Introduction
Nowadays, the use of natural ingredients is required in the food industry, because consumers are demanding “clean label” products and they prefer natural food ingredients. Many food manufactures are attempting to replace synthetic emulsifiers and stabilizers with natural ingredients to produce “clean label” products (Lam and Nickerson, 2013; Ozturk and McClements, 2016). Proteins and polysaccharides are considered good alternatives for the stabilization of emulsion foods (Bouyer et al., 2012; Setiowati et al., 2017). Proteins can effectively form small oil droplets even at low concentrations, but they are sensitive to environmental conditions such as pH and ionic strength. In contrast, polysaccharides can form extremely stable emulsions even in environments with varying pH, ionic strength, and temperature. However, a high concentration of polysaccharides is required for emulsion formation because of their low surface activity (McClements and Gumus, 2016; McClements et al., 2017). To overcome these individual limitations of proteins and polysaccharides, combining the advantages of proteins (high surface activity) and polysaccharides (high stability) may serve as a valuable strategy. There exist two classes of protein/polysaccharide complexes: Electrostatic complexes (EC) and Maillard conjugates (MC) (Bouyer et al., 2012).
Protein/polysaccharide EC are non-covalent bonds formed due to attractive electrostatic interaction between anionic polysaccharides and positively charged proteins (Evans et al., 2013). EC can improve the heat stability of emulsions through the formation of a homogenous biopolymer layer on the surface of oil droplets. Nevertheless, these complexes are sensitive to environmental conditions because the protein–polysaccharide interaction is influenced by solvent conditions such as pH and ionic strength (Lam and Nickerson, 2013; Sedaghat Doost et al., 2019). In contrast, MC are covalent bonds that can occur spontaneously during the early stages of the Maillard reaction (Nooshkam and Varidi, 2020). The polysaccharide moieties of MC provide greater layer thickness on the droplet surfaces, which can improve stability owing to the strong steric repulsion between droplets. In addition, the thickness and steric stabilization effects of MC-stabilized droplets can offset the electrostatic screening effects, which can be stable at high salt concentrations and under acidic pH (Akhtar and Ding, 2017; O’Mahony et al., 2017).
Whipping cream is a representative oil-in-water emulsion food containing 30%–40% milk fat, and κ-carrageenan (κ-Car) is widely used in dairy products to improve their physical and textural properties because of its ability to interact with casein micelles (Olivares et al., 2010; Seo et al., 2018). However, the addition of κ-Car to cream-based products with high levels of milk fat can lead to depletion-flocculation, eventually resulting in creaming destabilization (Seo and Yoo, 2021a). In a previous study, milk protein isolate (MPI) was sufficiently conjugated with κ-Car through the Maillard reaction at 65°C for 24 h in a wet-heating system, and the stability of the oil-in-water emulsions stabilized using the MPI/κ-Car conjugates was effectively improved because of the steric hindrance effects caused by the absorption of the MPI/κ-Car conjugates onto the fat droplet surfaces (Seo and Yoo, 2021b). In the present study, we investigate the effects of MPI/κ-Car MC as a natural emulsifier in whipping creams and compare them with MPI/κ-Car EC.
Materials and Methods
To prepare the MPI/κ-Car MC and EC, MPI (MPI 1900; protein 85% w/w) was obtained from Vitalus Nutrition (Abbotsford, Canada), and κ-Car was purchased from Sigma-Aldrich Chemical (MO, USA). Fresh cream containing 38% (w/w) milk fat was supplied by Seoul Dairy Co-op (Ansan, Korea) for preparing whipping cream samples stabilized with MPI/κ-Car MC and MPI/κ-Car EC.
MPI/κ-Car MC and MPI/κ-Car EC were prepared following the method of Seo and Yoo (2021b). MPI and κ-Car were dissolved at concentrations of 1% (w/w) in distilled water for 3 h at 25°C and 90°C, respectively. The MPI/κ-Car EC was produced by blending the dissolved MPI and κ-Car solutions for 30 min in a 1:1 ratio, and the MPI/κ-Car MC was prepared by heating the MPI/κ-Car EC solution with continuous shaking. The reaction temperature was set at 65°C to minimize protein denaturation, and the 24 h reaction time was determined according to the result in a previous study. Conjugation between the MPI and the κ-Car was identified by means of gel electrophoresis and size-exclusion chromatography (Seo and Yoo, 2021b).
