Introduction
Cellular agriculture refers to the cells of agricultural products, such as meat, milk, eggs, and seafood, and is being studied as meat stem cell cultures (Fig. 1). The present outlook is that cell cultures of agricultural products will be needed not only in Korea but also worldwide for the following reasons. First, according to a United Nations (UN) report, the population will increase from 7.8 billion in 2021 to approximately 9.5 billion in 2050 (UN, 2015). As a result, there are concerns that a food shortage will occur, and in particular, the need for protein, which maintains and promotes the growth of the human body, will be approximately twice as high (Wikandari et al., 2021).
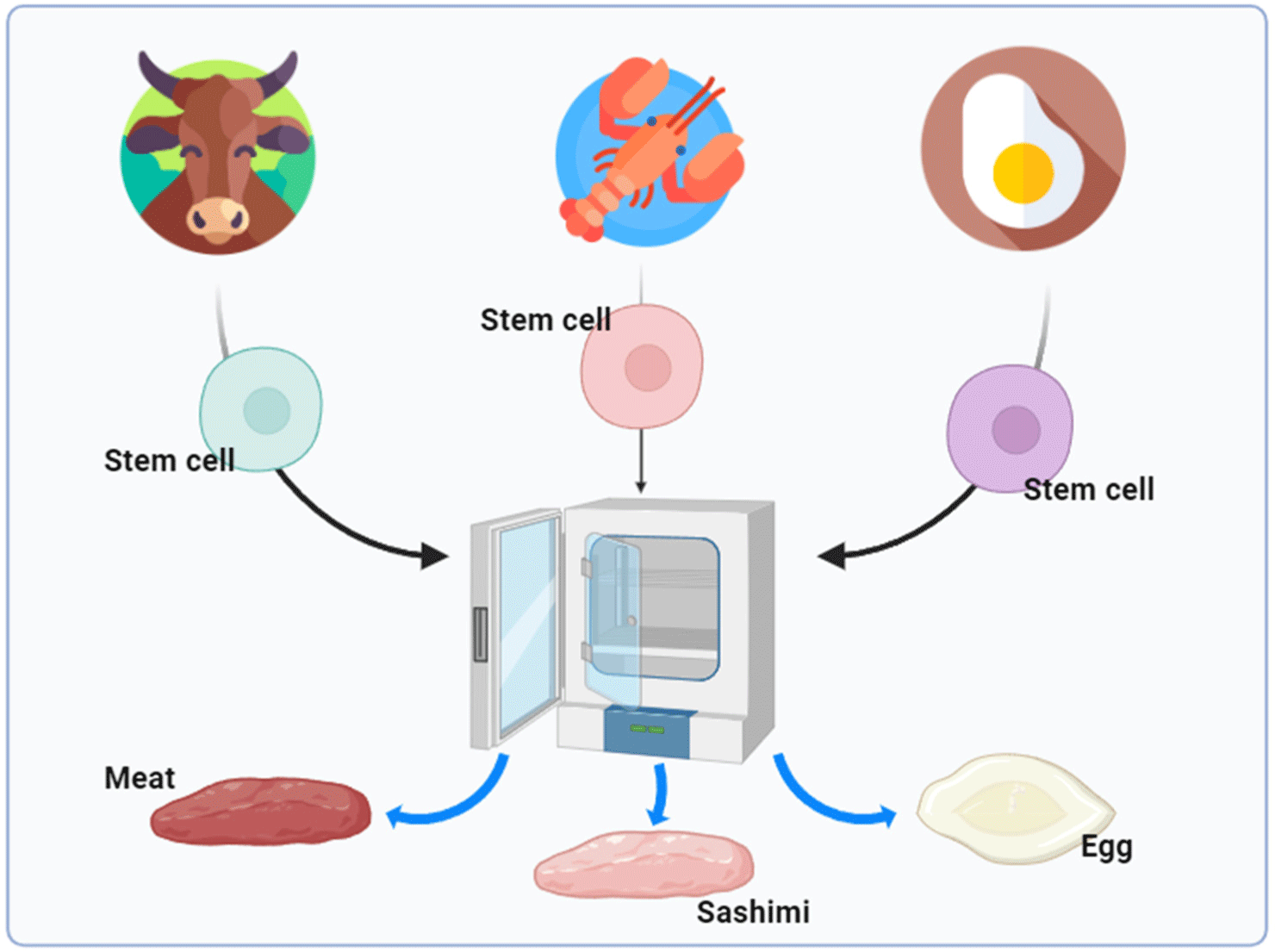
Additionally, some vegetarians with animal welfare concerns may consider consuming cultured meat (Hopkins, 2015). Simply increasing the livestock population is not the clear answer to increases in protein needs, as this can cause many additional problems. As the number of livestock increases, the mass production of crops used as feed (corn, soybeans, etc.) follows, and environmental problems due to gases and excrement generated during livestock ingestion, the absorption of nutrients, and excretion arise (Herrero et al., 2016; Zhou, 2003). Culture meat may also be a way to solve two problems that may arise from livestock diseases: the loss of livestock due to highly infectious diseases, such as avian influenza, African swine fever, or foot-and-mouth disease, and instability in the price of livestock products (Chriki and Hocquette, 2020). Therefore, animal cell culture can be an important way to produce a certain amount of meat.
