Introduction
Hanwoo (HW), a representative beef cattle in Republic of Korea, is known for its high marbling and palatability. The name ‘Hanwoo’ consists of Han (meaning Korea) and Woo (meaning cattle) and refers to Korean cattle. The coat color of HW is generally brown. However, HW comprises four different breeds: Chickso (brindle with black and brown coat color), Heukwoo (coat color is black but occasionally mixed brown on the face and back), and Jeju black (BL; whole coat color is black; Lee et al., 2014). In the National Report on the State of Animal Genetic Resources from the Ministry for Food, Agriculture, Forestry and Fisheries (2004), cattle in the Republic of Korea comprised 67% brown cattle, 37% Holstein, and the rest included Chickso, Heukwoo, and BL. BL has been a natural monument 546 in the Republic of Korea after being formally registered with the FAO in this report. The population of BL was 1,087 in 2023, and the concerned body of Jeju Island has planned to increase this population to 2,000 by 2030. Overall, the BL breed still faces the threat of extinction, probably owing to the lack of consumer knowledge and need for this breed.
Studies on BL are scanty. Dadi et al. (2014) analyzed cattle genes distributed in East Asia and reported specific mitochondrial DNA sequences of BL, brown HW, and brindle HW. Alam et al. (2021) reported lower genetic heterozygosity in BL than in brown HW and Holstein in the Republic of Korea. Han et al. (2011) reported that the melanocartine-1 receptor alleles ED, E+, and e are found in BL and that these traits are directly related to the coat color phenotype of HW, BL, and their crossbreeds. Although BL exhibits lower average daily gain, live weight, and carcass weight, a higher feed efficiency has been observed than that in Charolles and the crossbreeds of BL and Charolles raised for 18 months (Oh et al., 2008). Lee et al. (2007) observed that pure BL shows a low average daily gain until 16 months, which increases during the period of 17–24 months, when compared with the those of other breeds. In the same study, although the carcass weight of pure BL was low, no significant difference in the carcass yield was observed. Moon et al. (2012) have compared same-quality grade brown HW, BL, and imported Australian beef and found no significant differences in the proximate composition; however, higher CIE a* and lower melting point were observed for BL than for the other two breeds. Additionally, the fatty acid composition of BL showed low levels of saturated and high levels of unsaturated fatty acids (Hoa et al., 2024; Lee et al., 2019; Moon et al., 2012; Song and Hwang, 2023; Tuell et al., 2022).
BL is a traditional cattle breed raised on Jeju Island in the Republic of Korea, and is served at national events such as the birthday of the King or memorial dates of the Royal Family (Lee et al., 2019). Although some studies have focused on the characteristics of BL, these were restricted to only genetics; additionally, meat quality has been evaluated only in loins. Therefore, this study aimed to evaluate the meat quality and fiber characteristics of longissimus lumborum, psoas major, and semimembranosus muscles from BL, brown HW, and their crossbreeds.
Materials and Methods
A total of 36 steers (12 steers per breed) of HW (31.8±1.0 months of age, 620.3±6.5 kg carcass weight), BL (33.2±2.4 months of age, 583.3±17.9 kg carcass weight), and crossbreds (BH; 31.3±1.4 months of age, 720.1±23.8 kg carcass weight), which were raised under the same conditions and fattened on a cattle farm located in Jeju Island, were randomly selected and transported to the abattoir of the National Institute of Animal Science (NIAS), Wanju, Republic of Korea. Feeding was conducted according to the Korean Feeding Standard for Hanwoo (NIAS, 2020). Livestock transport, preslaughter management, and slaughter were conducted in accordance with the Livestock Products Sanitation Control Act. Beef carcasses were chilled at 1°C for 48 h after slaughter; then, three muscles (M. longissimus lumborum, M. psoas major, and M. semimembranosus) were removed from the left sides of the carcasses. For immunohistochemistry, muscle tissues (1.5× 1.5×2.0 cm) were immediately frozen in 2-methylbutane chilled with liquid nitrogen and stored at –80°C until analysis. The remaining muscle samples were used for analyzing physicochemical properties (proximate composition, pH, color, cooking loss, and shear force).
Moisture, crude protein, and crude ash contents were analyzed according to the guidelines of the Association of Official Agricultural Chemists (AOAC, 2000), with some modifications. Briefly, moisture content was determined by drying 10 g sample for 24 h in a dry oven at 105°C and expressed as the percentage of total weight by calculating the difference in weight before and after drying. To determine the crude protein content, 1.0 g sample was digested with sulfuric acid and distilled ammonia into boric acid following the Kjeldahl method (AOAC, 2000). Borate ions were titrated with hydrochloric acid, and crude protein content was calculated from the total nitrogen content. Crude ash contents were calculated by measuring the weights of samples before and after burning the muscles at 200°C, 400°C, 600°C, and 800°C for 2 h after pre-drying at 100°C for 2 h; the ash content was expressed as a percentage. Crude fat content was measured as described by Folch et al. (1957). Briefly, approximately 5.0 g sample was homogenized in 30 mL extraction buffer (chloroform:methanol at a 2:1 v/v ratio) and filtered through a Whatman No. 1 filter paper (Merck, Darmstadt, Germany). The filtrate was mixed with 0.88% sodium chloride and incubated at room temperature for 2 h. Once the upper layer was removed using an evaporator, 10 mL of the lower layer was dried in pre-weighted aluminum dish to obtain crude fat. Crude fat content was expressed as the percentage of the sample. The proximate composition was obtained from triplicate measurements for each sample.