Whipping cream samples stabilized with different concentrations of MPI/κ-Car MC and MPI/κ-Car EC (0%, 0.1%, and 0.2% w/w) were prepared by mixing 80% (w/w) fresh cream, and different amounts of MPI/κ-Car MC and MPI/κ-Car EC solutions, and distilled water for 10 min at 65°C by using a stirrer (Eurostar 20, IKA®, Staufen, Germany) operated at 1,000 rpm. Detailed formulations of these whipping cream samples are summarized in Table 1. The homogenization of blended mixes was carried out using a two-stage valve homogenizer (APV-1000, Invensys APV, Albertslund, Denmark) at pressures of 50 bar, and 10 bar was maintained in the second stage valve. The homogenized samples were immediately cooled and stored overnight at 5°C. The total solid and fat contents of the whipping cream samples were analyzed by the oven drying method and the Gerber method, respectively (AOAC, 2005).
The rheological properties of the whipping cream samples were measured by the method of Seo and Yoo (2021a) with some modifications using the Haake RheoStress 1 (Haake GmbH, Karlsruhe, Germany). The flow behavior was investigated in shear rates ranging from 0.4 to 500 s–1. The power-law model Eq. (1) was used to determine the steady shear rheological properties, and apparent viscosity (ηa,50) of samples was calculated at 50 s–1 by using the K (consistency index) and n (flow behavior index) values.
The dynamic rheological properties were also measured by the frequency sweep test, and G′ (storage modulus), G″ (loss modulus), and tan δ (G′/G″) values were used to describe the viscoelastic behavior of samples.
The fat droplet size and distribution of the whipping cream samples were analyzed by the method of Seo et al. (2018) using the Mastersizer 3000 (Malvern Instruments, Worcestershire, UK). The D[3,2], D[4,3], and span values were used to determine the fat droplet size distribution of the whipping cream samples. The D[3,2], D[4,3], and span values are surface-weighted mean, volume-weighted mean, and dispersion index, respectively, which were calculated from Eq. (2)–(4), respectively:
where di is the average diameter of the i class interval, and ni is the number of droplets with diameter di. The Dv10, Dv50, and Dv90 values are average fat droplet sizes corresponding to the cumulative distribution at 10%, 50%, and 90%, respectively.
The stability of the whipping cream samples was evaluated through acceleration testing at 40°C by using a multiple light scattering spectroscopy (Turbiscan Tower, Formulaction, Toulouse, France). The whipping cream samples with different levels of MPI/κ-Car MC and MPI/κ-Car EC were transferred to 20-mL glass tubes, separately, and changes in the backscattering (BS) intensity were measured to investigate the destabilization mechanisms. The glass tubes were scanned from the bottom to the top at intervals of 15 min for 24 h.
All whipping cream samples were stored at 5°C for 24 h, and 500 g of each of the whipping cream samples was whipped for 4 min in a stand mixer bowl (KitchenAid Heavy Duty, Max wattage 325 W, KitchenAid, Benton Harbor, MI, USA) to evaluate their whipping properties. The overrun value of the whipped creams was measured by weighting an equal volume of whipping cream and whipped cream, and it was calculated using the following Eq. (5):
where W1 and W2 are the masses of the whipping cream and whipped cream, respectively.
Whipped creams (50±3 g) were placed on a wire screen mesh, and the serum loss dripping through the wire screen mesh was collected at 24±1°C and weighed after 5 h. SD was calculated as a percentage (w/w) of serum loss from the whipped cream by using the following Eq. (6):
where W1 and W2 denote the masses of serum loss and whipped cream, respectively.
Results and Discussion
The flow behaviors of the whipping cream samples stabilized with MPI/κ-Car MC and MPI/κ-Car EC are summarized in Table 2. All samples exhibited shear-thinning behavior with lower flow behavior index values (n=0.27–0.47) than 1 in the shear rate range from 0.4 to 500 s–1. The shear-thinning behavior of dairy creams can be explained in terms of the breaking effect of flocculated fat droplets, which reduces the apparent viscosity at high shear rates (Derkach, 2009; Seo et al., 2018). Moreover, the whipping creams exhibited higher shear-thinning behaviors with increasing concentrations of MPI/κ-Car MC and MPI/κ-Car EC. This result is consistent with that of Kováčová et al. (2010), who reported that the flow behavior index (n) of whipping cream decreased as the κ-Car concentration increased from 0% to 0.04%. Carrageenan can induce the shear-thinning behavior due to its flow-resistant entangled network (Javidi et al., 2016; Velásquez-Cock et al., 2019). As a result, the shear-thinning behavior of whipping creams with MPI/κ-Car MC and MPI/κ-Car EC can be explained based on the destruction of the flocculated fat droplets and compact entanglements of κ-Car.
