Introduction
Lymphoid organs include primary organs, such as the bone marrow and thymus, where immune cells are produced or transformed into functional cells. Additionally, secondary organs such as the mucosa-associated lymphoid tissue, lymph nodes, and spleen are sites where immune responses occur (Tabilas et al., 2023). The lymphatic tissue within the nodes consists mainly of myeloid cells, such as macrophages and dendritic cells (DCs). In contrast, B and T cells interact with antigen-presenting cells and migrate to separate areas where clonal expansion occurs. Analyzing the distribution and changes of immune cells in different primary and secondary immune organs is a valuable tool for investigating the immune response to infection (von Andrian and Mempel, 2003).
Atopic dermatitis (AD) is a common inflammatory skin disease that affects ~20% of children and ~3% of adults (Nutten, 2015). It is also a highly heterogeneous disease with a multifactorial etiology that includes genetic, environmental influences, and microbiota composition (McCoy and Köller, 2015). AD is initiated when epithelial cells such as keratinocytes are exposed to allergens, which causes them to release cytokines [(e.g., thymic stromal lymphopoietin (TSLP)] to activate Langerhans cells (Fania et al., 2022). Langerhans cells play a central role in activating naive helper T cells, which then differentiate into Th2 and Th17 cells (Akdis et al., 2020). Th2 cells produce cytokines such as IL-4 and suppress FLG expression, leading to symptoms like barrier dysfunction, impaired keratinocyte differentiation, and itching (Haddad et al., 2022). On the other hand, Th17 cells produce IL-17F, IL-17, IL-21, and IL-22, which are necessary for eliminating pathogens during host defense reactions (Sugaya, 2020). When peripheral tissues like muscle tissue, subcutaneous adipose tissue, heart tissue, and lung tissue are exposed to allergens, Treg cells inhibit the migration of Th1, Th2, and Th17 cells, suppress the activity of DCs, mast cells, eosinophils, and basophils, and limit IgE production by B cells. Depletion of Treg cells results in the worsening of skin inflammation and elevated serum IgE levels and Th2 cytokines (Fyhrquist et al., 2012). Gal-9 suppresses excessive Th2 responses and promotes Treg cell differentiation, inhibiting acute allergic reactions and mast cell degranulation (Purushothaman et al., 2018).
The compound 2,4-dinitrochlorobenzene (DNCB) is known for causing contact sensitization and forming multiple haptens with intracellular and extracellular proteins in the skin (Pickard et al., 2007). Repeated DNCB irritation on murine skin can be divided into two phases: a sensitization phase, which is the first contact with the hapten, and a challenge phase, which is the second hapten encounter (Wang et al., 2022). Probiotics are live microorganisms that have health-promoting effects when consumed sufficiently and continuously (Hill et al., 2014). In addition, probiotics regulate the balance of intestinal microflora, modulate the host’s immune response, and can be used to treat various skin disorders, such as AD (Lee et al., 2023; Plaza-Diaz et al., 2019). On the other hand, postbiotics consist of heat-killed bacteria, purified microbial components, and cell-free supernatants, and they have beneficial properties for safe pharmaceutical applications. They ensure safety and stability while maintaining the beneficial properties of probiotics (Taverniti and Guglielmetti, 2011; Vinderola et al., 2023).
Bifidobacterium are among the first bacteria to colonize the fetal intestine, making up about 90% of the intestinal bacteria in infants (Collado et al., 2010). Bifidobacterium have specific immunostimulatory properties that influence the Th1/Th2 balance, and these properties are partially attributed to the presence of unmethylated CpG motifs. Compared to Lactobacillus, Bifidobacterium have higher GC content (60.1% vs. 46.61%) and more CpG motifs (Kant et al., 2014; Ménard et al., 2010). Since discovering that Mycobacterium bovis BCG DNA has an anti-cancer effect by increasing type I interferon (IFN) production and natural killer cells, various cytosine-phosphate-guanine oligodeoxynucleotides (CpG ODNs) have been synthesized and used (Tokunaga et al., 1984). CpG ODN is one of the most promising adjuvants as a Toll-like-receptor 9 (TLR9) agonist. After uptake by DCs, it binds to the integral membrane receptor TLR9 of the endosomes and endoplasmic reticulum. Activation of the CpG-TLR9 signaling pathway activates myeloid differentiation gene 88 adaptor proteins, leading to upregulation of type I IFN and pro-inflammatory cytokines genes in DCs, macrophages, and B cells (Marongiu et al., 2019). The gut microbiota is closely linked to the host’s physiological function and immune ability (Kayama et al., 2020). Supplementation with Bifidobacterium bifidum plays a central role in reducing the occurrence and development of AD and improving gut dysbiosis (Bellomo et al., 2024). In particular, supplementation with B. bifidum increases beneficial intestinal microorganisms, such as the genus Bifidobacterium and Bacteroides, and reduces harmful microorganisms, such as Escherichia, Haemophilus, and Shigella. It also activates Treg and Th1 cells for immunomodulation and inhibits the activity of Th2 cells (Chichlowski et al., 2020).
This study focused on the effects of three types of B. bifidum (probiotics, postbiotics, CpG ODN) on gut microbiota, gut immunity regulation, and skin atopy through the interconnection of the Gut-Skin Axis. AD was induced by treating the dorsal skin of NC/Nga mice with DNCB to investigate the effect of B. bifidum CBT-BF3. The effects of probiotic, postbiotic, and CpG ODN on SCORing atopic dermatitis (SCORAD) intensity, body weight (BW), T cell differentiation in the mesenteric lymph nodes (mLNs) and spleen, and changes in major intestinal microbiota in AD mice were investigated.
Materials and Methods
A total of 30 five-week-old female NC/Nga mice (Central Lab, Seoul, Korea) were maintained at room temperature (22°C±1°C) and humidity (60±10%), with a 12-hour light-dark cycle during the experimental period. They were provided ad libitum access to AIN-76A pellet feed (Central Lab) and water. Probiotics were B. bifidum CBT-BF3 strain (KCTC12201BP; Cell Biotech, Gimpo, Korea) in the form of freeze-dried powder, and were orally administered at 2% of the BW (W:W, 2×109 CFU/g). In addition, postbiotics and CpG ODN were prepared from equivalent probiotics. After a one-week preliminary experimental period, the mice were randomly assigned into five groups, with six mice in each group: (1) Control group (C: basal diet), (2) Negative control group (N: basal diet, DNCB-AD), (3) Probiotics group (T1: basal diet, DNCB-AD+live B. bifidum), (4) Postbiotics group (T2: basal diet, DNCB-AD+heat-killed B. bifidum), (5) CpG ODN group [T3: basal diet, DNCB-AD+B. bifidum fragmented genomic (fg) DNA]. Throughout the 4-week challenge phase, BW and food intake were recorded weekly. At the end of challenge phase, mice were euthanized using diethyl ether anesthesia. The intestine, mLNs, spleen, and liver were collected, and the weights of the spleen and liver were measured. Intestinal contents from the small intestine, cecum, and large intestine were collected for microbiological analysis. The spleen and mLNs were rinsed with phosphate-buffered saline (PBS, pH 7.4) and then stored at –80°C for mRNA extraction.