For measuring the pH, 3.0 g sample was homogenized with 27 mL deionized water, and the pH was measured using a pH meter (S220, Mettler Toledo, Greifensee, Switzerland) calibrated with standard buffer solutions (pH 4.01, 7.00, and 9.21). The pH values were determined from three replicates of each sample.
Meat color intensity on the sample surface was measured after exposure to air for 20 min at room temperature. A colorimeter (CR-400, Konica Minolta, Tokyo, Japan) was set up with a D65 light source, an 8° illumination angle, and an 8-mm measuring aperture after calibration with a standard plate (Y, 93.5; x, 0.3132; y, 0.3198). Color values for CIE L*, CIE a*, and CIE b* were represented according to the Commission Internationale de l’ Eclairag System (CIE, 1978). Meat color intensity was measured in five different regions of each sample.
Approximately 25 g sample was cooked in a water bath (WB-22, Daihan Scientific, Wonjoo, Korea) at 75°C to measure cooking loss. Samples for which the internal temperature reached 70°C were cooled to room temperature, and their weights were measured. The difference in weight before and after cooking was monitored. Cooking loss was calculated as the percentage of initial weight from triplicate measurements.
Cooked samples were used for measuring the shear force. Three cores (1.0 cm diameter) of each sample were obtained by cutting parallel to the orientation of muscle fiber. Shear force (N/cm2) was measured using a texture analyzer (TA1, AMETEK, Largo, FL, USA) equipped with a Warner-Bratzler shear blade at 3.0 mm/s and 50 kgf of load cell.
Immunohistochemistry was conducted to analyze muscle fiber characteristics according to the method described by Song et al. (2020), with some modifications. Briefly, cross-sections (10 μm thickness) obtained from each frozen sample were blocked with 10% normal goat serum (Cell Signalling Technology, Danvers, MA, USA) and incubated with primary antibodies (BA-F8, SC-71, and 6H1; DSHB, Iowa City, IA, USA). The cross-sections were then incubated with secondary antibodies (anti-IgG and anti-IgM) conjugated with Alexa Fluor 405, 488, or 594 (Thermo Fisher Scientific, Waltham, MA, USA). Images of three different regions of each section were captured using a microscope (EVOS M5000, Thermo Fisher Scientific). Muscle fiber characteristics (cross-sectional area, μm2; relative fiber area, %; fiber density, number/mm2) were evaluated using approximately 800 muscle fibers of each section. Image Pro Plus (Media Cybernetics, Rockville, MD, USA) was used to analyze each image.
All data are presented as mean±SE. Statistical analyses were conducted using SAS software v.9.4 (SAS Institute, Cary, NC, USA). Differences in physicochemical and muscle fiber characteristics within the same muscle among breeds were evaluated using a one-way analysis of variance (ANOVA), followed by Duncan’s multiple range post-hoc test. Pearson’s correlation coefficients between physicochemical properties and muscle fiber characteristics were analyzed to describe the relationship between muscle fiber characteristics and physicochemical properties, regardless of muscle type. Statistical significance was set at p<0.05.
Results
The proximate composition and meat quality traits of BL, brown HW, and BH are shown in Figs. 1, 2, and 3. All characteristics, except the pH, were significantly different in M. longissimus lumborum. BL had relatively high moisture content and low crude fat and protein contents (p<0.05; Figs. 1A, B, and C). BH showed intermediate traits, in which the moisture content was higher than that of HW, and a moderate level of crude fat content between those of HW and BL (p<0.05). The crude ash content of BH was higher than that of HW (p<0.05; Fig. 1D), but no significant difference was observed when compared with that of BL (p>0.05). HW showed higher CIE L*, CIE a*, and CIE b* than did BH (p<0.05; Figs. 1F, G, and H). BL showed lower CIE L* than did HW and higher CIE a* and CIE b* than did BH (p<0.05). The cooking loss in BL was significantly lower than in HW and higher than in BH (p<0.05; Fig. 1I). The shear force was highest for BL, followed by that for BH and HW (p<0.05; Fig. 1J).