The ηa,50 (apparent viscosity) and K (consistency index) of the whipping cream samples increased as the MPI/κ-Car EC and MPI/κ-Car MC concentrations increased. This result was caused by an increase in the concentration of carrageenan aggregates in the aqueous phase of whipping creams (Ji et al., 2008). In terms of the ηa,50 and K values, the whipping cream samples stabilized with the MPI/κ-Car EC exhibited higher ηa,50 and K values than the whipping cream samples stabilized with the MPI/κ-Car MC at the same concentration. Milk fat droplets stabilized with protein/polysaccharide EC are predominantly surrounded by protein molecules, and most polysaccharide molecules are in the aqueous phase, leading to an increase in the apparent viscosity. In case of the protein/polysaccharide MC, the polysaccharide molecule conjugated with protein can be adsorbed on the surface of the fat droplets because of the amphipathic properties of the protein (Seo and Yoo, 2021b). As a result, the ηa,50, and K values of the whipping cream samples stabilized with the MC can be reduced by decreasing the amount of free carrageenan molecules in the aqueous phase. Therefore, we concluded that the flow behavior of the whipping creams stabilized with MPI/κ-Car MC and MPI/κ-Car EC can be influenced by stabilizer concentrations and the conjugation between the MPI and κ-Car molecules.
Changes in G′ (storage modulus) and G″ (loss modulus) are plotted as functions of frequency (ω) in Fig. 1. The dynamic moduli (G′ and G″) of the control sample without MPI/κ-Car MC and MPI/κ-Car EC were not measured, because the widely scattered dynamic data yielded unreliable values. The G? and G? values of the whipping cream samples increased as the concentrations of MPI/κ-Car MC and MPI/κ-Car EC increased, indicating the increased intermolecular interactions. Similar results were reported in cream desserts with different κ-Car concentrations (Míšková et al., 2021). The higher G′ and G″ values probably resulted from κ-Car gel formation at high concentrations. The κ-Car molecules can form the helical aggregation due to the formation of junction regions between κ-Car chains with the strong negative charge owing to sulfate groups (Ji et al., 2008; Seo and Yoo, 2021a). Thus, the increased G′ and G″ values of the whipping cream samples with higher κ-Car concentrations can be attributed to the formation of a strong gel structure by κ-Car molecules.
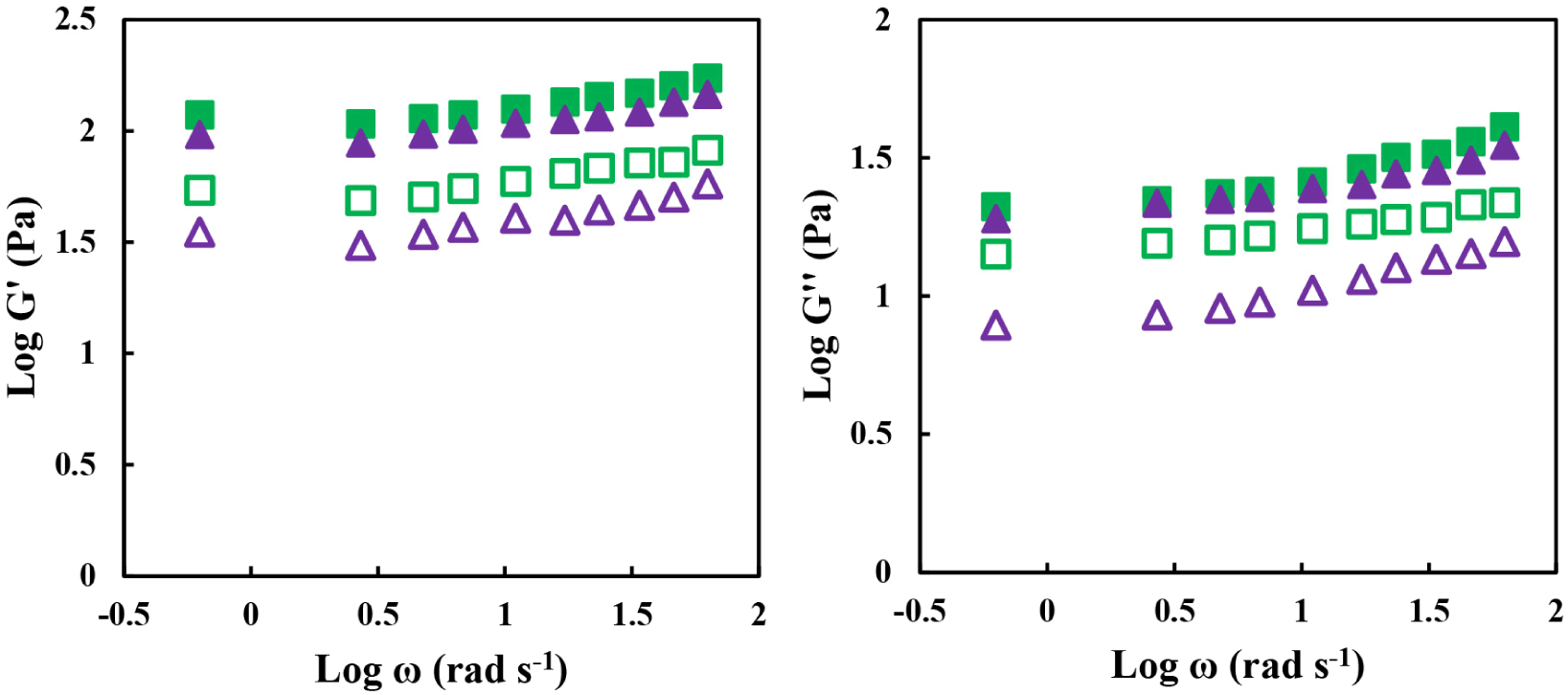