Based on the method detailed by Shin et al. (2016), AD-like skin lesions were induced in mice by using DNCB (Sigma-Aldrich, St. Louis, MO, USA) following a one-week preliminary experimental period. The mice’s back hair was shaved using an electric clipper one day before the DNCB treatment. A 1% DNCB solution in an acetone olive oil (3:1) suspension was prepared and applied to the mice’s dorsal skin twice a week for the sensitization phase (3 weeks). Three weeks after AD induction, probiotic B. bifidum, postbiotic B. bifidum, and CpG OND B. bifidum were dissolved in PBS (pH 7.4) and 0.2 mL was administered orally three times a week using a feeding needle to the treatment group, while only PBS (pH 7.4) was administered to the C group (control group) and the N group (negative control group) during the challenge period (4 weeks). The mice were challenged with 0.5% DNCB weekly during feeding.
Postbiotic B. bifidum was prepared by dissolving probiotic B. bifidum CBT-BF3 in PBS (pH 7.4) and heat-treating at 121°C for 20 minutes under an overpressure of 1.1 atm, and then stored in a –80°C freezer until oral administration. CpG OND B. bifidum was prepared by extracting gDNA from probiotic B. bifidum CBT-BF3 using ZR Fecal DNA MiniPrepTM (Zymo Research, Irvine, CA, USA). gDNA was digested with Sau3AI restriction enzyme (New England Biolabs, Rowley, MA, USA) at 37°C for 5 minutes, treated at 65°C for 20 minutes to terminate the enzyme reaction, dissolved in PBS (pH 7.4), and stored in a –80°C freezer. The size of the digested gDNA fragments was confirmed by 2% agarose gel electrophoresis, and fragmented gDNA (fgDNA) that was less than 500 bp in size was used as CpG OND B. bifidum (Supplementary Fig. S1).
The severity of AD was visually assessed once a week following treatment with DNCB. The SCORAD index, as described by Oranje et al. (2007), was used to determine the severity level. Erythema, edema/papules, scratching, dryness, lichenification, and oozing/crust formation were scored as absent (0), mild (1), moderate (2), or severe (3), and the scores for these six symptoms were added together to determine the AD intensity. The score for the most representative lesion was used, and the assessments were performed by a single investigator who was blinded to the treatments in order to minimize technique variations throughout each experiment.
To assess the immunomodulatory effects of probiotic B. bifidum, postbiotic B. bifidum, and CpG ODN B. bifidum in DNCB-induced AD mice, the mice were sacrificed, and their spleens and mLNs were collected. The spleen and mLNs tissues were placed in Trizol® reagent (Ambion, Austin, TX, USA) and homogenized using Silent Crusher M (Heidolph, Schwabach, Germany) for RNA isolation, following the method of Chomczynski and Sacchi (1987). The isolated RNA was stored at –80°C for cDNA synthesis. cDNA synthesis was performed at 50°C for 30 minutes using an reverse transcription-polymerase chain reaction (RT-PCR) kit (Enzynomics, Daejeon, Korea). The quantitative PCR (qPCR) amplification cycle conditions were as follows: initial denaturation (95°C, 10 min), 35 cycles of denaturation (95°C, 30 s), annealing (55°C, 30 s), extension (72°C, 1 min), and final extension (72°C, 5 min). The PCR primers used in this study can be found in Supplementary Table S1, and the glyceraldehyde-3-phosphate dehydrogenase (GAPDH) housekeeping gene was used for normalization. To assess the effects of the three types of B. bifidum on T cell differentiation, the mRNA expression levels of transcription factors (T-bet, GATA-3, RORγT, Foxp3) and cytokines (IFN-γ, IL-4, IL-17, TGF-β) genes of Th1, Th2, Th17, and Treg cells were analyzed. Additionally, the mRNA expression levels of genes associated with AD, including Gal-9, FLG, and TSLP, were also analyzed.
In order to analyze the effects of the three types of B. bifidum on significant intestinal microorganisms, mice were sacrificed, and the contents of the small intestine, large intestine, and cecum were collected. Intestinal microorganism gDNA was extracted using ZR Fecal DNA MiniPrepTM (Zymo Research). Real-time qPCR was performed using a Rotor-Gene SYBR® Green PCR kit (Qiagen, Hilden, Germany), and the PCR cycling conditions were denaturation (95°C, 10 s), annealing (56°C, 30 s), and extension (72°C, 10 s) for 40 cycles. The primers utilized for qPCR analysis are listed in Supplementary Table S2, while intestinal microorganism universal primers were employed as an internal reference for normalization. Ten representative microorganisms were analyzed by classifying the intestinal microorganisms into four functions (anti-obesity, obesity, butyric acid production, and lactic acid production) through a literature review. Regarding obesity, Bacteroides spp. was selected as an anti-obesity bacterium (De Filippo et al., 2010), and Ruminococcus spp. was selected as an obesity bacterium (Palmas et al., 2021). In addition, four butyric acid-producing bacteria, Bifidobacterium spp., Faecalibacterium prausnitzii, and Roseburia spp. (Barcenilla et al., 2000; Duncan et al., 2004), and five lactic acid-producing bacteria, Leuconostoc citreum, Leuconostoc mesenteroides, Lactobacillus sakei, Weissella cibaria, and Weissella koreensis (Choi et al., 2024; Lee et al., 2022b), were selected, and these bacteria are beneficial intestinal bacteria that have anti-inflammatory effects and function as immunostimulants.
Results
To analyze the effects of the three types of B. bifidum on SCORAD intensity, AD was induced during a 3-week sensitization phase, and then the SCORAD intensity of the dorsal skin lesions was measured once a week during a 4-week challenge phase (Fig. 1A). The SCORAD intensity decreased from week 5 to week 7 of the challenge phase in the C and T groups compared to the N group (p<0.05). In particular, the T1 group showed a significant decrease at seven weeks compared to the other T groups (p<0.05). Compared to the C group, the AD skin lesions in the N group were drier and had more dead skin cells, and as the treatment period progressed, the AD symptoms in the T groups recovered to the level of the C group (Figs. 1B and C). DNCB-induced AD in the dorsal skin increased the SCORAD intensity due to a local inflammatory response. However, oral administration of the three types of B. bifidum decreased the SCORAD intensity in the skin lesions through the interconnection of the microbiome-immune-skin axis.