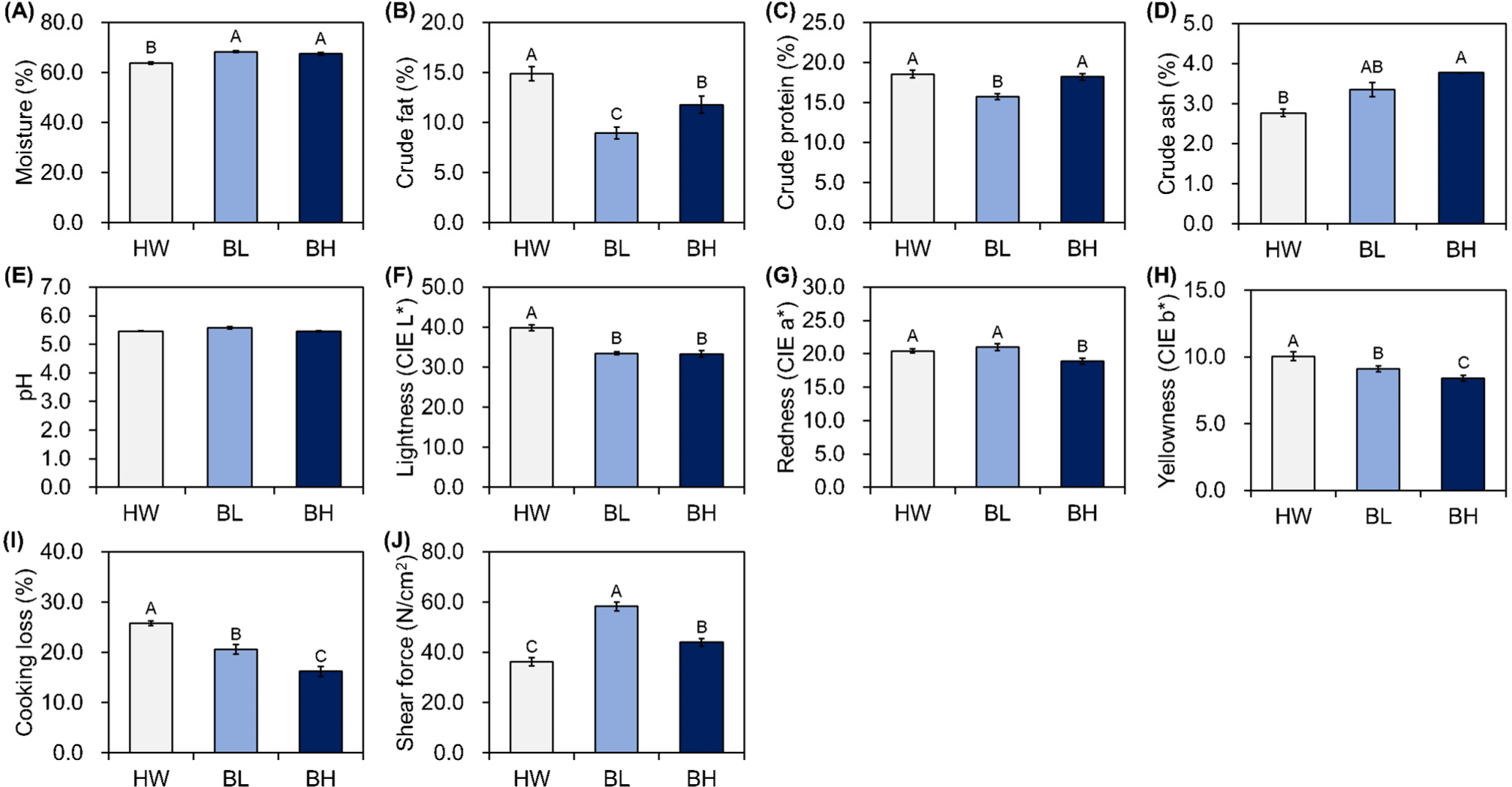
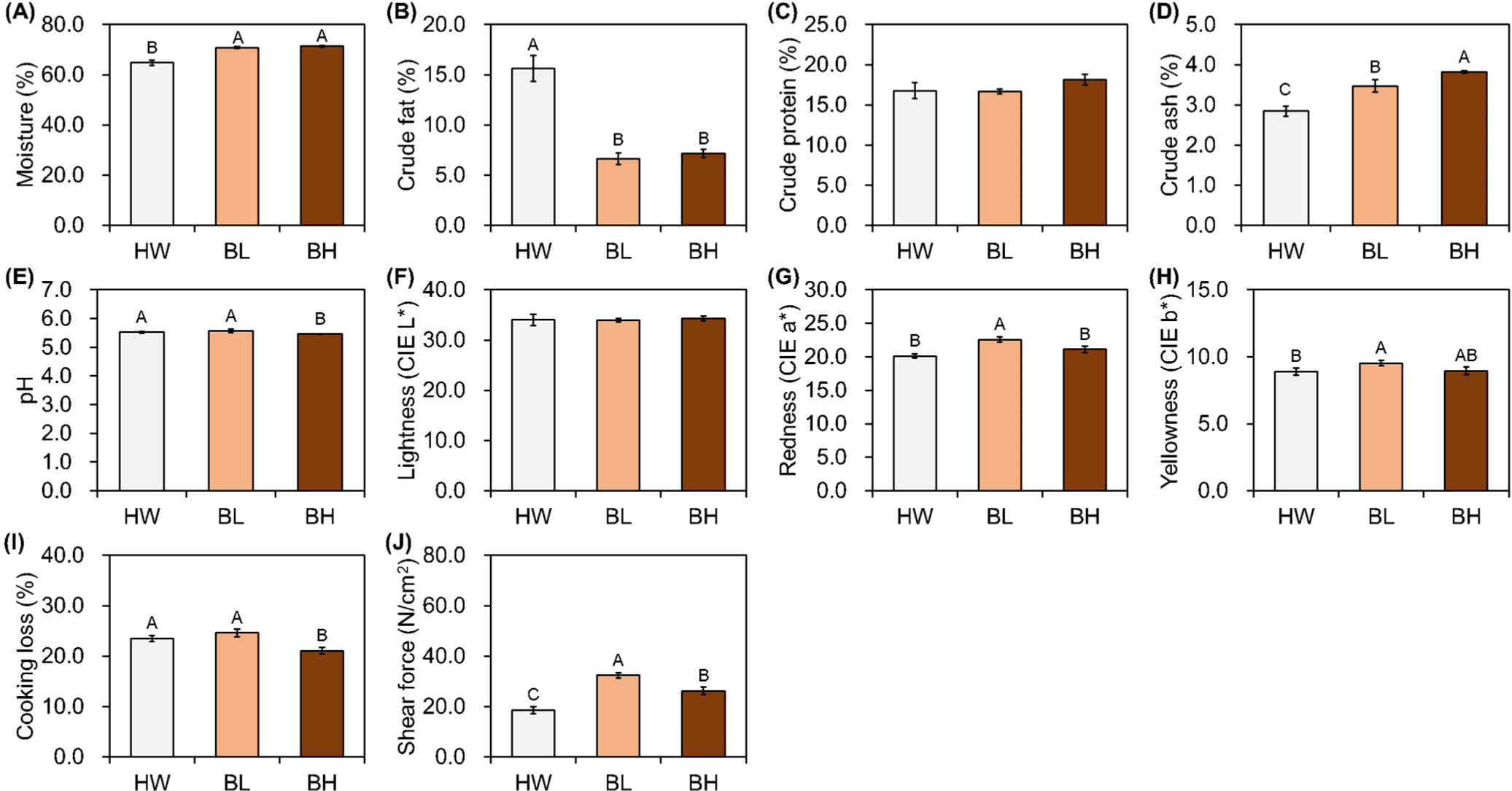
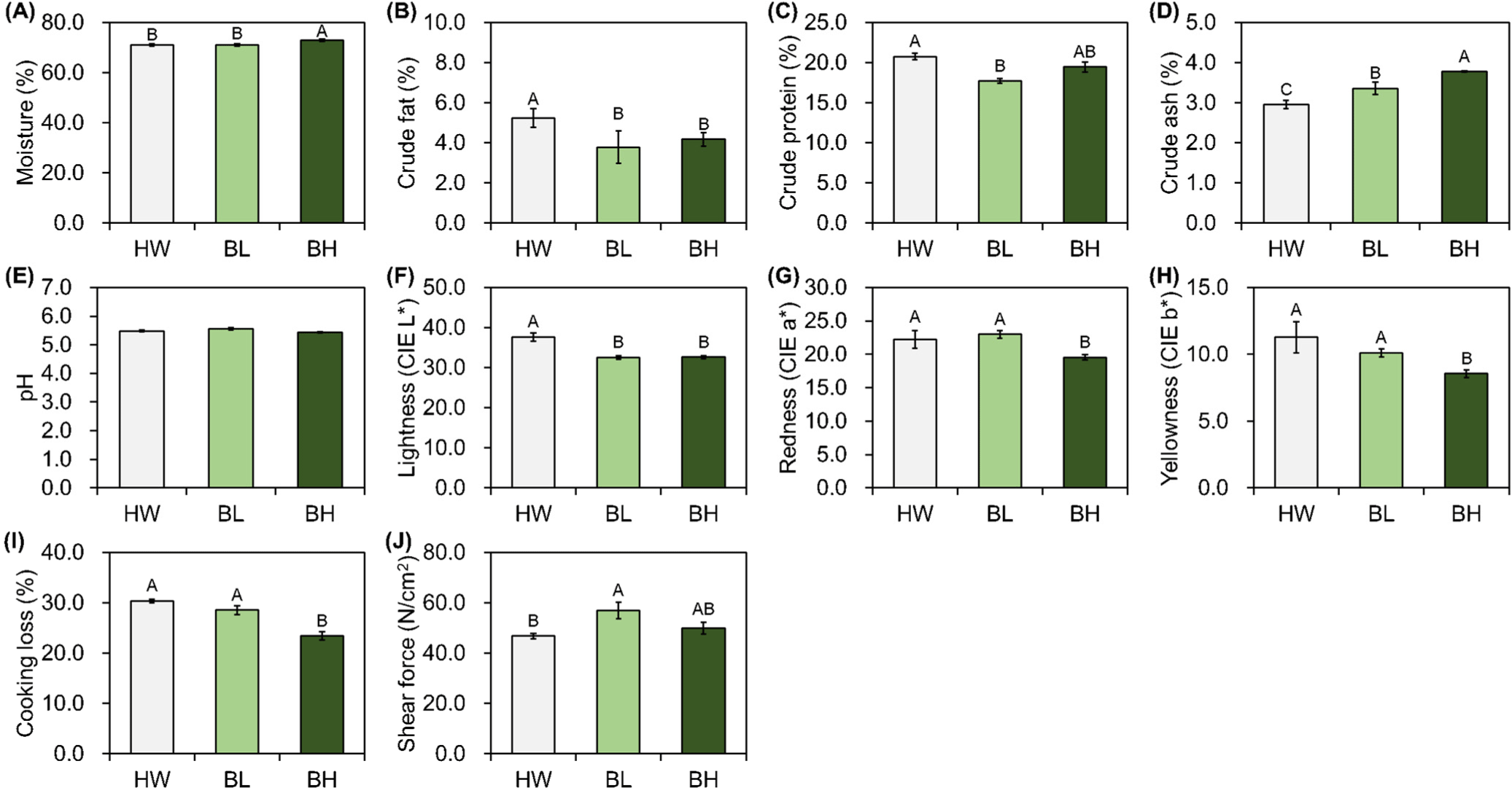
Most traits, except crude protein content and CIE L*, showed significant differences in M. psoas major among breeds. BL had higher moisture and crude ash contents and a lower crude fat content than those of HW (p<0.05; Figs. 2A, B, and D). The proximate composition of the psoas major muscle of BH was similar to that of BL, but the crude ash content was relatively higher in BL (p<0.05; Figs. 2A, B, and D). The pH value for BH was significantly lower than the values for HW and BL (p<0.05; Fig. 2E). BL showed greater CIE a* than did HW and BH; CIE b* was significantly different between BL and HW (p<0.05; Figs. 2G and H). Cooking loss in BH was significantly lower than that in BL and HW (p<0.05; Fig. 2I). The highest and lowest shear force values were observed in BL and HW, respectively (p<0.05; Fig. 2J).