The dynamic moduli (G′ and G″) of the whipping cream samples stabilized with MPI/κ-Car MC were lower than those of the whipping cream samples stabilized with MPI/κ-Car EC at the same concentration (Table 3). This result is consistent with that of Sun et al. (2011), who reported lower G′ and G″ values of whey protein isolate/dextran conjugates in the gel structure system. The conjugation caused by the Maillard reaction between protein and polysaccharide can induce changes in properties such as surface charge, polarity, and molecular interaction (Consoli et al., 2018). Seo and Yoo (2021b) reported the conformational change of MPI/κ-Car conjugates through fluorescence emission analysis. Therefore, the lower G′ and G″ values of the whipping cream samples stabilized with MC can be ascribed to the steric hindrance effect induced by the structural changes due to the conjugation between MPI and κ-Car, which can inhibit the helical aggregation of κ-Car molecules (Spotti et al., 2014).
All of the whipping cream samples stabilized with MPI/κ-Car MC and MPI/κ-Car EC exhibited tan δ (G″/G′) values lower than 1, meaning that the elastic behavior is more pronounced than the viscous behavior. The tan δ of the whipping cream samples decreased as the concentrations of MPI/κ-Car MC and MPI/κ-Car EC increased. However, no significant differences in tan δ values were observed between MPI/κ-Car EC and MPI/κ-Car MC at the same concentration. The decrease in the tan δ value with increasing MPI/κ-Car MC and MPI/κ-Car EC concentrations may be ascribed to intermolecular interactions, and this strong interaction can be attributed to helical aggregation at high κ-Car concentrations, as mentioned above. The electrostatic interaction between the negatively charged κ-Car molecules and the positively charged region of the casein micelles in MPI can lead to the formation of the elastic gel structure (Míšková et al., 2021; Spagnuolo et al., 2005). Based on these observations, we can conclude that the rheological properties of the whipping cream samples were strongly influenced by the addition of MPI/κ-Car MC and MPI/κ-Car EC and by the conjugation between MPI and κ-Car molecules.
The droplet sizes and distributions of the whipping cream samples with different levels of MPI/κ-Car MC and MPI/κ-Car EC are illustrated and summarized, respectively, in Fig. 2 and Table 4. The D[3,2] and D[4,3] values of the whipping cream samples stabilized with MPI/κ-Car MC and MPI/κ-Car EC were considerably higher than those of the control sample. The elevated D[3,2] and D[4,3] values can be attributed to the formation of milk fat globule clusters by κ-Car with long thin stranded structure, which can be adsorbed on casein micelles and form interconnections between casein micelles on different fat droplets (Martin et al., 2006). According to Guzey and McClements (2006), the presence of excess polysaccharide molecules in the aqueous phase can cause the flocculation of fat droplets through the depletion mechanism. Therefore, the larger droplet size of the whipping cream samples with MPI/κ-Car MC and MPI/κ-Car EC can be explained using the depletion effect and the interconnection of milk fat droplets by κ-Car molecules.