However, research on cellular agriculture needs to progress more actively and quickly. Until now, this research has mainly been conducted in medical fields such as medicine and pharmacy, especially histology. However, since the development of cultured meat by Professor Post of Mosa Meat in the Netherlands in 2013, cultured meat research has been conducted in various fields (Bodiou et al., 2020). Cultured meat still has various problems directly related to consumption and sales, such as being unfamiliar or causing feelings of distaste, and this is largely thought to be because consumers have not yet actually encountered cultured meat. In 2020, the Singapore Ministry of Food began selling cultured chicken breast developed by the American company Eat Just, and as of 2022, 156 cultured meat companies have been established around the world (Clare et al., 2022). Likewise, the mass production of cultured meat is being studied in every country except Singapore, and it is believed that the future of cultured meat can be accelerated if consumers are approached with a familiar image.
Meat was initially cultured by adhering cells to a flat flask. However, when the cells covered the flask, abnormal proliferation and differentiation occurred, or they died, making mass production difficult (Choi et al., 2020). This occurred because cells grown in a single layer have difficulties excreting waste products, and problems arise in the uptake of nutrients contained in the culture medium (Hubalek et al., 2022). Therefore, the need for cell culture using scaffolds that can replace blood vessels has emerged (Kim et al., 2018). Additionally, because the scaffold has a three-dimensional (3D) shape, it has the additional advantage of being able to be manufactured in larger quantities compared to flask cultures. Research on different scaffold materials and methods is actively underway, and as the quality and quantity of cultured meat are produced differently, research is underway for mass cultivation. Additionally, because cultured meat should not only focus on mass production but also resemble edible meat, research focusing on the quality characteristics of cultured meat such as flavor, appearance, and texture, is necessary.
Therefore, in this review, we investigated the current manufacturing technology of scaffolds to develop cultured meat similar to edible meat, and previous studies on the appearance, flavor, and texture of cultured meat when scaffolds were used in cultured meat.
Classification and Introduction of 3D Scaffolds
The scaffolds mainly used in cultured meat-cell culture include fiber scaffolds, hydrogels, and micro-carrier beads (MC) to maximize the adhesion ability of cells (Fig. 2). A suspension method (floating culture method), which suppresses the tendency of cells to attach and does not use a scaffold, can also be used. These scaffolds are all manufactured by imitating living tissue to directly deliver oxygen and nutrients to cells or remove waste (Bružauskaitė et al., 2016).
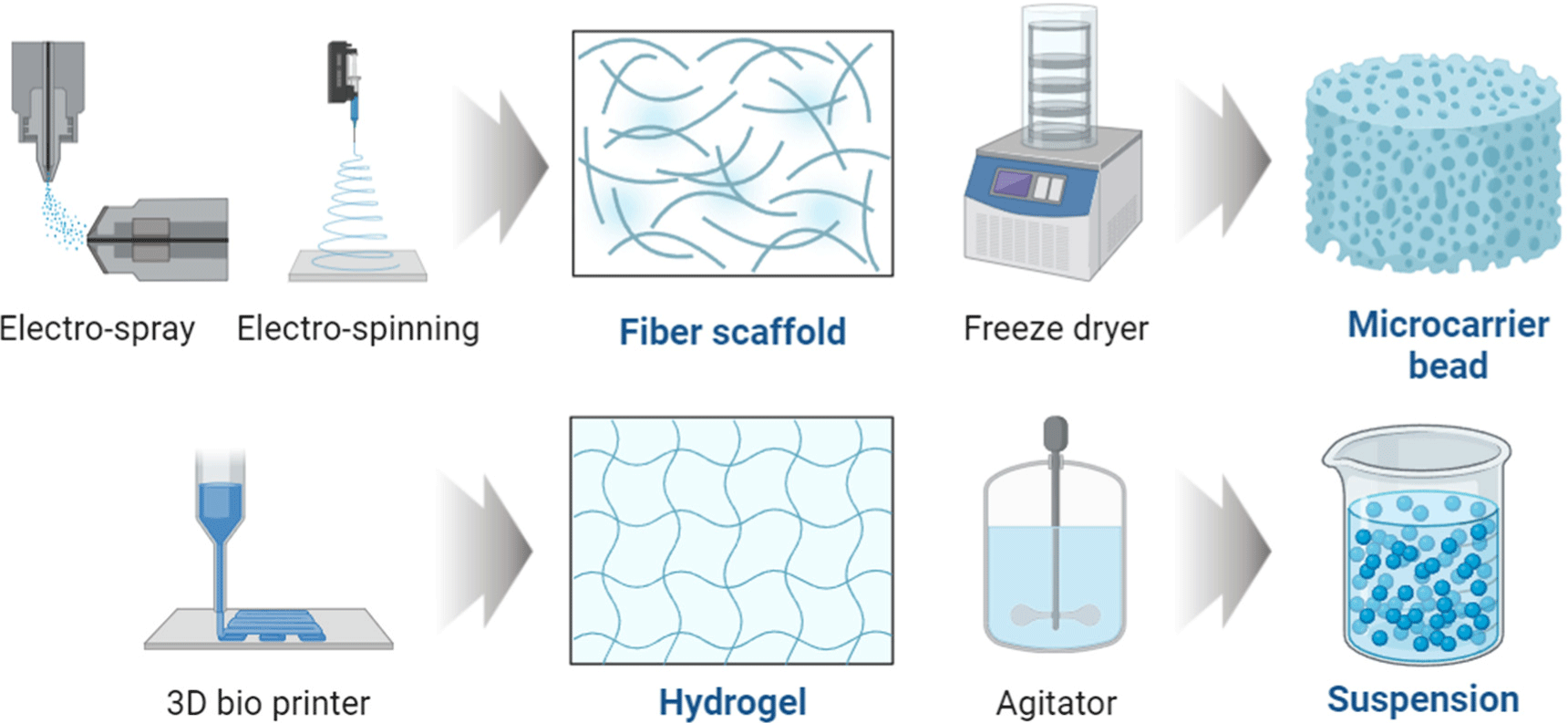
A fiber scaffold is a support in which thin and long fibers produced by electrospinning (ESI) form a matrix for cells to attach to, proliferate, and differentiate (Badami et al., 2006). ESI combines a variety of natural and synthetic polymers into fibers that are randomly or aligned according to the fiber diameter (μm) or the setter’s purpose (Bai et al., 2022). Fiber scaffolds also appear to have an excellent ability to form shapes because they can easily form muscle bundles, and their ability to form aligned fibers facilitates mass production (Feng et al., 2021). Since the proliferation and differentiation ability of cells varies as the fibers are arranged, research continues to determine which shape is most effective.
