Introduction
Meat is a rich source of protein, vitamins, and minerals. Due to the perishable nature of meat, it has been processed in various ways for preservation. Sausage fermentation may be one of the earliest forms of meat processing. The meat is preserved long-term by fermentation and drying after filling the casing with a mixture of minced meat, fat, salt, color retention agent, sugar, and spices (Campbell-Platt, 1995). Fermented sausages have been produced in Southern and Central Europe, especially in Germany, Italy, Spain, and France, with the highest production and consumption rates per capita (Lücke, 1998). Fermented sausage production is approximately 200,000 tons annually in Spain and 110,000 tons in France (Safa et al., 2015). In 2014, fermented sausage production in Norway and Finland was 7,300 tons and 7,000 tons, respectively (Holck et al., 2014). According to the Ministry of Food and Drug Safety, fermented sausage production increased by 77% from 272 tons in 2018 to 614 tons in 2021, and sales during the same period increased by 67% from 266 tons to 445 tons (MFDS, 2019, 2022). However, most fermented sausages in Korea are still imported, even though there is a significant increase in the production of fermented sausages (Jung and Yoon, 2020).
The main ingredients of fermented sausage are meat, fatty tissue, carbohydrates, curing agents, spices, and starter culture (Lücke, 1998). The microorganisms that are primarily involved in sausage fermentation include species of lactic acid bacteria, gram-positive and catalase-positive cocci (GCC), molds, and yeasts, mainly including Lactobacillus sakei, Lactobacillus curvatus, Lactobacillus plantarum, Lactobacillus brevis, Lactobacillus buchneri, Lactobacillus paracasei, Staphylococcus xylosus, Staphylococcus carnosus, Staphylococcus saprophyticus, Kocuria varians, Penicillium nalgiovense, Penicillium chrysogenum, and Debaryomyces hansenii (Leroy et al., 2006). During fermentation, the fermented sausage starter cultures should adapt to environmental conditions, such as high salt concentrations and low temperatures (Lee et al., 2006). Moreover, they are required to compete with other microorganisms by rapid growth and pH lowering so that various harmful microorganisms can be suppressed, securing microbiological safety (Lee et al., 2006). Furthermore, the starter cultures should be resistant to sodium-nitrite scavengers and sodium nitrite, which is added for fermented sausage preservation (Leistner, 1995). The starter cultures are responsible for the functions mentioned above and the sensual quality of the fermented sausage. During the short fermentation period, the starter cultures generate distinctive tastes, flavors, and colors of the sausages (Kunz and Lee, 2003). Likewise, the starter cultures play a role in improving the texture and flavor of the fermented sausages.
In this review, the requirement and role of starter cultures in sausage fermentation are intensively reviewed, and fermented sausage quality improvement by starter cultures is discussed.
Requirement for Starter Culture in Fermented Sausages
The growth of harmful microorganisms, such as foodborne pathogens, in fermented sausages can be inhibited by applying starter cultures to them, lowering their pH (Laranjo et al., 2019). The starter culture should be able to inhibit toxin production by other microorganisms. P. nalgiovense, widely used for starter culture, can inhibit the production of toxin-producing fungi, ensuring the safety of fermented sausages (Bernáldez et al., 2013).
The starter culture should grow well under the physical and chemical conditions of the sausages and be harmless to humans. To ensure the safety of starter cultures, hemolysis, toxin production, and biogenic amine production by the microorganisms in the starter culture must be evaluated (Jeong and Lee, 2015; Sirini et al., 2022). Some starter cultures produce biogenic amines in fermented sausages, and thus, starter cultures producing no biogenic amines need to be developed, and processing methods such as packaging, additives, hydrostatic pressure, and smoking can also reduce biogenic amines (Doeun et al., 2017; Özogul and Hamed, 2018).
During the ripening of fermented sausages, enzyme activities derived from starter culture can positively affect the sensual elements of the fermented sausages (Wang et al., 2019). Thus, the microorganisms of the starter culture should be evaluated for degradation of carbohydrates, proteins, peptides, and lipids to produce a pleasant aroma and taste in fermented sausage.