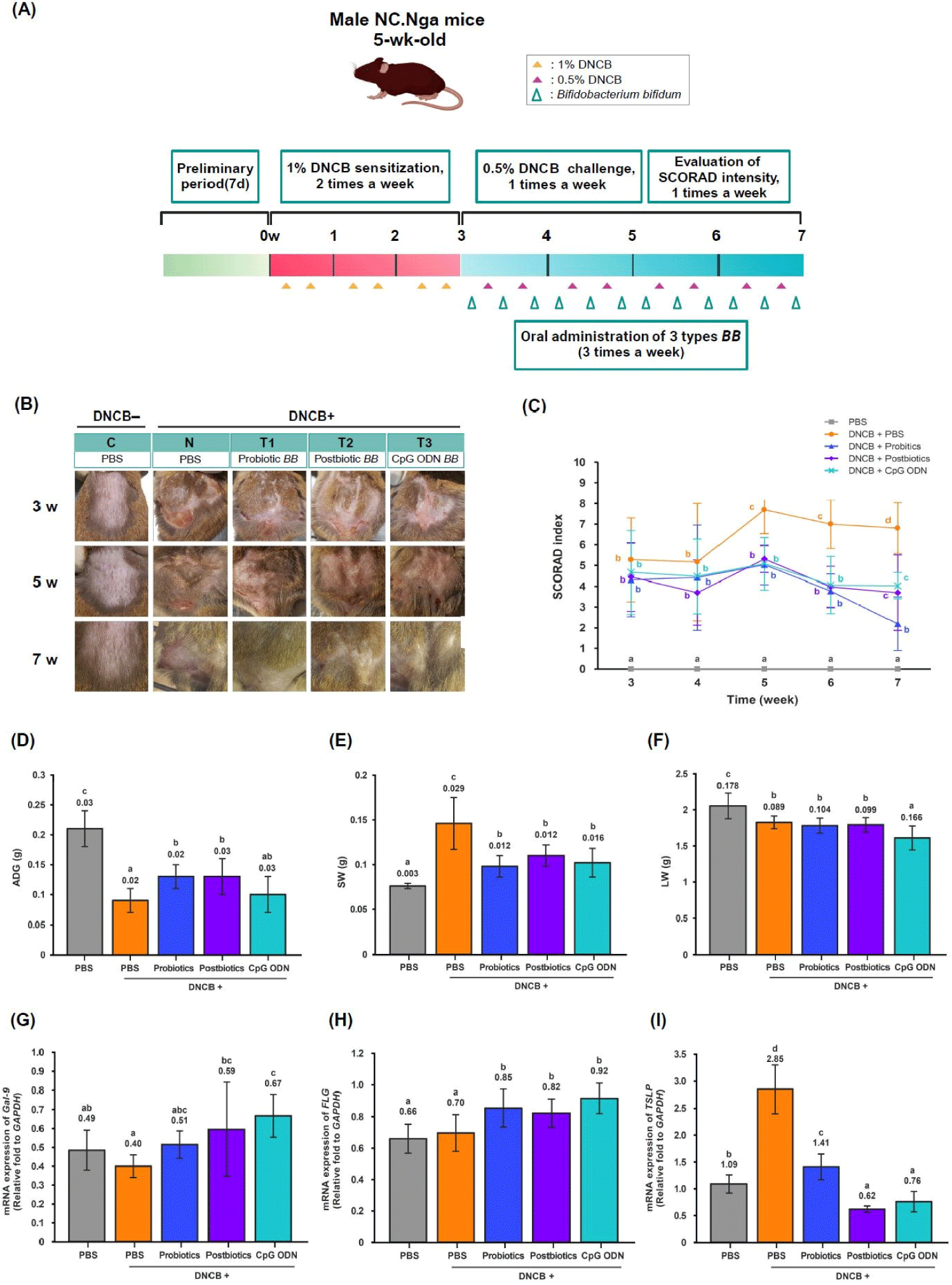
Cutaneous inflammation is a localized skin problem and causes inflammation in various organs through a multi-directional communication axis, leading to comorbidities such as weight loss and amyloidosis (Blancas-Mejía and Ramirez-Alvarado, 2013). In this study, a preliminary test period of one week was conducted to allow the experimental animals to adapt to the environment, and the main test period was conducted for seven weeks. The main test period was divided into a 3-week sensitization phase and a 4-week challenge phase. The effects of the three types of B. bifidum (probiotic B. bifidum, postbiotic B. bifidum, and CpG ODN B. bifidum) on BW were investigated once a week for seven weeks, and spleen weight (SW) and liver weight (LW) were measured at the end of the 7 weeks (Fig. 1A). Average daily weight gain (ADG) was lower in the N and T groups compared to the C group and increased in the T1 and T2 groups compared to the N group (p<0.05; Fig. 1D). Mice with AD have increased scratching behavior and energy expenditure and decreased BW (Kawano and Umemura, 2013). In this study, the AD mice showed a decrease in BW, but oral administration of the three types of dietary B. bifidum showed a tendency to increase their BW. The DNCB-induced AD mice showed decreased BW due to the multi-directional communication via the skin-organ axis, and the three types of B. bifidum increased their BW via the microbiome-immune-organ axis. SW was higher in the N and T groups compared to the C group and was significantly lower in the T groups compared to the N group (p<0.05; Fig. 1E). LW was lower in the N and T groups compared to the C group and lower in the T3 group compared to the N group (p<0.05; Fig. 1F). These results suggest that DNCB-induced AD in the dorsal skin increased SW and decreased LW through the multi-directional communication of the skin-organs axis. However, oral administration of the three types of B. bifidum to AD mice showed a tendency to restore LW to normal through the communication of the microbiome-immune axis.
Gal-9 is a galectin protein that contains two carbohydrate-recognition domains. It is expressed in immune and non-immune cells and regulates important biological functions such as cell-cell signaling, immune responses, cell growth, differentiation, and cell death (Hirashima et al., 2002). Additionally, Gal-9 acts as an immunomodulator, increasing the population of regulatory T cells and immunosuppressive macrophages to control excessive immune reactions (Ikeda et al., 2017). FLG is a structural protein that plays a crucial role in forming the skin’s barrier. It is also involved in aggregating keratin intermediate filaments, preventing water loss through the skin, modulating the immune response, and providing protection against bacteria. When FLG levels decrease, the skin’s barrier function is compromised (Hughes et al., 2024). TSLP is a cytokine produced by epithelial cells that influences DCs and contributes to allergic and inflammatory diseases (Song et al., 2024). This study analyzed the effects of oral administration of the three different types of B. bifidum on the expression of Gal-9, FLG, and TSLP genes in mLNs using RT-qPCR.
The expression of the Gal-9 gene in the mLNs did not differ between the C and N groups but was higher in the T2 and T3 groups compared to the N group (p<0.05; Fig. 1G). There was no difference in the expression of the FLG gene between the C and N groups, but it was higher in the T groups compared to the N group (p<0.05; Fig. 1H). The expression of the TSLP gene was higher in the N group compared to the C group and lower in the T groups compared to the N group (p<0.05; Fig. 1I). The AD mice promoted the expression of the TSLP cytokine gene in the mLNs. However, the three types of B. bifidum treatment induced the expression of Gal-9 and FLG genes and suppressed the expression of the TSLP cytokine gene. Postbiotic B. bifidum and CpG ODN B. bifidum were particularly effective.
TSLP is released by epithelial cells and stromal cells in skin, gastrointestinal tract, and lung when they are exposed to allergens, chemicals, and microorganisms. It is a pleiotropic cytokine that affects various cell types (including DCs, mast cells, T cells, B cells, neutrophils, and eosinophils) and promotes Th2-type immunity. This enhances the immune responses to allergens through adaptive and innate immune systems (Ebina-Shibuya and Leonard, 2022). Additionally, TSLP can exacerbate inflammation by acting as an alarmin, being rapidly released from cells, and inducing both endogenous and exogenous danger signals.
