protein subunits through hydrophobic, hydrogen, and disulfide bonds (Liu et al., 2015).
TPA is an indicator of gel quality. It showed the highest hardness in FD and HD, and the lowest value in VD. Springiness showed no significant differences among the treatments (p>0.05), and the lowest cohesiveness was observed in HD. In addition, the highest gumminess and chewiness was observed in FD. Proteins in the blood that are denatured during the drying process may affect aggregation during gelation (Parés et al., 1998). Denaturation of the protein tertiary structure by an appropriate heating process may have increased the hardness of HD (Samsalee and Sothornvit, 2014). Referring to the microstructure in Fig. 3, more moisture may have been eluted from SD and VD with larger pore sizes.
The microstructure of heat-induced duck blood gels of different drying methods is shown in Fig. 4. The gel microstructure of the FD sample displayed a relatively dense structure. The gel structure of the HD sample was irregular, but it displayed a more aggregated structure compared to the SD and VD samples. In the SD and VD samples, small pores and somewhat larger pores were observed together. This may have affected cooking loss and WHC. Irregular gel network structures affect the mobility of moisture and the binding strength of the gel. The number and size of pores allow us to assume the movement of water molecules within the gel network structure (Han et al., 2014). The denaturation of the protein structure by drying can cause exposure of amino acid residues in the protein (Feng et al., 2021), which is thought to affect the gel structure.
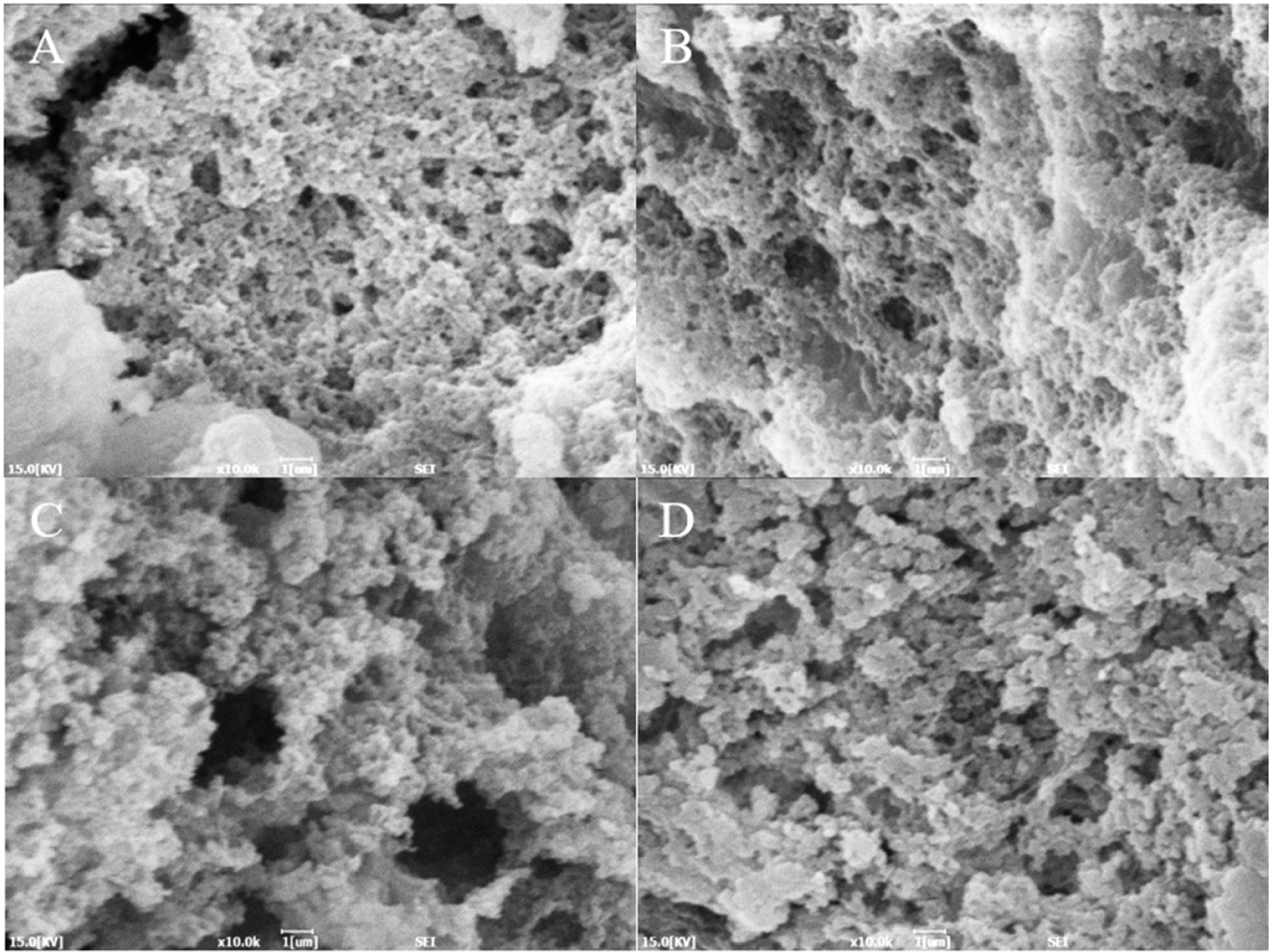
Conclusion
The effects of various drying methods on the biochemical properties and functional quality of duck blood were investigated in this study. The FD sample displayed a low protein oxidation and high thermal stability. In addition, FD displayed good quality in terms of WHC, cooking loss, and TPA. Among the heating methods, HD displayed high values for the functional properties, while SD displayed low protein oxidation and lipid oxidation. The degree of protein denaturation was influenced by the heating method, and rapid, low-temperature drying minimized denaturation. The results of this experiment show that freeze-drying, which is a non-heating method, is suitable for effectively drying duck blood. Among the heat drying methods, SD showed high physicochemical stability. Future research should focus on improving the physicochemical quality of blood powders in the food industry.