The second scaffold to be introduced is hydrogels, which are hydrophilic scaffolds made of one or more polymers. Like fiber scaffolds, they are supports that form a network structure to allow cells to adhere better to a planar substrate (Chimene et al., 2020). Hydrogel has the advantage of responding to cell secretion signals by forming bioactive ligands and requires a high moisture content to maintain this ability (Rosales and Anseth, 2016). However, since hydrogel is mainly composed of animal/plant proteins, it can be degraded by the precursor proteins of extracellular proteolytic enzymes, such as matrix metalloproteinase proteins secreted from its own proteins (Samorezov and Alsberg, 2015). Therefore, an accurate analysis of manufacturing materials is required, and efforts are being made to improve chemical and structural aspects, such as porosity and elasticity (Myung et al., 2007). In addition, because hydrogel can retain a large amount of moisture, it is possible to mix substances such as water-soluble growth factors and hormones during design. Thus, research is underway to determine the mixing ratio.
MC have been developed from materials such as cellulose, gelatin, alginate, chitosan, and polystyrene, and most of the materials are used after being molded into a sphere, which induces electrostatic interaction with negatively charged cells through a positive charge coating on the cell attachment surface (Chang and Wang, 2011). In addition to electrostatic coating, the adhesion ability of cells is also improved through coating with proteins such as extracellular matrix. MC culture, similar to a suspension, requires additional mechanical functions, such as a stirrer, to help prevent cells from sticking to the wall. Although it may require more elements than other technologies, it is widely used in industry because it allows for the production of cells in large quantities compared to other culture techniques.
The suspension technique is the method most similar to 2D and has been used for the longest time in 3D cell culture. Like MC, suspended cells grow while floating in the culture medium and require machinery such as a stirrer or impeller (Fenge and Lüllau, 2005). In this method, cells are dispensed into a culture medium and allowed to grow on their own by supplying them with nutrients contained in the suspension or facilitating the excretion of waste products. Although it is easy to study the benefits of additives in suspension cultures, such as fetal bovine serum, and the ability of additives to participate in proliferation and differentiation, it has the disadvantage of being difficult to separate cells from the culture medium, thereby consuming the researcher’s labor and time. Research is underway to easily distinguish cells from the culture medium when changing the culture medium and develop technologies, such as coatings or micro-wells, that can prevent adherent cells from sticking to the wall of the culture plates (Dang et al., 2002; Silk et al., 2010). Although this manuscript does not cover everything, various scaffolds can be used, depending on the cultured meat production technology and purpose.
Scaffold Production Methods
The scaffolds described above are a type of support, and various machines and technologies exist to manufacture them, including ESI/spraying, 3D printing, molding, freeze drying, and decellularization, depending on the composition of the material and the researcher’s design (Table 1).