Significant differences in all meat quality traits, except the pH, were observed in M. semimembranosus (p<0.05) among different breeds. The results of proximate composition showed significantly high moisture and crude ash contents in BH, and crude fat and crude protein content in HW (p<0.05; Figs. 3A, B, C, and D). The color of M. semimembranosus was similar to that of M. longissimus lumborum; HW showed higher CIE L* and lower CIE a* and CIE b* than did BL and BH (p<0.05; Figs. 3F, G, and H). BH showed lower cooking loss than did BL and HW (p<0.05; Fig. 3I), and the values for BL and HW were not significantly different (p>0.05). Significantly higher shear force was observed for BL than for HW (p<0.05; Fig. 3J), and no significant difference was observed in BH (p>0.05).
Representative immunofluorescent images of BL, HW, and BH for the analysis of muscle fiber characteristics are shown in Fig. 4. The cross-sectional area, relative area ratio, and density of muscle fibers were analyzed using those images; the results are shown in Fig. 5. Regarding the cross-sectional area of M. longissimus lumborum, the area was significantly higher in BL than in the other breeds, regardless of the muscle fiber type (p<0.05; Fig. 4A). Specifically, muscle fiber types IIA and IIAX were not significantly different between BL and BH (p>0.05); however, fiber types I and IIX and mean cross-sectional area were significantly higher in BL than in BH (p<0.05). Type IIX fibers appeared to be largest in BL and BH, whereas type IIAX was largest in HW (p<0.05). No significant difference was observed for type IIX (p>0.05; Fig. 5A); BL had a higher area ratio of type I, and a lower area ratio of type IIA and IIAX than the other breeds (p<0.05; Fig. 5B). Regardless of