Introduction
Pork belly has the highest fat content among various pork cuts and is highly preferred by consumers in some countries (Albano-Gaglio et al., 2024; Jo et al., 2023; Munezero and Kim, 2023). Pork belly consists of various muscle and intermuscular fat layers (Jeong et al., 2024; Jo et al., 2022) and has different characteristics depending on its location (cranial, caudal, dorsal, and ventral sides) in the muscle and fat layers (Albano-Gaglio et al., 2024; Lee et al., 2018).
Fat in pork belly is important for sensory qualities such as flavor, texture, and juiciness, and for processing properties such as firmness (Ahammad and Kim, 2024; Jo et al., 2024; Kim et al., 2023). Therefore, pork belly with low fat content may have poor quality. However, the high fat content of pork belly is also a concern for consumers because of its high calory and saturated fatty acid content (Gaffield et al., 2022; Lee et al., 2023; Seo et al., 2023). In addition, the high fatness in pork belly reduces the processing yield because thick fat layers are generally discarded during processing. Therefore, information on the fatness of pork bellies can be helpful for the evaluators of carcass grades, producers, and consumers. In particular, information about the fatness of pork belly located in the region from the 10th to 14th thoracic vertebrae (TV) may be more important because of the high fat content in these pork belly slices (Lee et al., 2018; Trusell et al., 2011).
Various factors such as genotype (commercial pigs with crossbreeds, pure breed pigs), sex (male, female, physical, or immune castration), and diet (high energy intake, fat sources) have been reported to influence the fatness of carcasses, and consequently the fatness of pork cuts (Albano-Gaglio et al., 2024; Duziński et al., 2015; Font-i-Furnols et al., 2023; Gaffield et al., 2022; Harsh et al., 2017; Overholt et al., 2016). The results of previous studies may imply that owing to the effects of the various factors described above, changes in the fatness of pork carcasses are accompanied by the changes in the fatness of pork cuts. Hot carcass weight (HCW) and back fat thickness (BFT) of pork carcasses are generally used to predict carcass fatness (Duziński et al., 2015; Harsh et al., 2017; Ko et al., 2023). Previous studies have reported that the pork belly firmness is positively correlated with the HCW of pork carcasses, which is positively correlated with the pork belly fatness (Albano-Gaglio et al., 2024; Harsh et al., 2017). In addition, Uttaro and Zawadski (2010) reported a high correlation (r=0.86) between BFT and the pork belly fat content. However, the relationship between HCW, BFT, and pork belly fatness, particularly the fatness of belly slices from different locations, has not been sufficiently reported.
Therefore, in this study, we measured the fatness (v/v) of whole pork belly and belly slices from different locations. Additionally, we investigated the effects of HCW and BFT on the fatness of pork belly. Furthermore, the differences in the fatness of belly slices between barrows and gilts were investigated.
Materials and Methods
The pork belly was obtained from pigs (Landrace×Yorkshire×Duroc) raised and slaughtered in commercial systems. Therefore, the rearing environment, diet, and age were not considered as factors affecting the fatness of pork belly in this study. Pork belly was procured from the left half- carcasses of 50 barrows (surgically castrated) and 50 gilts 24 h postmortem; a total of 100 pork bellies were used for this study. Pork bellies were collected in 10 batches (10 pork bellies per batch). The HCW values were measured automatically during the slaughter process. The BFT was measured manually at two sites, between the 11th and 12th TV and between the last TV and the first lumbar vertebra (LV), and the mean values of the two sites were used. The half-carcass was vertically cut from the dorsal to the abdominal area at the positions of the 5th TV and 6th LV, and divided into the front leg, body, and hind leg 24 h postmortem. Subsequently, the pork belly was separated from the body after deboning. The skin and subcutaneous fat of the pork belly were removed, leaving 3 mm of fat. The pork belly was vacuum-packed and transported to the laboratory under refrigeration at 4°C.
The fat content of pork belly was first measured on the whole pork belly using computed tomography (CT). Then the pork belly was sliced and fat content was measured on the pork belly slices at selected locations using hyperspectral image analysis. To select the location for measuring the fat content of the pork belly slice, the pork belly was divided into three groups (5th–10th TV, 10th–14th TV, and 1st–6th LV) based on the fat distribution and fac content identified through animal muscle atlas (Korea Institute for Animal Products Quality Evaluation, 2024) and previous studies (Lee et al., 2018; Trusell et al., 2011). In the first and third groups, the 6th TV and 4th LV were selected as representative samples respectively. The 10th–14th TV groups were all selected because they were considered important information to consumers due to their high fat content.
The total fat content (v/v) of whole pork belly was measured using CT. The pork belly was positioned with the muscle part downward and scanned from the cranial to the caudal side using a 32-detector-row CT scanner (AlexionTM, Toshiba Medical Systems, Tochigi, Japan). The scan parameters were 120 kVp, 150 mA, slice thickness of 1 mm, rotation time of 0.75 s, and collimation beam pitch of 0.938. The acquired CT images displayed a soft tissue window (window level=40 Hounsfield units, window width=400 Hounsfield units) and were extracted using commercially available software (Xelis, INFINITT Healthcare, Seoul, Korea). The CT images were checked using a picture archiving and communication system. The volume of the muscle and fat in the pork belly in the cross-sectional CT images was estimated using the Vitrea workstation version 7 (Vital Images, Minnetonka, MN, USA).