Production method | Materials | Cells | Introduction | References |
---|---|---|---|---|
Electrospinning | Textured soy protein | Bovine satellite cell | A step forward for the production of cell-based meat as food | Ben-Arye et al., 2020 |
Electrospinning | Polycaprolactone | Endothelial cell | Developing new scaffolds by investigating the interactions between endothelial cells, starch, and polyfibers | Santos et al., 2007 |
Electrospinning | Cellulose fibers | - | Systematic study of electrospinning conditions and application as reinforcing fiber for biocomposites | Han et al., 2008 |
Molding | Soybean powder and gelatin | C2C12 and 3T3-L1 cells | Manufacture cultured meat with a muscle-like texture by adding pre-fat cells that produce mass-produced cultured meat | Li et al., 2022 |
Molding | Polydimethylsiloxane and naringenin | Porcine satellite cells | Development of an efficient and innovative cultured meat production system through upregulation of signal transduction | Yan et al., 2022 |
Molding | Sodium alginate | Murine myoblast C2C12 cell line | Utility of a dual cross-linked alginate hydrogel system to support in vitromeat growth | Tahir and Floreani, 2022 |
3D printing | Salecan and κ-carrageenan | Mouse fibroblast cells | A new strategy for fabricating and optimizing polysaccharide-based hydrogel scaffolds | Qi et al., 2020 |
3D printing | Sol-gel transition and ionic gelation | Mouse C2C12 | Analyzing the adhesion of gellan gum and developing a new support accordingly | Koivisto et al., 2019 |
3D printing | Soy protein isolate | Primary bovine satellite cell | Great potential for research on cultivated meat through the use of peas, which have low allergenicity | Ianovici et al., 2022 |
Decellularization | Spinach | Primary bovine satellite cell | A cost-effective and environmentally friendly scaffold, potentially accelerating the development of laboratory-grown meat | Jones et al., 2021 |
Decellularization | Fresh whole jackfruit | Primary bovine satellite cell | Proposing a new closed bioreactor system for cellular agriculture products | Perreault et al., 2023 |
Decellularization | Grass blade | Murine C2C12 myoblasts | Presents the need for the development of inexpensive and sustainable support materials and structures | Allan et al., 2021 |
Freeze-drying | Gellan gum and guar gum | Mouse fibroblast cell (L929) | Development of a new scaffold that confirmed the cytotoxicity and cell adhesion of the scaffold | Anandan et al., 2019 |
Freeze-drying | Hydrolyzed collagen | - | Focus on using food-grade materials to ensure commercial availability of developed collagen ink formulations | Koranne et al., 2022 |
Freeze-drying | Collagen from turkey tendons | Skeletal muscle satellite cells | A major challenge for successful cultured meat production is the need for large quantities of skeletal muscle satellite cells | Andreassen et al., 2022 |
ESI, which is mainly used to manufacture fiber scaffolds, can extract fibers with a diameter of 1 to several tens of μms, and thus, can be used to create a fiber matrix, mold it to make MCs or manufacture various scaffolds (Pu et al., 2015). These nanofibers are produced by dry-spinning, which utilizes air or inert gas to evaporate residual solvents, and dry-jet wet spinning, which allows the polymer to orient and coalesce on the external surface before it hardens into shape. Wet-spinning, which solidifies, and melt-spinning, in which molten polymers are manufactured by exposing them to cooling air, are classified into various types depending on the material (Luo et al., 2012). The similar electro-spraying (E-SR) uses the same material as ESI. However, the material is emitted from the capillary itself rather than a nozzle during the spraying process. These two methods are similar in that they involve adding material to a capillary tube, a metal needle or spinneret, and a fiber collection device, but they are differentiated depending on the type of material.
These methods are also similar to the 3D printing method, which is used in two distinct ways: fused deposition modeling (FDM) and extrusion modeling (EM; Placone and Engler, 2018; Pu’ad et al., 2020). FDM uses two nozzles, one for the material used in manufacturing and the other for the material used to maintain the shape of the material. Therefore, two nozzles are used during production, and the shape-retaining material is later removed using a dedicated liquid (Ceretti et al., 2017). In contrast, EM uses a single material and is a method of developing a support by applying physical pressure. EM is divided into syringe-based extrusion, screw-based extrusion, and pneumatic extrusion (Guo et al., 2019), depending on the material it is manufactured from. The shape of the support produced differs depending on the density and physical properties, so it can be manufactured to reflect the researcher’s exact purpose. In particular, the difference between the two is that FDM is mainly used to develop a scaffold, and EM uses the cells themselves as ink, enabling the direct production of cultured meat.
Molding refers to a method of molding a scaffold polymer to which cells are attached into a mold designed according to the researcher’s intention. This includes molds formed by 3D printing (not a bioprinter), decellularization, and freeze-drying, in the same sense, MC is also used for this belongs (Ogawa et al., 2022). This scaffold has a relatively simple manufacturing method compared to other methods and is inexpensive because it can be used semi-permanently. Decellularization is a method in which cellulose-based cell walls are obtained using a chemical reaction, and nuclear material is removed from plant tissue (Toker-Bayraktar et al., 2023). This scaffold has a structure favorable for cell attachment and, like animal blood vessels, can promote cell growth because it is thin and has a large surface area (Walawalkar and Almelkar, 2021). In contrast, the freeze-drying method vaporizes water molecules to create a scaffold. Briefly, it is a method of mixing water and the solution used in the scaffold, molding it into the shape intended by the manufacturer, and then freeze-drying, which is a relatively simple process compared to other scaffolds (Chen et al., 2024). Cells attach and grow in the space created by vaporization and to freeze-drying is a widely used scaffold manufacturing method because mass production is possible.