Starter culture plays a role in maintaining the color of fermented sausages by producing nitrate reductase and nitrite reductase, which reduce nitrate and nitrite, respectively. For example, S. carnosus has been used to maintain the color of the fermented sausage by forming red nitrosomyoglobin, which is produced by nitrate reductase (Götz, 1990; Löfblom et al., 2017; Neubauer and Götz, 1996).
Changes in Free Fatty Acids and Volatile Compounds by Starter Cultures
Water content and pH in fermented sausages decrease during fermentation, and several biochemical reactions, including lipolysis, occur (Fernández et al., 2000; Lücke, 2000). Lipids in fermented sausages can be oxidized to free fatty acids (Chen et al., 2017; Gandemer, 2002), enhancing the flavor of fermented sausages (Flores and Toldrá, 2011). Lactic acid bacteria, yeasts, and molds are known to hydrolyze triglycerides and increase the free fatty acids content in fermented sausages (Chen et al., 2017; Ordóñez et al., 1999; Sanz et al., 1988). Subsequently, free fatty acids undergo enzymatic and non-enzymatic oxidation, yielding alcohol, aldehydes, carboxylic acids, and other flavor compounds (Casaburi et al., 2007; Fernández et al., 2000; Melgar et al., 1990). Thus, applying microorganisms with high lipolytic activity to the manufacturing process might improve the sensory quality of fermented sausages (Casaburi et al., 2007).
During sausage fermentation, Pediococcus pentosaceus and S. xylosus increased the contents of total free fatty acids from 0.6% to 6.8% (Johansson et al., 1994). Karsloğlu et al. (2014) investigated free fatty acid contents and lipolytic changes in fermented sausages inoculated with commercial starter cultures, including Lactobacillus sake, S. carnosus, S. xylosus, and P. pentosaceus. Oleic acid, linoleic acid, linolenic acid, and palmitic acid were the predominant fatty acids in the fermented sausage stored for 120 days. The oleic acid content had increased from 1.77%–1.99% to 6.56%–13.01%. Other researchers compared the changes in saturated fatty acid (SFA), monounsaturated fatty acid (MUFA), and polyunsaturated fatty acid (PUFA) levels in fermented sausages. When fermented sausages were inoculated with L. sakei and S. xylosus, the final SFA and PUFA contents increased, whereas the MUFA content was lower than that in sausages without a starter culture (Hu et al., 2007). In boar sausages, D. hansenii increased MUFA and PUFA contents, and it supports the lipolysis in fermented sausages (Patrignani et al., 2007). All these findings indicate that the use of starter culture contributes degradation of lipids in fermented sausages.
Volatile compounds may be produced from the degradation of lipid in fermented sausages when starter cultures are used (Table 1). The inoculation of D. hansenii in Salchichón changed the volatile compound profile at 54 days of fermentation, and the inoculated sausages had higher volatile compounds (58 volatile compounds) than those (41 volatile compounds) in the uninoculated sausages. Moreover, heptanal, octanal, and nonanal, known as cured-ham-like flavor compounds, were only detected in the inoculated batches (Andrade et al., 2010; Carrapiso et al., 2002). Corral et al. (2015) manufactured fermented sausages with low fat and salt content, and inoculated with D. hansenii in the fermented sausages. Compared to the uninoculated fermented sausages, the sausages with D. hansenii showed a higher content of 2-pentylfuran, which has a metallic odor. When fermented sausages were inoculated with L. sakei and combinations of different Staphylococcus species, the changes in volatile compounds differed with each Staphylococcus strain. In addition, only hexanal significantly decreased with the addition of starter cultures, reflecting decreased rancid flavor in the fermented sausages (Fonseca et al., 2013).