In this study, AD induction using DNCB in the dorsal skin of mice promoted the expression of TSLP cytokine genes in the mLNs through the interconnection of the skin-gut axis. However, oral administration of the three types of B. bifidum alleviated the clinical symptoms of AD in the skin lesions by promoting Gal-9 and FLG gene expression in the mLNs and suppressing TSLP cytokine gene expression through the bi-directional communication of the microbiome-immune axis. DNCB-induced AD mice promoted the expression of the TSLP cytokine gene, and TSLP cytokine acted as a master regulator of the Th2 immune response, activating Th2 and Th17 cells. However, the three types of B. bifidum played an essential role in maintaining gut-skin homeostasis by suppressing the activity of TSLP, Th2 cells, and Th17 cells and promoting the activity of Treg cells.
AD that occurs in the dorsal skin of mice causes local and systemic inflammation, which may be caused by an imbalance in the immune response of Th1, Th2, Th17, and Treg cells (Sheikhi et al., 2017). It is known to be mediated mainly by Th2 cells secreting IL-4, IL-5, IL-9, and IL-13 and is influenced by genes related to allergic inflammatory responses and individual genetic factors (Steinke et al., 2003). On the other hand, Treg cells secrete cytokines TGF-β and IL-10, which suppress excessive immune responses by Th2 cells, thereby controlling AD symptoms (Palomares et al., 2010). Therefore, this study analyzed the expression levels of transcription factors and cytokine genes of Th1, Th2, Th17, and Treg cells in the mLNs.
The mLNs are the most prominent lymph nodes in humans and other animals. As a component of gut-associated lymphoid tissues, they play a crucial role in immune defense as a central checkpoint for mucosal immunity (Lyu et al., 2022). In this study, to investigate the effect of oral administration of the three types of B. bifidum on the changes in T cell populations, the expression levels of specific transcription factors T-bet, GATA-3, RORγT, Foxp3, and major cytokines IFN-γ, IL-4, IL-17, TGF-β genes of Th1, Th2, Th17 and Treg cells in mLNs were analyzed using RT-qPCR.
The expression level of the Th1 cell transcription factor T-bet gene increased in the T2 and T3 groups compared to the T1 group, and the expression level of the cytokine IFN-γ gene decreased in the N group compared to the C group (p<0.05; Fig. 2A). The expression levels of the Th2 cell transcription factor GATA-3 and cytokine IL-4 genes were significantly increased in the AD-induced N group compared to the C group (p<0.05). However, compared to the N group, the expression levels of the transcription factor GATA-3 and cytokine IL-4 genes decreased in the T groups, and in particular, the transcription factor GATA-3 showed a tendency to decrease to the level of the C group (p<0.05; Fig. 2B). The expression levels of the transcription factor RORγT and cytokine IL-17 gene in the Th17 cells increased in the N group compared to the C group (p<0.05; Fig. 2C). However, compared to the N group, the expression levels of transcription factor RORγT and cytokine IL-17 decreased in the T groups, and in particular, the expression level of the cytokine TGF-β gene decreased to the C group level (p<0.05). The expression level of the transcription factor Foxp3 gene in the Treg cells was significantly decreased in the N group compared to the C group (p<0.05; Fig. 2D). However, compared to the N group, the expression level of the transcription factor Foxp3 gene increased in the T groups (p<0.05), and the expression level of the cytokine TGF-β gene increased in the T2 and T3 groups (p<0.05). In particular, the T3 group showed increased expression levels of the transcription factor Foxp3 and cytokine TGF-β genes compared to the C and N groups (p<0.05).
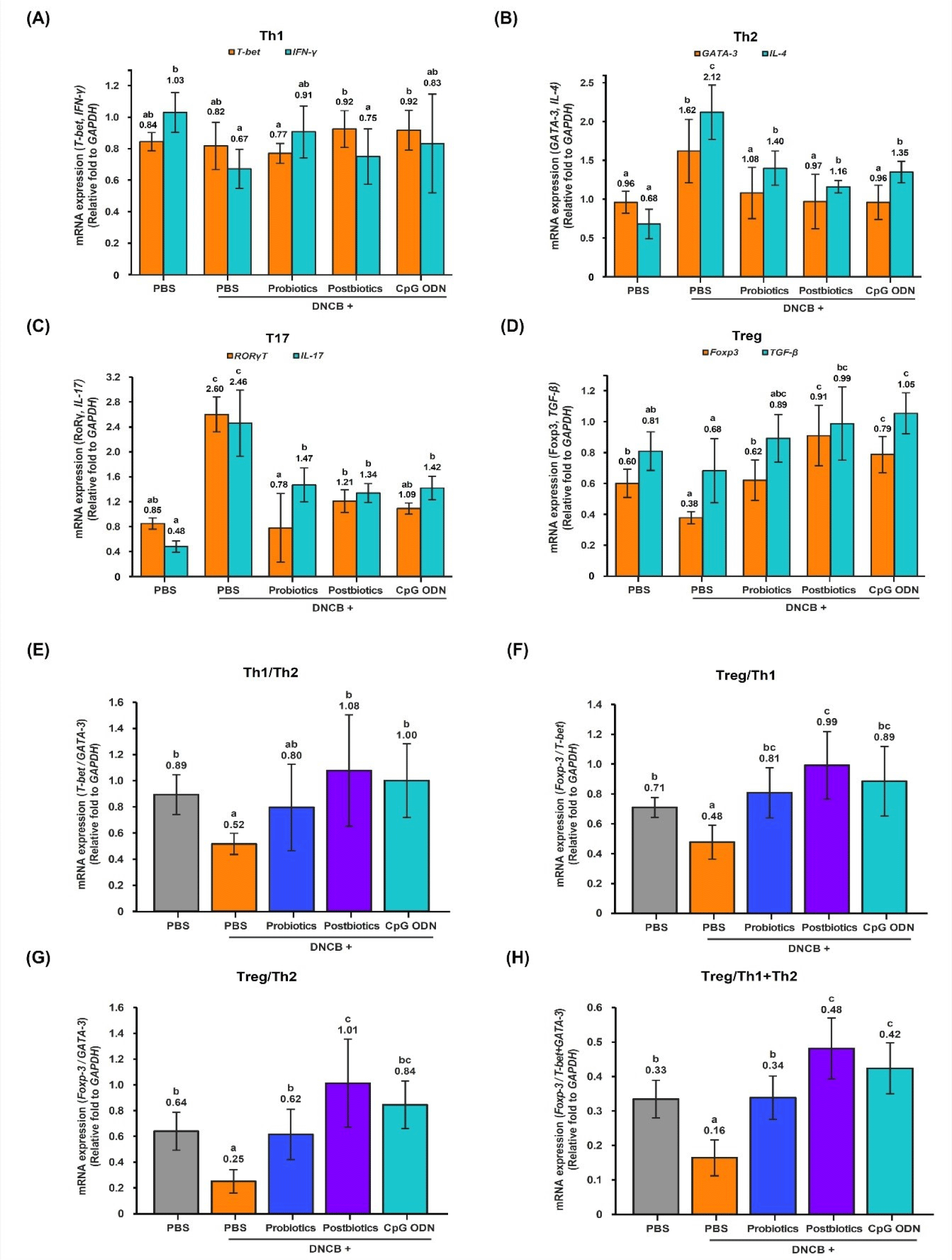
Mice with AD induced on their dorsal skin had enhanced activity of Th2 and Th17 cells in the mLN via skin-gut axis interconnections. However, oral administration of the three types of B. bifidum suppressed the activity of these cells through the bi-directional communication of the microbiome-immune axis. Therefore, the three types of B. bifidum showed the effect of regulating immunity by inhibiting the differentiation of Th2 and Th17 cells, which are central to the AD response, and promoting the differentiation of Treg cells.