Appearance Characteristics of Cultured Meat
The production of excellent scaffolds is a basic step in cultured meat, and further analysis is required to determine how similar cultured meat and edible meat actually are. When purchasing meat, the first factor consumers consider is the appearance of the meat itself, such as red color, bright color, and a harmonious proportion of fat (Lee et al., 2020; Fig. 3). However, unlike meat, cultured meat grows in a culture medium and is cultured in oxygen-blocked conditions, so it has a light color (Fraeye et al., 2020). Therefore, it is important to form myoglobin protein, which is a factor in the red color of meat, because it is not present in cultured meat (Suman and Joseph, 2013). Color changes are induced by manipulating the culture conditions to replace myoglobin, such as adding extracellular heme protein or adding additional iron to the culture medium (Post and Hocquette, 2017; Siddiqui et al., 2022). Research is also being conducted to replace the red color using additives that are natural colorants, such as beets, carrots, tomatoes, and paprika (Bohrer, 2019; Grispoldi et al., 2022). Other studies are underway to increase the expression of myoglobin by culturing muscle fibers under hypoxic conditions. However, further research is needed (Moritz et al., 2015). Scaffolds are essential for cultured meat, and since the amount of scaffold is greater than that of the edible meat produced, studies are also being conducted to dye and use the scaffold itself. Since the materials of most scaffolds are transparent or white, they are easy to stain with dyes, colorants, such as hematoxylin, rhodamine, beet extract, and natural polyphenols (Bezjak et al., 2023; Xiang et al., 2022), and have visual dispersion effects when cells attach, proliferate, and differentiate. Marbling, another exterior characteristic, is another important consideration of consumers when purchasing a product. According to research by Killinger et al. (2004), when purchasing beef, selecting the degree of marbling differed depending on the fat content that buyers wanted. Therefore, it seems worth researching the proliferation and differentiation of fat cells, as well as the production technology of cultured meat with a variety of fat contents rather than a single fat content. Since the fat content in cultured meat can be selected, the development of a scaffold that fat cells can easily attach to, such as polyunsaturated fat or omega-3, is also an area that needs to be continuously researched.