Starter culture | Major volatile compounds | Minor volatile compounds | References |
---|---|---|---|
Debaryomyces hansenii | Decane, 1-propanol, 1-butanol, 2-propanone, 2-pentanone, 2-heptanone, pentanal, heptanal, octanal, nonanal, propanoic acid, hexanoic acid, hexadecenoic acid | Hexane, 2-methylpentane, 3-methylpentane | Andrade et al. (2010) |
Propanal, 1-propanol, 2-methylfuran, 2-pentylfuran, hexanoic acid, 2-ethyl-1-hexanol, decanoic acid | Tetrahydrofuran, 2,5-dimethylfuran, heptanoic acid | Corral et al. (2015) | |
Lactobacillus sakei, Staphylococcus equorum | Hexane, nonanoic acid | Octane, hexanal, 3-ethyl-heptane, nonane, 3-ethyl-4-methyl-hexane, 3-methyl-nonane, 2,2,6-trimethyl-octane, decane, 3,7-dimethyl-nonane, 3,7-dimethyl-decane, undecane, 3,3-dimethyl-hexane, 2,2,5-trimethyl-decane, 5-ethyl-5-methyl-decane, dodecane, tridecane | Fonseca et al. (2013) |
L. sakei, Staphylococcus epidermidis | Hexane, 2,2,6-trimethyl-octane, 5-ethyl-5-methyl-decane, nonanoic acid | Octane, hexanal, 3-ethyl-heptane, nonane, 3-ethyl-4-methyl-hexane, 3-methyl-nonane, decane, 3,7-dimethyl-nonane, 3,7-dimethyl-decane, undecane, 3,3-dimethyl-hexane, 2,2,5-trimethyl-decane, dodecane, tridecane | |
L. sakei, Staphylococcus saprophyticus | Hexane, 3-ethyl-heptane, 3-ethyl-4-methyl-hexane, 3-methyl-nonane, decane, 3,7-dimethyl-decane, 5-ethyl-5-methyl-decane | Octane, hexanal, nonane, 2,2,6-trimethyl-octane, 3,7-dimethyl-nonane, undecane, 3,3-dimethyl-hexane, 2,2,5-trimethyl-decane, dodecane, tridecane, nonanoic acid | |
Penicillium aurantiogriseum (intracellular cell free extract) | 2-Butanone, 2-methylfuran, 2-ethylfuran | 1-Penten-3-ol, 2-pentanone | Bruna et al. (2001) |
P. aurantiogriseum (spore suspension) | - | 1-Penten-3-ol, 2-butenal, pentanal, hexanal, 2-hexanal, heptanal, nonanal, decanal, 2-butanone, 2-pentanone, 2-heptanone, 2-methylfuran | |
P. aurantiogriseum (intracellular cell fee extract, spore suspension) | - | 1-Penten-3-ol, 2-butenal, pentanal, hexanal 2-hexanal, heptanal, nonanal, decanal, 2-butanone, 2-pentanone, 2-heptanone, 2-methylfuran |
Few studies analyzed volatile compounds in fermented sausages inoculated with mold starters. Bruna et al. (2001) analyzed the difference in volatile compounds between the inoculation of Penicillium aurantiogriseum and the intracellular cell free extract of P. aurantiogriseum. The volatile compounds such as 1-penten-3-ol and 2-pentanone, which have unpleasant flavor, were significantly lower in inoculated samples than in uninoculated samples, regardless of the inocula type. On the other hand, no significant differences were observed in the free fatty acids profile between starter-culture-inoculated and uninoculated fermented sausages (Corral et al., 2015; Lorenzo et al., 2014). The different lipolytic activities might cause this among strains (Patrignani et al., 2007). These results suggest that using starter cultures increases free fatty acid contents and positively affects the production of volatile compounds in fermented sausages, which improves the flavor of fermented sausage (Fig. 1).