breed, the area ratio of IIX was highest and that of IIAX was lowest (p<0.05). No significant difference was observed in type I among the breeds (p>0.05; Fig. 5C). Relatively high fiber density was noticed for the other and total fiber types for M. longissimus lumborum in HW, whereas the density of type IIA fiber and total density in BL was lower than those in BH (p<0.05).
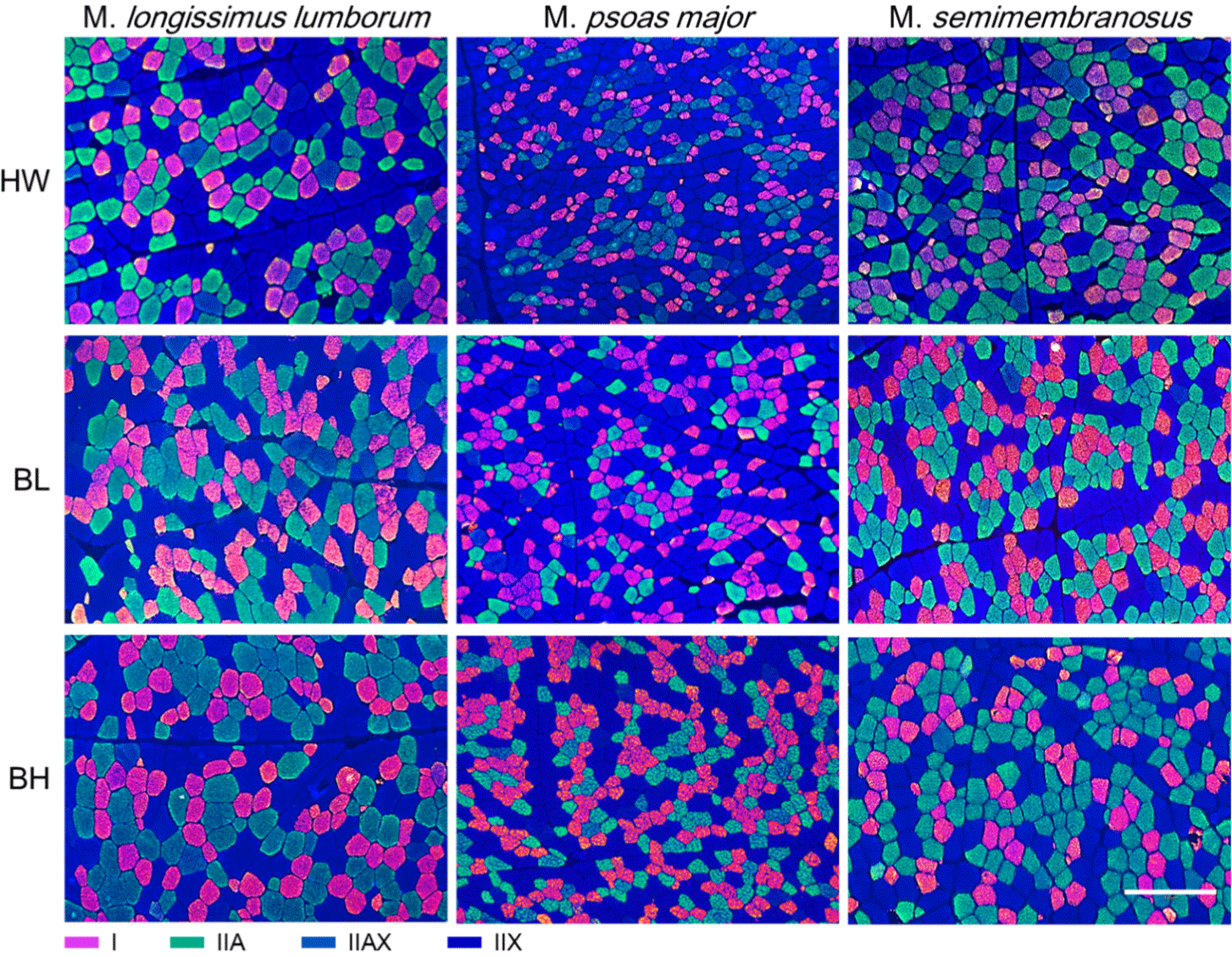
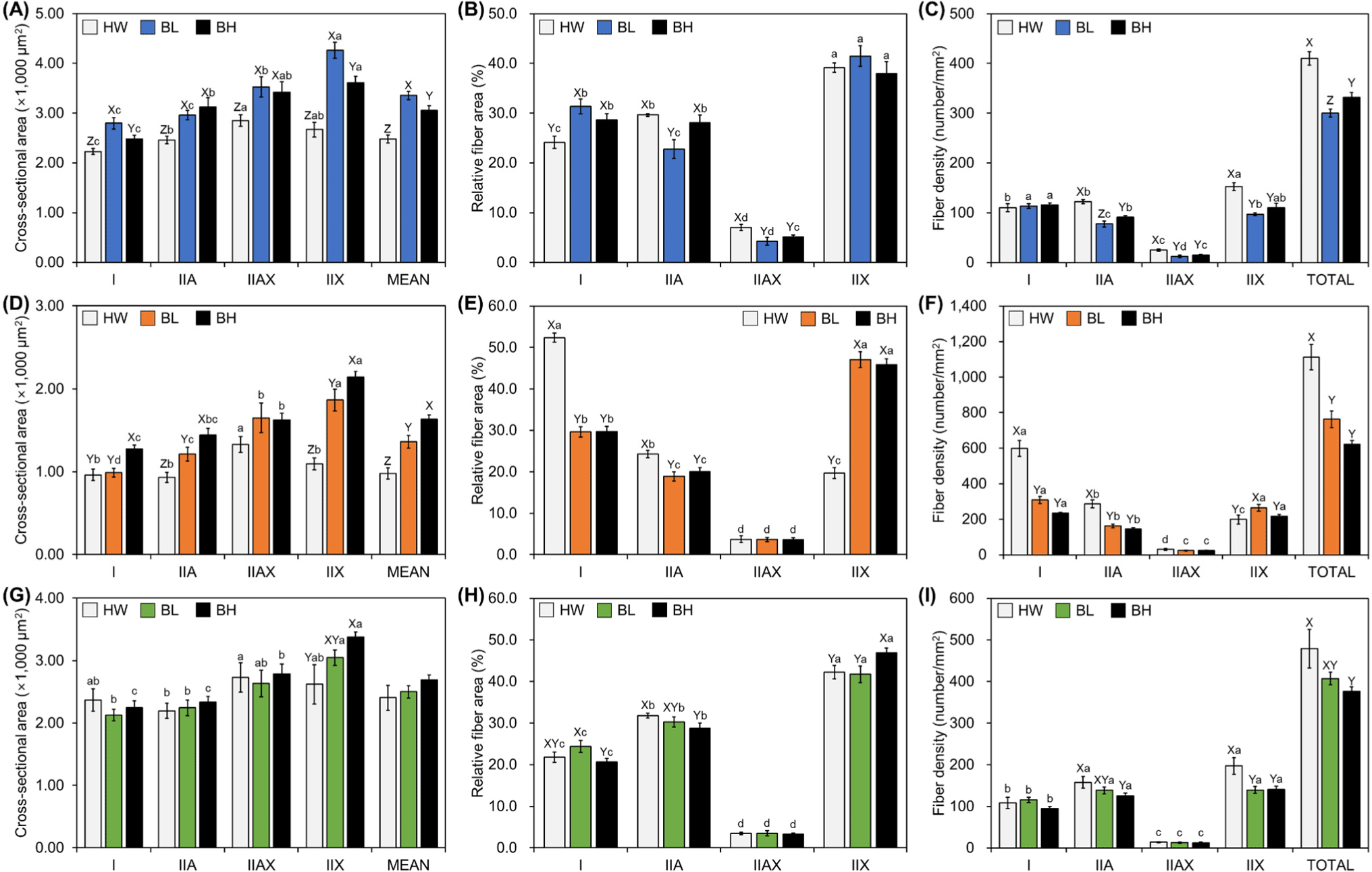
The fiber characteristics of M. psoas major of the three different breeds are shown in Figs. 5D, E, and F. The cross-sectional area of BH was largest among all types, except for type IIAX and the mean area (p<0.05). BL had significantly larger areas of types IIA, IIAX, and IIX than did HW (p<0.05). Within breeds, BL and BH had the largest areas of type IIX, whereas type IIAX was largest in HW (p<0.05). Regarding the relative area ratio, the highest ratio over 50% for type I was found in HW, and the area ratio of IIA was significantly high in HW (p<0.05). No significant difference in type IIAX among the breeds (p>0.05) was observed, but BL and HW showed a significantly high area ratio of type IIX (p<0.05). HW had the highest area ratio of type I, followed by that of IIA, IIX, and IIAX; BL and BH showed the highest area ratio of IIX, followed by that of I, IIA, and IIAX (p<0.05). The highest densities of type I and IIA, and the highest total density were noticed in HW; type IIX in BL was significantly higher than that in the other breeds (p<0.05). No significant difference was noticed for type IIAX among the different breeds (p>0.05). Within the breeds, the density of type I was highest across all breeds, followed by that of IIX, IIA, and IIAX in BL and BH, and IIA, IIX, and IIAX in HW (p<0.05).