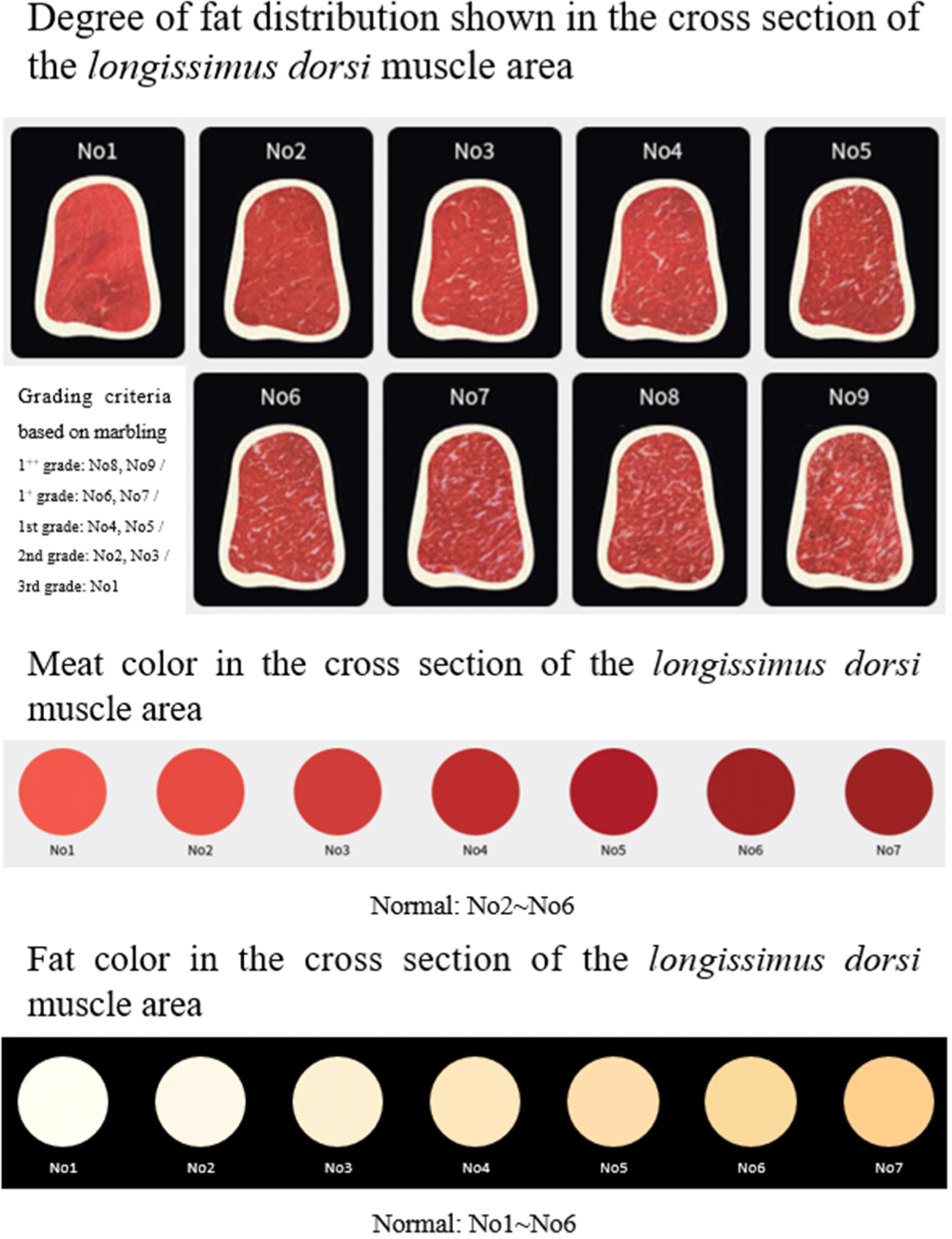
Flavor Characteristics of Cultured Meat
The factor that meat buyers consider most important next to appearance is flavor. Therefore, flavor resulting from volatile compounds generated from intramuscular fat is important. In general, most consumers have aversions to new things and tend to look for characteristics they are familiar with (Stallberg-White and Pliner, 1999). The aroma and taste of meat are generated by volatile substances produced by the reactions of non-volatile components induced by heat. Water-soluble compounds with low molecular weight and meat lipids have been reported to be important factors in the taste of cooked meat (Khan et al., 2015). Additionally, since amino acids, such as methionine and cysteine, are important factors in meat flavor, their inclusion means that the flavor can be similar to that of regular meat (Yang et al., 2022). Since the flavors generated by these compounds or lipid states are different, consumers’ preferences may also differ accordingly (Garmyn, 2020). Therefore, cells and scaffolds should be developed in the cultured meat market with flavors similar to or superior to those of meat (Lee et al., 2022). Since scent is mainly expressed by fat cells, it is also important to have fat cells with an attractive scent. Song et al. (2022) and others conducted a study aimed at improving flavor due to the proliferation of fat cells, reporting that a scaffold made from peanut protein promoted the proliferation of mesenchymal stem cells derived from pig fat. In addition, as previously mentioned, many different scaffolds can be used to culture meat, so it is important to select the appropriate scaffold components well (Post et al., 2020). According to reports that binding some flavor precursors of fat cells to a scaffold could promote cell differentiation and improve the taste of the final product, scaffolds are being developed using mushrooms that produce meat flavor or microbial flora (Yalman et al., 2023; Zhang et al., 2022). For cultured meat to have a flavor similar to that of edible meat, the combination of aromatic substances with a support and the development of a scaffold to which fat cells with the main flavor of meat adhere well must be continuously pursued (Fig. 4).
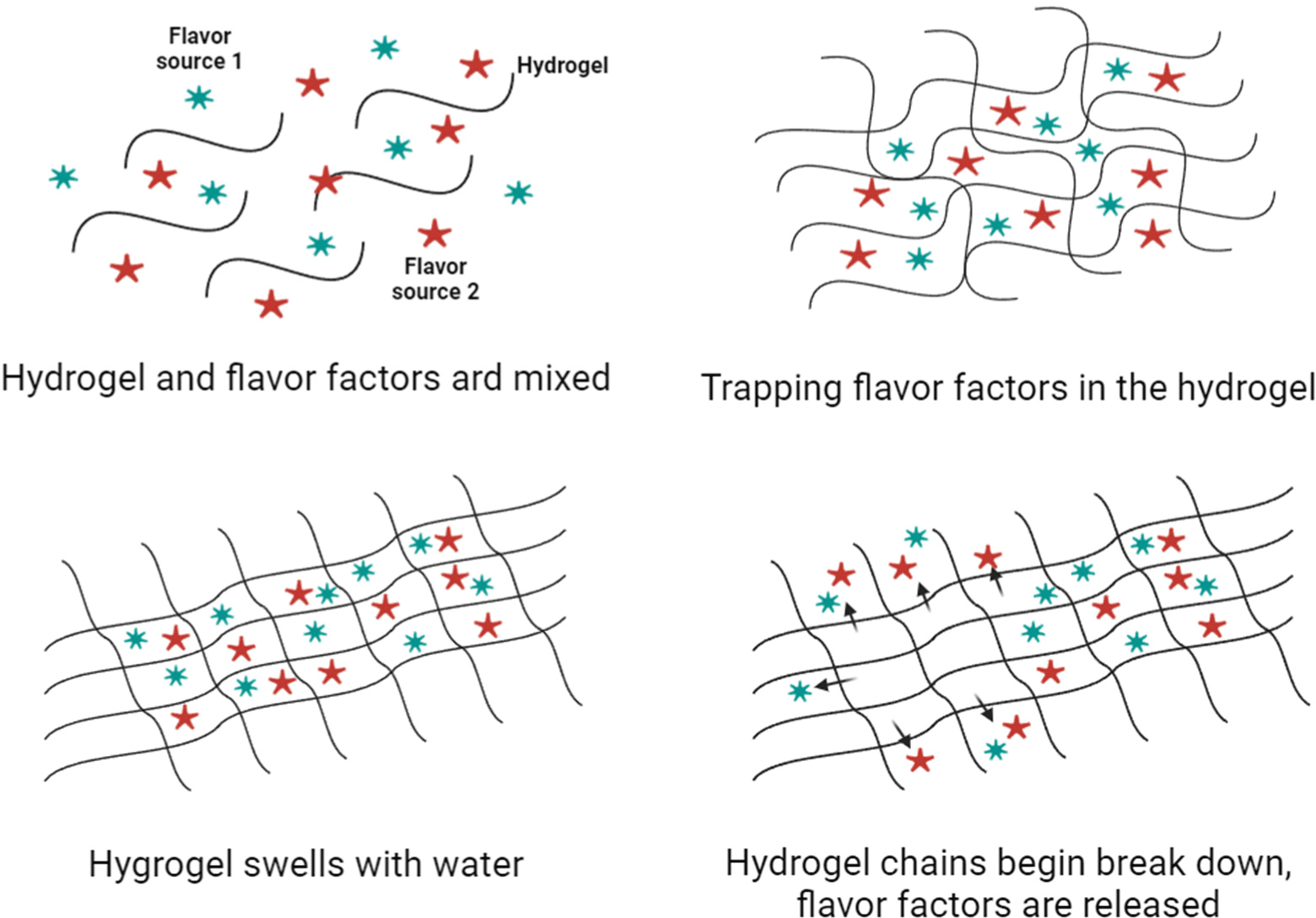
Textural Characteristics of Cultured Meat