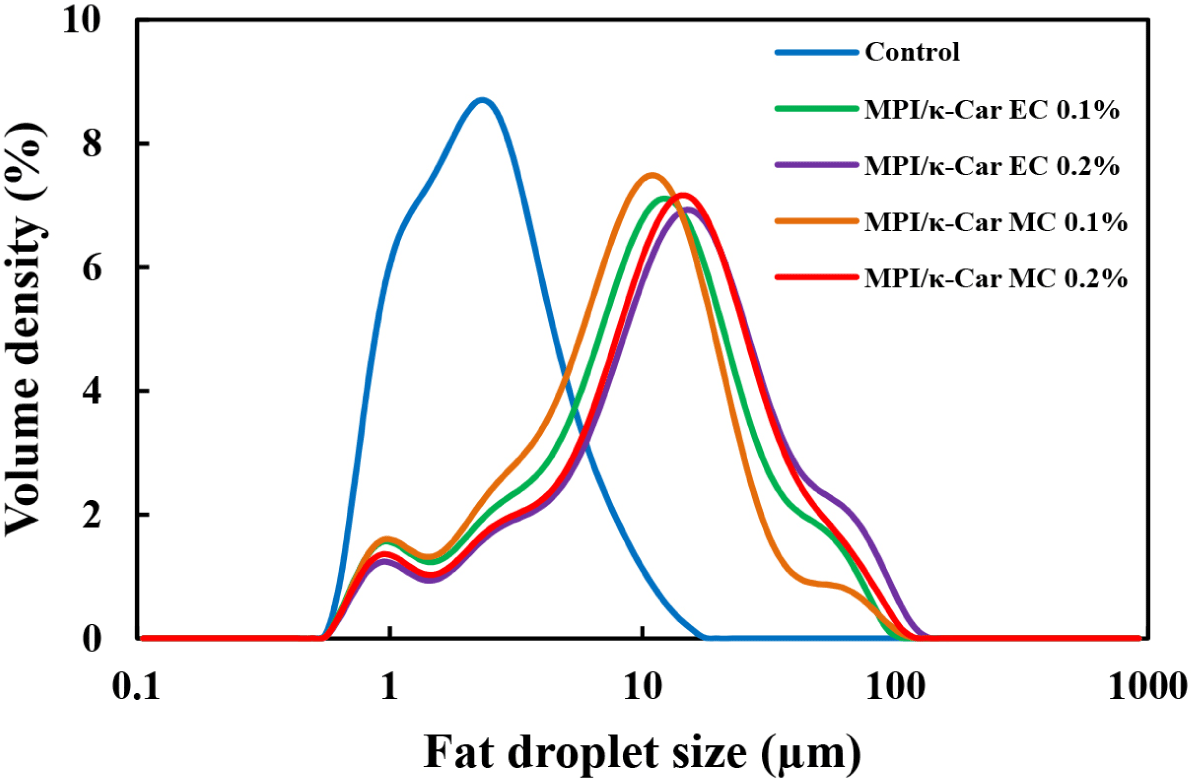
The span values of the whipping cream samples increased as the concentration of MPI/κ-Car MC and MPI/κ-Car EC increased, indicating that the whipping creams with MC and EC had a broader size distribution than that of the control sample. At the same concentration, the whipping cream samples with MPI/κ-Car MC had lower span values than those of the whipping cream samples with MPI/κ-Car EC. This result may be attributed to the inhibition of depletion-flocculation owing to the steric repulsion generated by MC on the droplet surface. Covalent attachment of κ-Car molecules to milk proteins can increase the thickness of the interfacial surface on milk fat droplets, leading to increased steric repulsion between the milk fat droplets (Gu et al., 2017; Guo et al., 2019). Seo and Yoo (2021b) reported that the stability of oil-in-water emulsions stabilized with MPI/κ-Car conjugates was improved owing to the strong steric hindrance between the fat droplets due to the adsorption of milk proteins linked to the κ-Car molecules on the surfaces of fat droplets. As a result, emulsification properties can be improved through conjugation between the MPI and κ-Car molecules.