DCs are a type of antigen-presenting cells that play a crucial role in connecting the body’s innate and adaptive immune responses (Banchereau and Steinman, 1998). Immature dendritic cells (iDCs) precursors travel through the bloodstream to different tissues, including the gut, where they interact with intestinal bacteria at mucosal sites. The iDCs’ pattern recognition receptors are responsible for recognizing specific molecular patterns of microbial carbohydrates, proteins, nucleic acids, and lipids. When stimulated by microbial cues, DCs produce cytokines that prompt naive T cells to differentiate into various lineages such as Th1, Th2, Th17, or Treg. The Treg population works to suppress cell proliferation and the differentiation of Th1, Th2, and Th17 by releasing anti-inflammatory cytokines like IL-10 and TGF-β. As a result, there is a growing interest in studying how probiotics can affect DC priming and regulate T-cell responses (Lasaviciute et al., 2022).
Th1/Th2 Balance: The expression ratio of Th1/Th2 transcription factors (T-bet/GATA-3) decreased in the N group compared to the C group (p<0.05; Fig. 2E). However, compared to the N group, it increased in the T2 and T3 groups (p<0.05), and there was no significant difference between the C and T groups, indicating that the three types of B. bifidum treatment had the effect of modulating the balance of Th1/Th2 (p<0.05). Treatment with the three types of B. bifidum in AD mice restored the Th1/Th2 balance to normal, and postbiotic B. bifidum and CpG ODN B. bifidum were effective. Treg/Th1 balance: The expression ratio of Treg/Th1 transcription factors (Foxp3/T-bet) decreased in the N group compared to the C group (p<0.05; Fig. 2F). However, it increased in the T groups (p<0.05) compared to the N group. In the comparison between the C group and the T groups, the T1 group and the T3 group did not show significant differences from the C group. This indicates that the three types of B. bifidum treatment modulated the Treg/Th1 balance (p<0.05). Therefore, the three types of B. bifidum treatment in AD mice predominantly activated Treg cells in the Treg/Th1 balance, and postbiotic B. bifidum was particularly effective. Treg/Th2 Balance: The expression ratio of Treg/Th2 transcription factors (Foxp3/GATA-3) decreased in the N group compared to the C group (p<0.05; Fig. 2G). However, compared to the N group, it increased in the T groups, and there was no significant difference between the C group and the T groups, indicating that the three types of B. bifidum treatments had a modulating effect on the Treg/Th2 balance (p<0.05). Therefore, Treg cell activation was dominant in the Treg/Th2 balanced by the three types of B. bifidum treatment in the AD mice, and postbiotic B. bifidum was particularly effective. Treg/Th1+Th2 Balance: The expression ratio of Treg/Th1+Th2 transcription factors (Foxp3/T-bet+GATA-3) decreased in the N group compared to the C group (p<0.05; Fig. 2H). However, it increased in the T groups compared to the N group (p<0.05). In a comparison between the C and T groups, the modulating effect of the three types of B. bifidum treatments on the balance of Treg/Th1+Th2 was confirmed by an increase in the T2 and T3 groups (p<0.05). Therefore, in the Treg/(Th1+Th2) balance in the mLNs of AD mice treated with the three types of B. bifidum, Treg activation was dominant, and postbiotic B. bifidum and CpG ODN B. bifidum were effective.
The gut microbiota is essential for regulating adaptive and innate immune responses and maintaining immune homeostasis (Postler and Ghosh, 2017). Animals and humans with AD exhibit an imbalance in the gut microbiota, which is characterized by decreased bacterial diversity and abundance of beneficial bacteria, such as Lactobacillus and Bifidobacterium, and increased abundance of harmful bacteria, such as Clostridium difficile (Peroni et al., 2020). In this study, to analyze the effects of oral administration of three types of B. bifidum on changes in the intestinal microbiota, a total of 10 species were selected through a literature review and presented in the Materials and Methods section.
Bacteroides spp. did not show any differences between the C and N groups but increased in the T groups compared to the C and N groups (p<0.05). Ruminococcus spp. showed no differences between the C and N groups but decreased in the T groups compared to the C and N groups (p<0.05). Therefore, the three types of B. bifidum treatment increased anti-obesity related Bacteroides spp. and decreased obesity related Ruminococcus spp. (Figs. 3A and B). Bifidobacterium spp. showed no differences between the C and N groups but increased in the T groups (p<0.05). F. prausnitzii and Roseburia spp. decreased in the N group compared to C and increased in the T groups compared to the N group (p<0.05). Therefore, the three types of B. bifidum treatment increased the Bifidobacterium spp., F. prausnitzii, and Roseburia spp. (Figs. 3C, D, and E). Lactic acid-producing bacteria Leuconostoc mesenteroides, L. citreum, W. cibaria, W. koreensis, and L. sakei are gut-derived microorganisms. Leuconostoc mesenteroides and L. sakei did not show any differences between the groups. However, W. cibaria and W. koreensis decreased in the N and T groups compared to the C group (p<0.05). L. citreum decreased in the N and T groups compared to the C group and decreased in the T2 and T3 groups compared to the N group (p<0.05; Figs. 3F, G, H, I, and J).