The most influential factor in the production of cultured meat using scaffolds is the textural characteristics of tissues such as cells, scaffolds, and fat. In addition, the composition and shape of the tissue are important because these affect sensory factors, such as the texture and juiciness of meat (Martinez et al., 2023). The factor that has the most practical influence on the scaffold of cultured meat is the tissue, and as shown in Chapters 1 and 2, the method of manufacturing the scaffold varies depending on the material (Szymczyk-Ziółkowska et al., 2020). Most 3D scaffolds are manufactured using natural materials, such as animal collagen, chitosan, and vegetable cellulose, as they are often consumed as-is after producing cultured meat (Moslemy et al., 2023). In most cases, collagen is made into hydrogel or MC, and because it is already extracted from animal protein, this scaffold has a texture similar to meat (Chen et al., 2023). However, when used as a cultured meat scaffold, if the concentration is too high or the amount is too large, hardness increases, and the textural preference is lower than that of meat (Grønlien et al., 2019). de la Cruz Bosques et al. (2023) stated that when bovine pericardium is decellularized and used as a scaffold, large amounts of cultured meat can be produced at a low cost. Soybean, a vegetable protein, has been used as a textured vegetable protein to mimic meat, and many recent studies have shown that it can be used as a scaffold (Ben-Arye et al., 2020; Guan et al., 2023). However, in the case of plant proteins, there is no arginine-glycine-aspartic acid sequence that allows cells to attach, so coating with animal protein is necessary to enable the cells to attach (Lee et al., 2022). In addition, decellularized cell tissues were reported to require animal proteins, such as collagen, or plant proteins, such as alginate, to attach to cells (Brown et al., 2017). Vegetable protein itself has a lower hardness than regular meat and may have a slightly heterogeneous feeling, so additional research is needed. Alternatively, cells may be attached through electrostatic biocompatibility. This production method is known to have a texture more similar to real meat than the method described above (Ravishankar et al., 2019). Yen et al. (2023) and others reported that cultured meat produced with MC made of 2% chitosan and 0.2%–0.3% collagen showed low hardness, elasticity, and cohesion in the raw state but that these factors could be improved in the cooked state. Therefore, cultured meat should be produced by manufacturing scaffolds using appropriate concentrations and processing methods to improve appearance, flavor, and texture (Fig. 5).