The muscle fiber characteristics of M. semimembranosus are shown in Figs. 5G, H, and I. Unlike that in other muscles, a significant difference in cross-sectional area was observed only for type IIX fiber (p<0.05). Within the breeds, muscle fiber type IIX was largest in BL and BH, and the cross-sectional area of type IIAX was higher than that of type IIA in HW (p<0.05). The relative area ratio of M. semimembranosus was significantly higher for types I and IIA in HW and BL than in BH and that for IIX was higher in BH than in the other breeds (p<0.05). A comparison of muscle fiber types within the same breed revealed high-to-low ratios in the order of type IIX, IIA, I, and IIAX, regardless of breed (p<0.05). Significant differences in fiber density were noticed for types IIA, IIX, and total density; the density was highest in HW (p<0.05). However, no significant differences were observed in type IIA and total density compared with those in BL (p>0.05). Among the types within the same breed, all breeds showed the same order of trend: types IIA, IIX, I, and IIAX (p<0.05).
The correlation coefficients between the muscle fiber characteristics and physicochemical properties are shown in Table 1. The cross-sectional area of each fiber was not significantly correlated with moisture and crude protein contents (p>0.05), while that of type IIX showed negative and positive correlations with crude fat and crude ash contents, respectively (p<0.05). CIE a* showed a correlation with the cross-sectional area, except in type IIAX fiber (p<0.05), while CIE L* and CIE b* exhibited no significant correlations (p>0.05). The cross-sectional area of type IIX showed a significant correlation with cooking loss (p<0.05). Shear force exhibited a positive correlation with each muscle fiber type (p<0.05). Relative fiber area of type I exhibited negative correlations with moisture, crude protein, crude ash contents, and shear force, while it showed a positive correlation with crude fat content (p<0.05). The relative area of type IIA was positively correlated with crude protein content, CIE b*, cooking loss, and shear force (p<0.05). Relative fiber area of type IIAX exhibited a negative correlation with moisture contents; however, it was positively correlated with crude fat content and CIE L* (p<0.05). The relative area of type IIX exhibited positive correlations with moisture, crude ash, and shear force but a negative correlation with crude fat content (p<0.05). Correlation of type I fiber density with physicochemical properties corresponded to that of relative type I fiber area (p<0.05). Proximate composition showed significant correlations with types IIA, IIAX, and total fiber density (p<0.05). CIE a*, CIE b*, and cooking loss showed positive correlations with types IIX and IIA (p<0.05). Shear force showed a negative correlation with all muscle fiber density, including total fiber density (p<0.05). Among the physicochemical properties, only the pH did not show any significant correlation with muscle fiber characteristics (p>0.05).
Discussion
In the present study, BL and BH had relatively high moisture contents and relatively low crude fat and protein contents in the three different muscles. In contrast, HW had a relatively low moisture content and relatively high crude fat and protein contents. BH had a relatively high crude ash content, regardless of the muscle type. The quality grade of beef is highly dependent on the intramuscular fat contents owing to the preference for grilling beef as thin slices in the Republic of Korea; the general intramuscular fat content of HW is 6.60%–23.85%. In the present study, the quality grade of HW seemed to be 1, and that of BH and BL between 1–2 (Gajaweera et al., 2020; Jo et al., 2012; Lee et al., 2015). The intramuscular fat content affects eating quality, particularly tenderness and flavor (Gotoh et al., 2018; Wheeler et al., 1994). The factors affecting the intramuscular fat contents of meat vary; nevertheless, consumer demands can be met by increasing the intramuscular fat content through breeding or feeding systems, and likewise, HW was originally used for farming to meet such demands (Avilés et al., 2015; Lee et al., 2014; Miller et al., 1987; Park et al., 2018). The pH significantly affects muscle fiber characteristics, meat color, and water-holding capacity (Ertbjerg and Puolanne, 2017; Hopkins et al., 2014; Ryu and Kim, 2006; Węglarz, 2010); however, it did not significantly affect other meat quality traits in the present study. The results of meat color showed higher CIE L*, CIE a*, and CIE b* in the M. longissimus lumborum and M. semimembranosus of HW than in those of others. Wulf and Wise (1999) reported that color positively correlates with lean maturity and marbling score; and another study has reported significantly low CIE a* of M. longissimus thoracis with low intramuscular fat in HW (Kim and Lee, 2003). However, a contrasting result for M. psoas major indicated that intramuscular fat did not affect meat color in the present study. Cho et al. (2010) and Jo et al. (2012) reported the lowest CIE a* in quality grade 1 in HW, which increased following an increase in the intramuscular fat content. Furthermore, Lee et al. (2012) have reported a significant difference in CIE a* in the intramuscular fat, ranging between 13.12%–18.69 %, as analyzed from the HW marbling score. M. psoas major used in the present study had higher intramuscular fat and lower moisture contents. These findings indicate that intramuscular fat affects meat color, and the extent depends on the fat content. In all the three muscles, cooking loss was higher in BH than in the other breeds, which is in line the results of a previous study that analyzed proximate composition by doneness, wherein the degree of change in crude fat and moisture contents was higher than that of crude protein and ash contents (Smith et al., 2011). Regardless of the muscle types, BL showed the highest and HW showed the lowest shear force values, which is in agreement with the findings of Wheeler et al. (1994), who reported that an increase in intramuscular fat content results in a reduction in the shear force value. However, shear force does not affect all traits of meat, and additional analyses, such as texture profile analysis or sensory evaluation, are necessary (Caine et al., 2003).