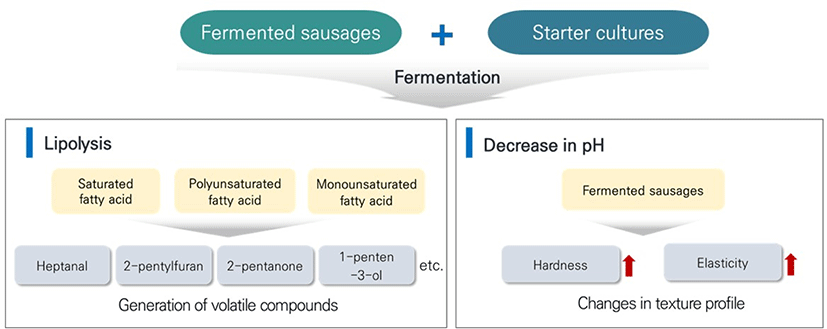
Changes in Texture Profiles of Fermented-Sausage
The physiochemical characteristics of fermented sausages can be evaluated during ripening or storage. One of the characteristics is the texture profile, which includes cohesiveness, hardness, or chewiness. The protein structure of the fermented sausage is responsible for the proper texture formation after salting, chopping, and fermentation (Katsaras and Budras, 1992). Texture formation is closely associated with the reduction of pH in fermented sausages. During ripening, the protein structure of the fermented sausage is denatured by organic acids such as lactic acid, and the moisture content decreases gradually. Moreover, unstable coagulation bonds in the protein structure are substituted with condensed bonds, which result in the transformation from the sol state to the gel state (Katsaras and Budras, 1992). Bozkurt and Bayram (2006), Casaburi et al. (2007), and Gonzalez-Fernandez et al. (2006) confirmed that decreases in pH were negatively correlated with hardness and chewiness. Thus, when the pH and moisture of the sausages decrease, muscle fiber proteins are accumulated, which induces the gel structure. This gel structure increases the hardness and elasticity of the sausages (Essid and Hassouna, 2013; Katsaras and Budras, 1992; Fig. 1 and Table 2).
Physico-chemical property | Changes in texture | References |
---|---|---|
Decrease in pH and water content | Negatively correlated with hardness and chewiness | Bozkurt and Bayram (2006); Casaburi et al. (2007); Gonzalez-Fernandez et al. (2006) |
Proteolysis | Formation of flavor and final texture of fermented sausages. | Dalmış and Soyer (2008); Roseiro et al. (2008) |
Proteolysis is one of the major phenomena that occurs during the ripening of fermented sausages, and it needs endogenous enzymes in meat and microbial enzymes (Dalmış and Soyer, 2008; Roseiro et al., 2008). Proteins degrade into small molecules such as peptides, amines, aldehydes, or amino acids by proteolysis, contributing to the final texture properties and flavor of the fermented sausages (Dalmış and Soyer, 2008; Roseiro et al., 2008; Spaziani et al., 2009).
Benito et al. (2005) showed that the hardness of fermented sausages decreased because of the proteolysis by the fungal protease in the starter culture. Aro et al. (2010) compared proteolysis among fermented sausages inoculated with no starter culture, a mixture of L. sakei and S. carnosus, and a mixture of P. pentosaceus and S. xylosus. The result showed that the microorganisms in the starter cultures broke down myofibrillar and sarcoplasmic proteins into short peptides and other small molecules. Nie et al. (2014) used L. plantarum or P. pentosaceus in fermented sausages, and free amino acid concentrations increased with adding starter culture. Hu et al. (2022) reported that the shear force of dry fermented sausages increased significantly in the samples inoculated with L. plantarum compared to those inoculated with L. sakei, L. culvatus, and Weissella hellenica. Wang et al. (2021) used L. sakei, a mixture of L. sakei and S. xylosus, and a mixture of L. sakei, S. xylosus and S. carnosus in fermented sausages. The result from this study implies that amino acid contents were higher in the sausages inoculated with mixed cultures than those inoculated with a single strain. Ayyash et al. (2020) compared the hardness and proteolysis effect of the dried fermented camel sausage and dried fermented beef sausage inoculated with the same starter culture. The result showed that the hardness of dried fermented camel sausages was significantly lower than that of beef. However, the proteolysis level of the fermented camel sausages was observed to be greater than that of beef. These results indicate that the proteolysis activity in fermented sausages may depend on the starter culture, and the mixed starter culture may cause higher activity than a single strain. In addition, the proteolysis activity may depend on the type of meat used in fermented sausages.
Conclusion
A starter culture in a fermented sausage should be able to inhibit the growth of harmful microorganisms, and the starter culture should be safe for humans. The starter culture plays a role in maintaining red color, breaking lipids into free fatty acid, and producing volatile compounds, which improve the flavor of fermented sausages. In addition, the texture profile of fermented sausages is affected by the proteolysis activity of the starter culture.