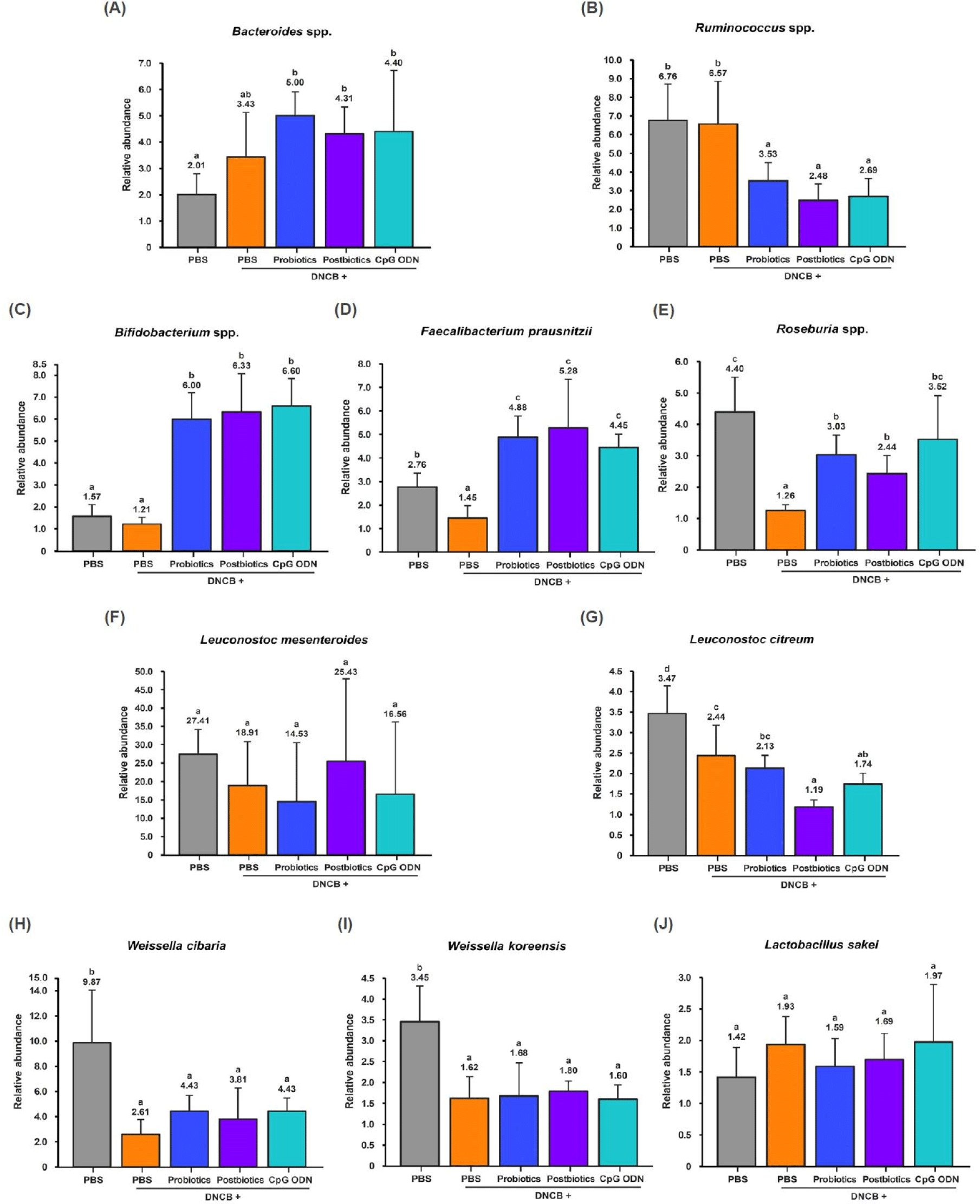
When AD was induced, butyric acid-producing bacteria F. prausnitzii and Roseburia spp., and lactic acid-producing bacteria L. citreum, W. cibaria and W. koreensis decreased (p<0.05). However, oral administration of the three types of B. bifidum increased the anti-obesity microorganisms Bacteroides spp. and butyric acid-producing bacteria Bifidobacterium spp., F. prausnitzii, and Roseburia spp. (p<0.05). The gastrointestinal tract is a microbiologically active ecosystem that is vital to the mucosal immune system. Oral administration of probiotics can modulate the intestinal microbiota, activate the signal networks, and stimulate the mucosal and systemic immune systems by bacteria or bacteria-derived bioactive molecules (cell walls, polysaccharide moieties, SCFAs, CpG ODN; Fig. 4).
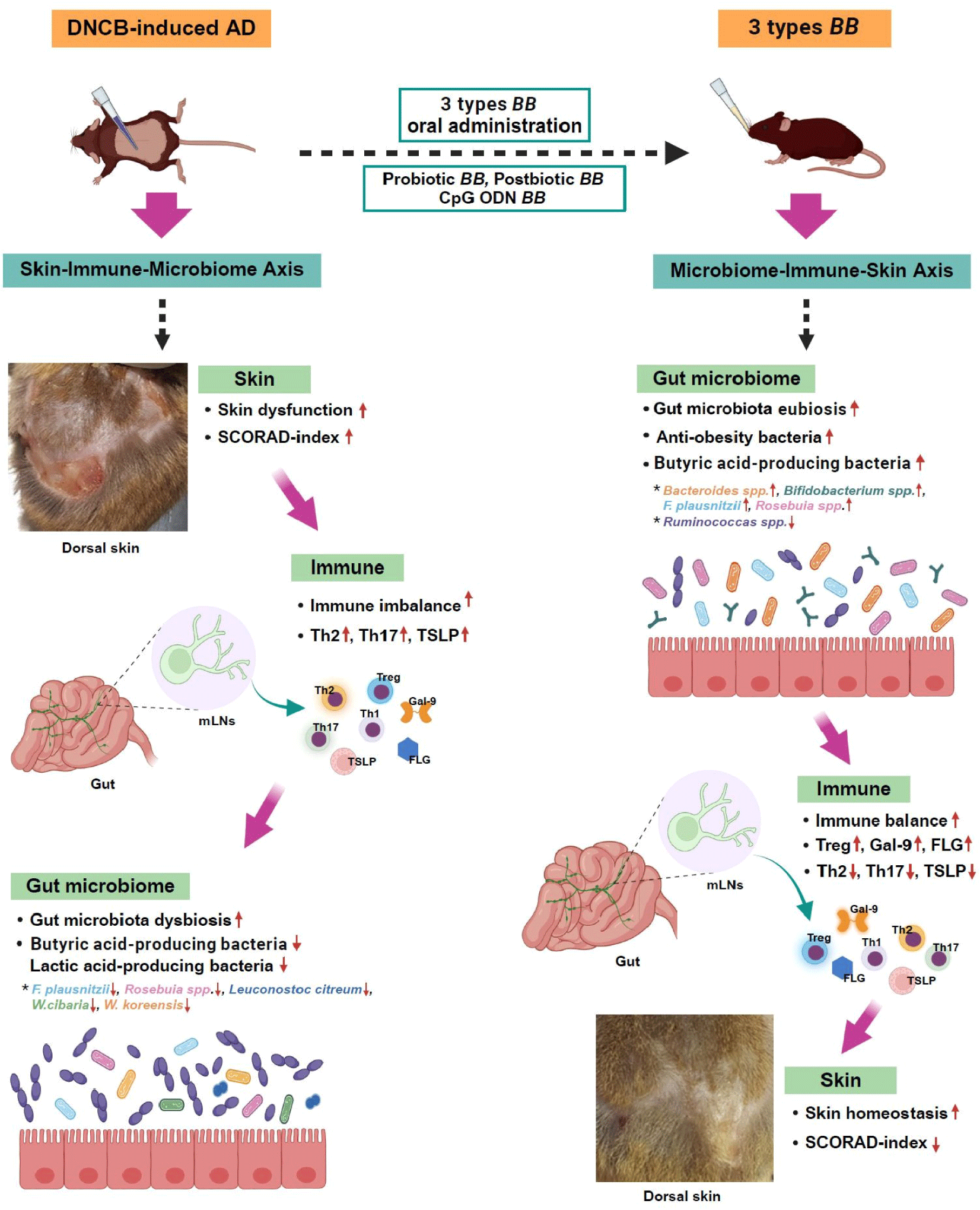
Discussion
AD is a skin condition characterized by symptoms such as rashes, swelling, and peeling of the skin. It is a complex condition involving abnormalities in the immune system, environmental factors, defects in the skin barrier function, and genetic predisposition (Fang et al., 2020; Puar et al., 2021). The skin’s immune system responds to external or internal stimuli by producing inflammatory cytokines. Keratinocytes in the epidermis release cytokines that activate the immune system and lead to local and systemic inflammation (Nakanishi et al., 2023). Cutaneous inflammation is characterized by high expression of the skin-derived inflammatory cytokines IL-17, and continuous systemic release of IL-17 causes amyloidosis-like damage to distant organs (Iida et al., 2022). Amyloidosis is a heterogeneous disease in which insoluble amyloid fibrils (misfolded proteins) accumulate in organs or tissues, causing localized or systemic organ dysfunction. Amyloid accumulates in the liver, spleen, kidney, and heart, causing various clinical syndromes, including cardiomyopathy and hepatomegaly (Bustamante and Zaidi, 2023). The most prevalent amyloid types detected across all anatomic sites are immunoglobulin light chain (59%) and transthyretin (28%), with these two proteins accounting for the majority (>85%; Chiu et al., 2023). Systemic amyloidosis presents with many non-specific symptoms, including loss of appetite, weight loss, fatigue, and weakness (Blancas-Mejía and Ramirez-Alvarado, 2013).