In the present study, Turbiscan was used to investigate the destabilization mechanisms of the whipping creams stabilized with MPI/κ-Car MC and MPI/κ-Car EC. This instrument can evaluate the real dispersion state of emulsion foods by measuring changes in the transmission light and BS light, which can be used to predict the destabilization mechanisms of emulsions that cannot be observed with the naked eye (Feng et al., 2020; Zheng et al., 2018). Changes in the BS profiles of whipping cream samples over 24 h at 40°C as a function of the height of the glass tubes are presented in Fig. 3. The BS profile of the control sample (whipping cream without MPI/κ-Car MC and MPI/κ-Car EC) exhibited a decrease in BS intensity at the bottom of the glass tubes (at a height of 0–5 mm) and an increase in BS intensity at the top of glass tubes (at a height of 40 mm) during storage. These results indicate that milk fat droplets migrate to the upper part, which decreases the fat droplet concentration at the bottom of the glass tubes (Boostani et al., 2020). A similar tendency in the BS curve was observed for the other whipping cream samples with MPI/κ-Car MC and MPI/κ-Car EC. Based on these observations, we conclude that the whipping cream was destabilized according to the creaming mechanism.
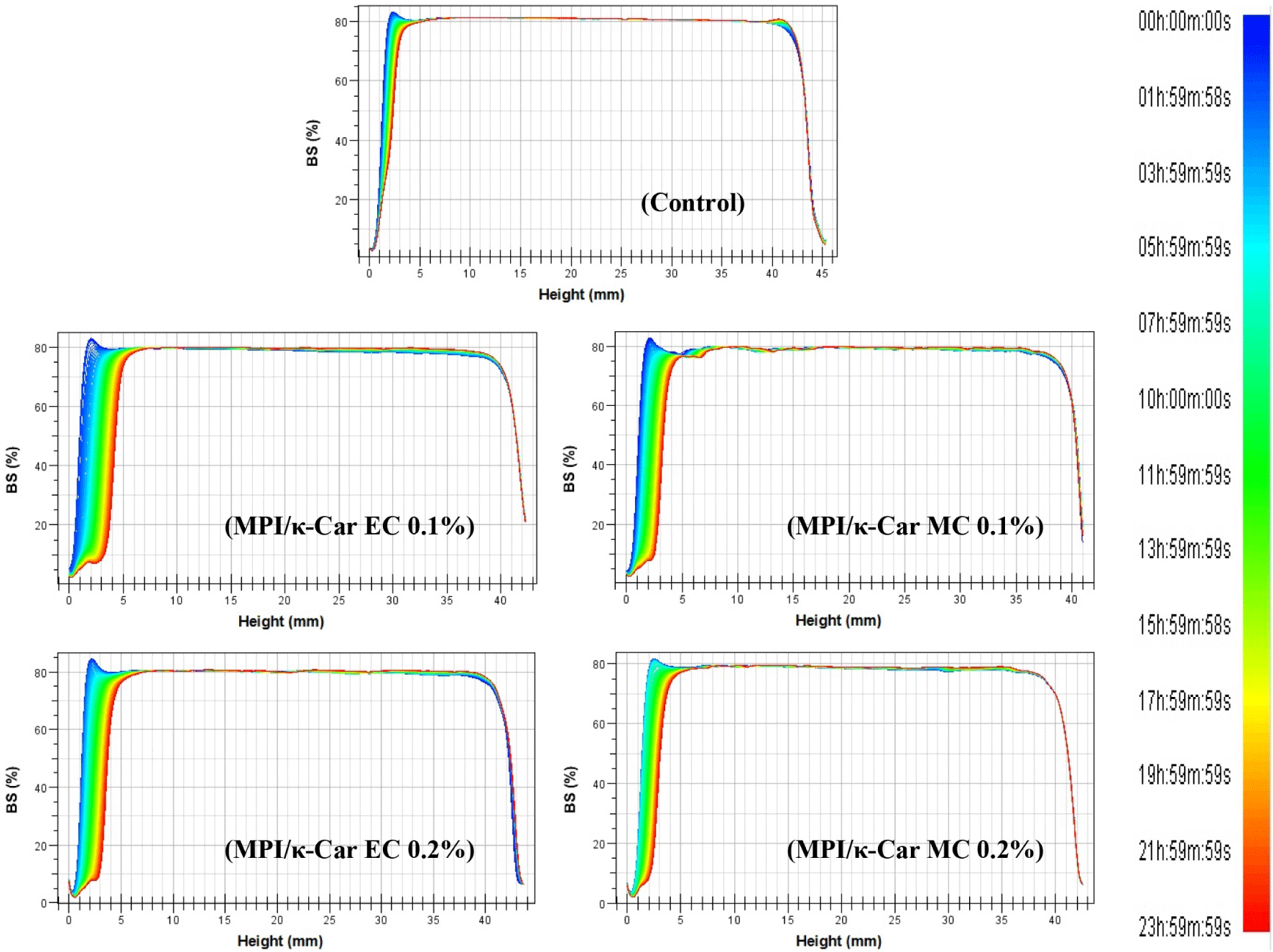
The BS intensity profiles of the whipping creams stabilized with MPI/κ-Car MC and MPI/κ-Car EC exhibited broader fluctuations than that of the control sample, meaning that the stability of the whipping creams decreased with the addition of MC and EC. This result may be caused by the presence of excess free κ-Car molecules in the aqueous phase, which can lead to depletion-flocculation (Guzey and McClements, 2006; Seo and Yoo, 2021a). In case of the whipping creams with the same concentration of MPI/κ-Car MC and MPI/κ-Car EC, the BS profiles of the whipping creams stabilized with MPI/κ-Car MC exhibited narrower fluctuations than those of the whipping creams stabilized with MPI/κ-Car EC. This result indicates that the whipping creams stabilized with MC underwent creaming at a slower rate than the whipping creams stabilized with EC. The improved stability can be explained by the ability of the MC adsorbed on the surface of milk fat droplets to form a thick layer and effectively prevent flocculation between the milk fat droplets owing to strong steric hindrance (Pirestani et al., 2017; Seo and Yoo, 2021b). Consequently, the MC derived from MPI and κ-Car can improve the physical stability of whipping cream systems with protein/polysaccharide complexes owing to the steric hindrance effect resulting from their adsorption on the surface of milk fat droplets.