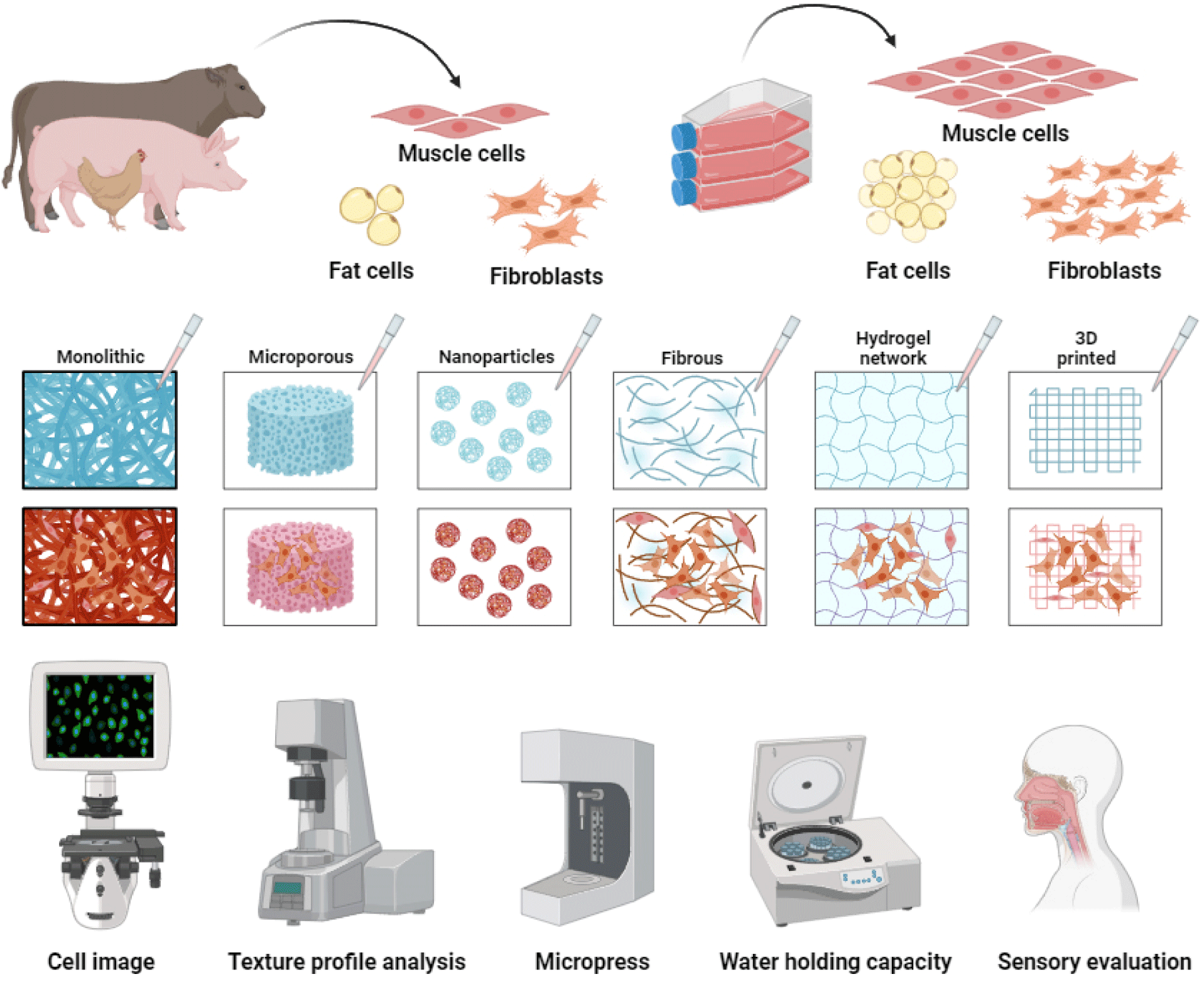
Conclusion
Cultured meat appears to be valuable as a future protein because it can solve various problems, such as food and environmental problems, not only in Korea but also around the world. Because the impact on the future protein market varies depending on the level and extent of current development, various efforts, such as symposiums and seminars on future proteins, are currently ongoing. Globally, the research and development of cultured meat continues to increase. Accordingly, Korea has set a goal to mass produce cultured meat, and many companies are starting up or collaborating to produce cultured meat. Among the various methods for mass production, scaffold technology, mostly manufactured from edible materials, is described in this manuscript. When culturing muscle cells using a scaffold, more cells can be attached and mass-produced compared to 2D. These scaffolds have various manufacturing methods (e.g., ESI, E-SR, 3D printing, molding, decellularization, and freeze drying) using animal, vegetable, and chemical materials. Fiber scaffolds, hydrogels, MC, and suspensions made through these manufacturing methods utilize the adhesion of cells to enable mass culture. However, if the cultured meat produced in this way is different from actual meat, it may not be preferred by consumers, so additional research is needed. First, when muscle cells proliferate and differentiate, they are submerged in a culture medium and grow in an anaerobic state, resulting in a lack of myoglobin, a pigment-protein. Therefore, the appearance (red, light, dark, etc.) can be supplemented using natural colorants or adding heme protein or iron to the scaffold. In addition, research on flavor and taste, such as producing scaffolds with strains that exhibit the flavor of meat or attaching precursors related to the flavor of the scaffold, is ongoing. Lastly, cultured meat manufactured using scaffolds is also related to texture because, like regular meat, the scaffold (tendon, etc.) affects the texture. Therefore, it is important to control the concentration and strength of the material and the thickness and hardness of the scaffold to achieve a texture similar to that of meat. Thus, since the use of a scaffold is inevitable for the mass production of cultured meat, research on scaffolds, as well as research to produce products that are similar to meat in external factors such as appearance, flavor, and texture while using the scaffold, need to continue.