The spleen is a representative secondary organ that contains various immune cells and plays an important role in regulating immune responses. Additionally, the spleen becomes larger when infection or inflammation occurs in the body. In BALB/c mice, 2,4-dinitrofluorobenzene-induced AD increased spleen weight (p<0.001), and treatment with Sarsasapogenin (steroidal sapogenin) and Fluticasone (glucocorticoids) decreased spleen weight (p<0.05; Mandlik et al., 2021). DNCB-treated AD mice showed significant increases in spleen weight and LW (p<0.05; Kim et al., 2018c). In addition, AD mice had increased spleen weight but decreased productivity, and the number of total lymphocytes, CD4, CD8, and CD20, was reduced (Ko et al., 2019).
DNCB-induced AD mice have an increased SCORAD-index as well as increased ear thickness, serum IgE, and serum histamine levels, but probiotics supplementation alleviates these symptoms (Kim et al., 2018b; Kim et al., 2019; Kim et al., 2020). DNCB, which is captured by local skin DCs, acts as an allergen-associated hapten to induce inflammatory responses in the skin (Riedl et al., 2023), which leads to an imbalance of Th1/Th2 immune cells in the gut mucosa (Yuqing et al., 2014). DNCB-induced AD mice have enlarged cervical lymph nodes, increased weight, and increased mRNA expression levels of Th1 cytokine IFN-γ, Th2 cytokines IL-4, and Th17 cytokine IL-17A in ear skin and lymph nodes (Lee et al., 2022a). In addition, AD mice showed increased scratching behavior and serum IgE levels and decreased BW gain (Seino et al., 2011). Thus, AD is a significant stress that affects the regulation of biological processes in a variety of ways.
The intestinal microbial composition of 21 allergic and 18 healthy infants was investigated at three weeks, three months, and six months of age. Healthy infants were colonized with a higher abundance of commensal Bifidobacterium, whereas, in allergic infants, the opportunistic pathogen Klebsiella was significantly abundant. Surprisingly, infants with a higher Klebsiella/Bifidobacterium ratio at three months of age had a higher chance of developing allergies by three years, whereas infants with a lower K/B ratio did not (Low et al., 2017). One hundred thirty mothers were supplemented with probiotics (Bifidobacterium breve M-16V and Bifidobacterium longum BB536) starting one month before delivery and their infants were supplemented for six months after delivery while another 36 mother-infant pairs that did not receive bifidobacterial supplementation. The probiotic group showed a significant decrease in the incidence of eczema and AD and the proportion of Proteobacteria during the first 18 months after birth (Enomoto et al., 2014). Bellomo et al. (2024) reported that supplementation of the probiotic B. bifidum for six months in 164 infants born by cesarean section significantly reduced the incidence of atopy and respiratory infections during the first year of life compared to 249 infants in a control group. Additionally, B. bifidum supplementation significantly increased Bacteroidota, Actinomycetota, and Bifidobacterium and decreased Escherichia coli, Shigella, and Haemophilus. Oral administration of a synbiotic mixture (B. longum and galactooligosaccharide) to AD mice improved DNCB-induced skin inflammation, abnormal transepidermal water loss, AD-like skin, and epidermal barrier protein FLG deficiency (Kim et al., 2022). Vorobieva et al. (2023) conducted a study on 92 children ages 4 to 5 with food allergy symptoms. T group children (n=46) were supplemented with probiotics (Lactobacillus rhamnosus GG, Bifidobacterium animalis spp. lactis ВB-12) for 21 days, while C group children (n=46) were not supplemented with probiotics. The SCORAD index of the T group children decreased from 12.4±2.3 to 7.6±1.8 (р≤0.05) and decreased significantly more than that of the C group (SCORAD index changed from 12.1±2.4 to 12.2±1.9; р≤0.05). In the T group, pro-inflammatory cytokine IL-17 decreased by 27%, and anti-inflammatory cytokine IL-10 increased by 38.9% (р≤0.05). The IgE level of the T group children decreased by 38.0%, but the IgE level of the C group children did not change (р≤0.05). When a probiotic mixture (Lacticaseibacillus casei, Lactobacillus plantarum, L. rhamnosus, and B. lactis) was orally administered to mice with AD, a Th1 cell-mediated immune response was elicited, whereas Th2 and Th17 cell-mediated immune responses were suppressed. In addition, oral administration of probiotics increased the number of Treg cells in the Peyer’s patches of AD mice and the mRNA expressions of Gal-9 and FLG genes in the mLNs, whereas it decreased the mRNA expression of the TSLP cytokine gene. These results suggest that probiotics may act as effective immunomodulators in AD patients by regulating DCs to induce Th1 and Treg responses and as potential preventive agents for AD (Kim et al., 2018a; Kim et al., 2019; Yu et al., 2023).