All whipping cream samples reached the maximum volume within 4 min, and the control sample without MPI/κ-Car MC and MPI/κ-Car EC had the highest overrun value (130%). This result can be attributed to the higher foaming ability of milk proteins, which can be adsorbed rapidly on the foam interface and enhance air incorporation (Dhungana et al., 2020; Long et al., 2016). The overrun values of the whipping cream samples decreased with the addition of MPI/κ-Car MC and MPI/κ-Car EC, and the decrease was remarkable when the stabilizer concentration was 0.2%, as shown in Fig. 4A. A similar tendency was observed by Ghribi et al. (2021), who reported that the overrun value of whipping creams containing soy protein isolate/κ-Car mixture decreased as the κ-Car concentration increased. According to Biasutti et al. (2013), the overrun value is influenced by viscosity, and a high viscosity level can prevent vigorous agitation and reduce the incorporation of gas bubbles, resulting in a lower overrun value. At the same concentrations of MPI/κ-Car MC and MPI/κ-Car EC, no significant difference in the overrun value was observed between the whipping creams with MC and those with EC. Consequently, the overrun values of the whipping creams stabilized with MPI and κ-Car were not influenced by the conjugation between MPI and κ-Car molecules, but they were strongly influenced by the concentrations of MPI/κ-Car MC and MPI/κ-Car EC, which can increase the viscosity of whipping creams.
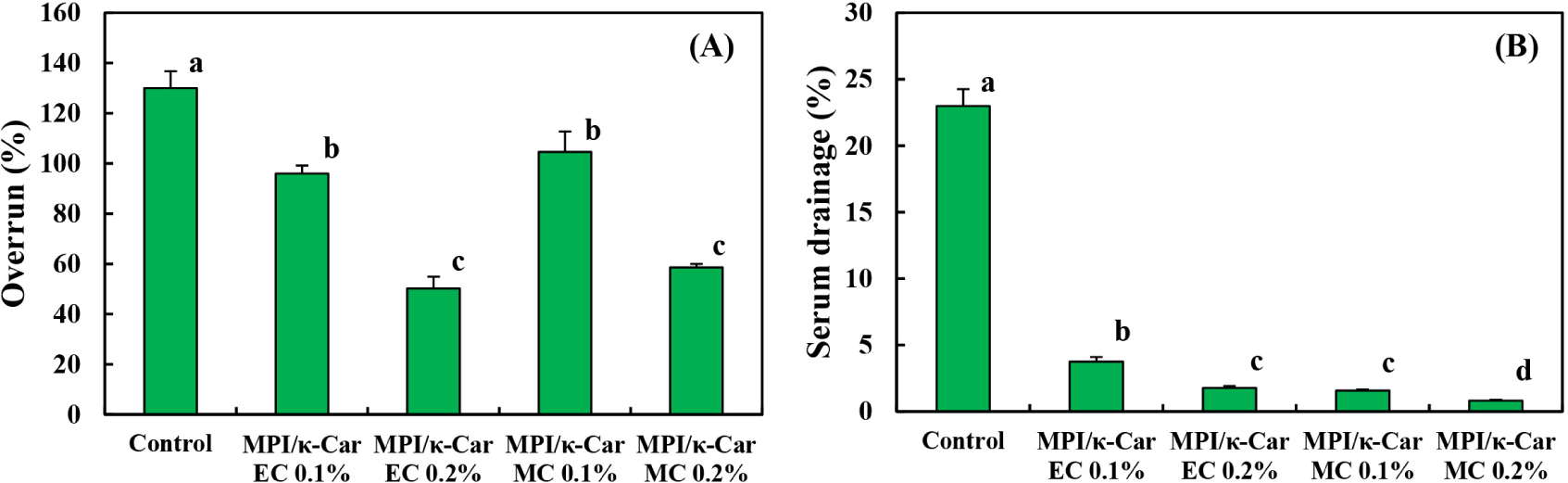
SD was measured to evaluate the stability of the whipped creams stabilized with MPI/κ-Car MC and MPI/κ-Car EC, and the SD values of the whipping cream samples are shown in Fig. 4B. In this study, the SD values of the whipping cream samples ranged from 0.94% to 23%, and the control sample had a considerably higher SD value than those of the whipping creams stabilized with MPI/κ-Car MC and MPI/κ-Car EC. This result indicates that the whipping cream samples without MC and EC had a fragile foam structure, resulting in higher serum loss. The serum loss of the whipping cream samples was improved with the addition of MPI/κ-Car MC and MPI/κ-Car EC, and their SD values decreased as the concentration of MC and EC increased. This improved stability can be attributed to the formation of the gel structure owing to the aggregation of κ-Car helices (Ji et al., 2008). According to Ghribi et al. (2021), κ-Car can enhance foam stability by increasing film rigidity and viscoelasticity, which can stabilize air bubbles.