The aforementioned meat quality traits are dependent on individual trait and muscle fiber characteristics. The trends such as large cross-sectional area of glycolytic muscle fibers, small oxidative fibers, and intermediate fibers are intermediate have been reported previously (Maltin et al., 1998; Spindler et al., 1980; Xie et al., 2012). Regarding significant differences in the cross-sectional area, those of HW were smaller than those of the other breeds. No significant difference was found in the cross-sectional area of M. semimembranosus between BL and BH, and M. longissimus lumborum in BL and M. psoas major in BH had larger cross-sectional areas than those of the other breeds. Although the carcass weight of BL was lowest, M. longissimus lumborum in BL and M. psoas major in BH exhibited largest cross-sectional area than those of other breeds, while the cross-sectional area of M. semimembranosus was not significantly different between BL and BH. These results are in accordance with previous findings showing a positive correlation with intramuscular fat and a negative correlation with CIE L* of the cross-sectional area of types I and IIA (Wegner et al., 2000). However, no significant correlation was observed in the present study. Similarly, a previous study has reported that relatively small fiber size and increased number of oxidative fibers promote metabolism, resulting in increased fat accumulation owing to energy supplementation, thereby affecting meat quality (Schiaffino and Reggiani, 1996). Although no significant correlation was observed between crude fat content and oxidative fibers, type IIX showed a negative correlation with crude fat content. Probably the increasing size resulted in increased shear force (Joo et al., 2017), while the diameter did not (Hwang et al., 2010; Ozawa et al., 2000).
Generally, the muscle fiber density of HW was higher, while the cross-sectional area was similar to or lower than those of the other breeds in the present study. This implies that the muscle fiber number in BL and BH is less than that in HW, and hyperplasia of HW or hypertrophy of BL and BH can be considered, indicating that the characteristics of each cell from the three breeds are different (Picard and Gagaoua, 2020). While only a few studies have compared muscle fiber characteristics of HW with those of other breeds, Kim et al. (2021) have reported similar results, showing that HW consists of an increased number of muscle fibers compared to that in Holstein raised for meat in the Republic of Korea; additionally, a relatively low shear force has been observed. Xie et al. (2012) have reported different muscle fiber characteristics among five different breeds raised in the same feeding system. Johnston et al. (1975) and Spindler et al. (1980) have observed different muscle fiber characteristics among breeds. Generally, the cross-sectional area of HW is smaller than that of the other breeds, whereas the cross-sectional areas of BL and BH are larger than the area of HW.
Muscle fiber composition, representing muscle characteristics, is affected by breed, similar to that in other species (Park et al., 2022; Picard and Gagaoua, 2020). The present study reported that each muscle has a different muscle-fiber composition. Unexpectedly, the compositions of types I and IIX did not vary much from those of other muscles and were found to be similar to those of M. psoas major of BL and BH. Some differences were observed between the muscles in terms of muscle fiber composition. HW showed results similar to those of previous studies (Hwang et al., 2010; Kim et al., 2016). Type IIX fibers are positively correlated with the shear force. A relatively low shear force value was observed for HW, which had a relatively low type IIX composition in the present study. A high number of glycolytic fibers results in a reduction in the pH via accumulation of lactic acid during glycolysis in the early postmortem period; however, this does not fully harmonize with the results of the present study (Yu et al., 2019). The high content of oxidative fibers (types I and IIA) results in an increase in CIE a* owing to their metabolic traits (Jeong et al., 2009; Lefaucheur, 2010); however, the present study did not show similar results, which may be due to the low fat content. Muscle fiber composition exhibited changes in proximate composition in terms of fat accumulation, as previously described by Schiaffino and Reggiani (1996). Moreover, a high ratio of type IIX fiber resulted in a high shear force, as also previously described by Hwang et al. (2010). However, whether fat contents, type IIX characteristics, or other factors cause this phenomenon should be clarified.
Conclusion
The meat quality traits and muscle fiber characteristics of three different muscles (M. longissimus lumborum, M. psoas major, and M. semimembranosus) from three different breeds (BL, HW, and BH) were evaluated. BL showed lower intramuscular fat content and higher CIE L*, CIE a*, CIE b*, cooking loss, and shear force than the other breeds. The BH cattle had intermediate characteristics between those of BL and HW cattle. HW had smaller muscle fiber size and higher muscle fiber density than did the other breeds. In conclusion, differences in physicochemical and muscle fiber characteristics were confirmed, regardless of the type of muscle, among three breeds of Korean native cattle.