Gal-9 is widely expressed in various cellular organelles such as the cell membrane, cytoplasm, and nucleus. It performs various functions by binding to receptors. Additionally, Gal-9 is present in activated CD4+ Th1 and Th17, but not in Th2 lymphocytes, DCs, and macrophages (Nio-Kobayashi and Itabashi, 2021). TSLP is produced by various cells in AD skin lesions. It induces allergic inflammatory responses by promoting the maturation of DCs and the differentiation of naive CD4+ T cells into inflammatory Th2 cells (Ebina-Shibuya and Leonard, 2022). FLG is an essential protein for the skin barrier. It is broken down into water-soluble, low-molecular-weight molecules such as free amino acids, pyrrolidone carboxylic acid, and urocanic acid. These components act as natural moisturizing factors for the skin and have an immunomodulatory effect. Impaired skin barrier function due to decreased FLG expression in the epidermis increases allergen influx, stimulating the production of TSLP, IL-25, and IL-33 in keratinocytes. Therefore, maintaining skin barrier function by upregulating FLG in keratinocytes protects against AD (Hasegawa et al., 2022). Bifidobacterium is a representative beneficial bacterium that activates naive CD4+ T cells and promotes the polarization of Treg cells in the intestines of breast-fed infants. It is effective in treating inflammatory diseases such as AD (López et al., 2011). In addition to live probiotic cells, non-viable cells and bioactive molecules derived from cells, known as postbiotics, have also gained significant attention for their potential use in advanced biological therapies. Postbiotics have the advantages of easy production and guaranteed safety and stability and they are commonly used for food additives and safe pharmaceutical applications (D’ambrosio et al., 2024). Jeong et al. (2020) conducted a study on 66 children (ages 1–12) with moderate AD symptoms. The T group children (n=33) were supplemented with postbiotics (heat-killed L. rhamnosus IDCC 3201), while C group children (n=33) were not supplemented with postbiotics. T group children showed a decrease in SCORAD-index, levels of eosinophil cationic protein, and IL-31, suggesting that postbiotics have a therapeutic effect on AD. Oral administration of postbiotics (heat-killed B. bifidum B1628) to DSS-induced colitis mice decreased the serum levels of pro-inflammatory cytokines IL-1β and TNF-α. It increased the level of anti-inflammatory cytokine IL-13. It also improved DSS-induced gut dysbiosis, increasing beneficial bacteria such as Lactobacillus and decreasing unfavorable taxa associated with inflasmmatory bowel diseases, such as Alistipes indistinctus, Lachnospiraceae bacterium 3_1_46FAA, Porphyromonadaceae, and Subdoligranulum (Feng et al., 2022).
The gastrointestinal tract serves as the primary entry point for foreign agents from the external environment to enter the host and is responsible for about 70% of the immune system (Backhed et al., 2005). Among commensal microbiota, lactic acid-producing bacteria B. infantis and L. rhamnosus are well-known as beneficial microorganisms that induce the activity of Tregs cells. The primary mechanisms by which these commensals induce the activation of Tregs cells include extracellular microbial products, such as SCFAs, polysaccharide moieties, and gDNA contained in postbiotics. The gDNA GC content of B. longum infantis, L. rhamnosus, and E. coli are 59.86%, 46.76%, and 50.78%, respectively. B. longum infantis gDNA is a potent Treg cell inducer and showed a dose-dependent response pattern when the dose threshold of gDNA was 20 mg, but no Treg induction response was observed in the gDNA of L. rhamnosus and E. coli (Li et al., 2020). Additionally, a unique CpG methylated motif was found in the gDNA of B. longum infantis but not in L. rhamnosus and E. coli strains. These motifs in B. longum infantis gDNA activate Toll-like receptor 9 (TLR 9) to exert immunostimulatory effects. Bifidobacterium may have many CpG motifs due to their high GC content, and this characteristic may lead to immunostimulatory effects. Therefore, these results suggest that B. longum infantis and L. rhamnosus strains contribute to health through different mechanisms. Additionally, methylated CpG ODN from B. longum infantis offers properties for treating immunologic diseases such as AD in which Treg cell populations are reduced. CpG-ODN derived from Cryptococcus neoformans and the methylated CpG sites present in the genomic DNA of B. infantis induce Th1 or Treg cell differentiation (Jacquet, 2021).
Imbalances in the gut microbiota can disrupt gut immune balance and are also linked to the development of allergies in infants. In studies with twin cohorts (some infants with, some without allergies) and mice, allergic infants had increased Ruminococcus gnavus. Sensitization and challenges with ovalbumin in mice resulted in a rapid increase in endogenous R. gnavus. Additionally, oral administration of purified R. gnavus to mice produced histologic evidence of airway inflammation. The expansion of R. gnavus stimulated the secretion of cytokines IL-25, IL-33, and TSLP in colon tissues, activated type 2 innate lymphoid cells and DCs. It promoted the differentiation and production of Th2 cells. Eosinophils and mast cells spread this phenomenon to the colon and lung parenchyma (Chua et al., 2018). Supplementation of a probiotic mixture in AD children significantly increased Bacteroides fragilis and L. acidophilus in the gut microbiome profile (Choy et al., 2023). Climent et al. (2021) reported that probiotics (B. animalis subsp. lactis CECT 8145, B. longum CECT 7347, and L. casei CECT 9104) supplementation significantly increased the genera Bacteroides, Ruminococcus, and Bifidobacterium and decreased Faecalibacterium. The gut microbiome of patients with AD showed a decrease in butyrate and propionate producers F. prausnitzii (Song et al., 2016), and orally administering F. prausnitzii and Akkermansia muciniphila to DNCB-induced AD mice reduced the levels of AD-related markers such as the dermatitis score, scratching behavior, and serum IgE level, and decreased the production of TSLP and Th2 cytokines (Lee et al., 2022c). F. prausnitzii is a micro-biomarker of inflammatory diseases and is significantly reduced along with butyrate in the gut microbiome of atopic dermatitis patients (Effendi et al., 2022).
Gut-microbial butyrate is one of the physiologically important SCFAs and is produced when Faecalibacterium and Roseburia metabolize carbohydrates. Butyrate serves as an energy source for colonocytes, maintains gut barrier integrity, limits the production of pro-inflammatory cytokines IL-6 and IL-12, and inhibits oncogenic pathways. Additionally, gamma-aminobutyric acid acts as a neurotransmitter to inhibit itch-signaling and alleviate skin lesions by balancing Th1 and Th2 levels (Song et al., 2016). In particular, Roseburia produces anti-carcinogenic metabolites such as conjugated linoleic acid precursor and shikimic acid. Therefore, butyrate producers Faecalibacterium and Roseburia are commensal bacteria expected to be next-generation probiotics or microbial therapeutic agents to restore imbalances in the intestinal ecosystem to normal (Singh et al., 2022).
Conclusion
This study confirmed that the three types of B. bifidum ameliorate the clinical syndromes of AD through the multidirectional communication of the gut-skin axis. In particular, the effect of modulating the Treg/Th2/Th17 balance and suppressing TSLP cytokine was more prominent in the mLNs than in the spleen (Supplementary Figs. S1, S2, and S3). When AD was induced in the dorsal skin, skin-derived inflammatory cytokines caused systemic inflammation in the spleen and mLNs through the skin-gut axis, and the gut microbiota changed.
Among the three types of B. bifidum, the first type, probiotics, is a short-lived fermented product that is expected to be used to modulate intestinal microbiota and maintain immune homeostasis. The second type, postbiotics, is a product that has a long shelf life and is expected to be used for food additives and safe pharmaceutical applications. The third type, CpG ODN, is expected to be used for vaccine adjuvants, the development of CpG ODN nanomedicines, the development of CpG-ODN spray as a novel therapeutic agent, and the development of CpG-ODN-containing ointments for transdermal applications.