In case of the whipping cream samples with the same concentration of MPI/κ-Car MC and MPI/κ-Car EC, the whipped creams with MPI/κ-Car MC exhibited slightly lower SD values than the whipped creams with MPI/κ-Car EC, indicating that MC can form more stable whipped creams than EC. These results can be explained by the enhanced water-holding ability of MC. Capar and Yalcin (2021) reported that the water-holding ability of whey protein isolate was reinforced after conjugation with the κ-Car molecule through the Maillard reaction. The syneresis phenomenon of κ-Car gel can be improved through conjugation with proteins due to the steric hindrance effect, which limits the formation in junction regions between κ-Car chains that can squeeze water from the κ-Car gel network (Ji et al., 2008; Wang et al., 2018). Based on these observations, we concluded that the stability of whipped cream can be reinforced by adding MPI/κ-Car MC, and MC can effectively improve the stability of whipped creams rather than EC owing to the enhanced water-holding ability due to conjugation.
Conclusion
Protein/polysaccharide complexes are considered good alternatives to synthetic emulsifiers and stabilizers for producing stable emulsion foods, and protein-polysaccharide conjugation through the Maillard reaction is an effective strategy to improve functional properties. Thus, we attempted to apply the MPI/κ-Car MC to whipping creams to improve their rheological and whipping properties in this study. The rheological parameters (ηa,50, K, G′, and G″) of the whipping cream samples stabilized with MPI/κ-Car MC were lower than those of the whipping cream samples stabilized with MPI/κ-Car EC owing to the steric hindrance effect of conjugation. The whipping cream samples stabilized with MPI/κ-Car MC and MPI/κ-Car EC exhibited higher D[3,2], D[4,3], and span values than those of the control sample, but the whipping cream samples with MC exhibited a narrower distribution than that of the whipping cream samples stabilized with EC at the same concentration, indicating that the emulsification ability can be improved owing to the conjugation between MPI and κ-Car molecules. Although the addition of 0.1% MPI/κ-Car MC and MPI/κ-Car EC to the whipping cream samples marginally reduced the overrun value, serum loss was effectively reduced upon the addition of MC and EC. In particular, the whipping cream samples stabilized with MC had lower SD values than those stabilized with EC due to enhanced water-holding ability. However, the concentration of the MPI/κ-Car MC should be considered from a sensory point of view, because an excessively high MPI/κ-Car MC concentration can influence the color of whipping creams, making them appear more yellow. Consequently, MC can be used as natural emulsifiers and stabilizers instead of EC in whipping cream products to obtain the desired rheological and whipping properties.