After a CT scan of the pork belly, the pork belly was vertically sliced from the dorsal side to the ventral side at the positions of the 6th, 10th, 11th, 12th, 13th, and 14th TV and 4th LV (Fig. 1). Seven slices were obtained from each pork belly sample. The fat content (v/v) of the belly slices was measured using hyperspectral image analysis. A hyperspectral image of the belly slice was captured using a snapshot-type Cubert Ultris X20 plus camera (Cubert, Ulm, Germany) in the reflectance mode. Halogen lamps were used as the light source, and images were collected using the CUVIS software (Cubert). The perClass Mira software (perClass BV, Delft, The Netherlands) was used to measure the volume of muscle and fat in the belly slices.
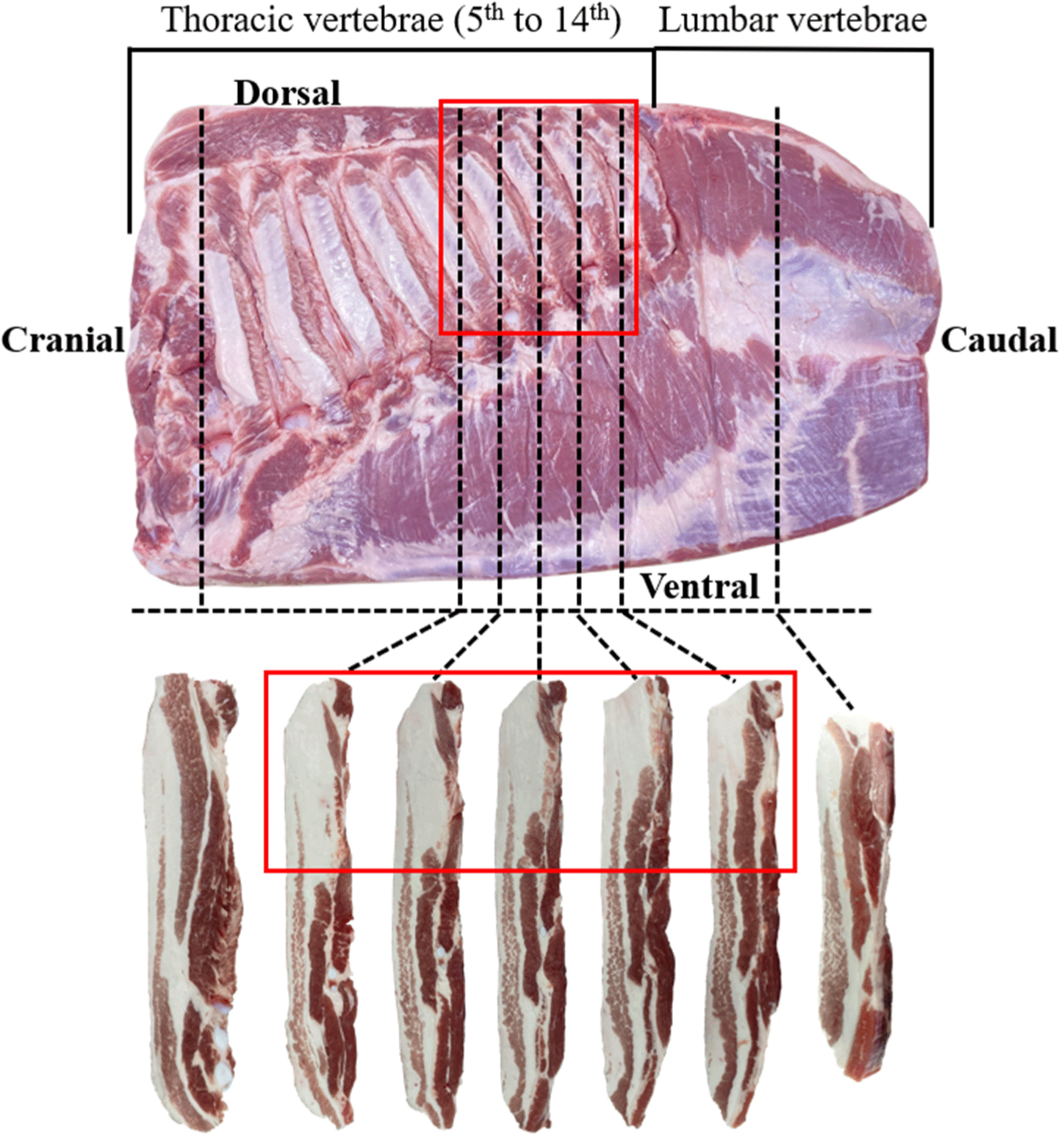
For all data, statistical analysis was performed using the SAS software (version 9.4, SAS Institute, Cary, NC, USA). The descriptive statistics of the carcass properties (HCW and BFT) and the fat contents of pork belly were presented in Supplementary Table S1. The univariate procedure was used to test the normality of the data, which was determined using the Shapiro-Wilk (p>0.05) test. Comparison of pork belly fatness between barrows and gilts was performed using a t-test for normally distributed data and Wilcoxon’s rank sum test for non-normally distributed data. The relationship between continuous data was confirmed using Spearman rank correlation analysis because of the non-normal distribution of some data. The significance of the correlation was set at p<0.05.
Results and Discussion
Carcass properties such as HCW and BFT have been used to monitor the fatness of pork carcasses. In this study, the HCW values for barrow and gilt were 87.67 kg and 89.61 kg, respectively, showing that barrows had significantly lower HCW compared to gilts (Table 1, p<0.05). By contrast, there were no significant differences between the BFT values for barrow and gilt (p>0.05). Previous studies have reported various results for the HCW and BFT differences between barrows and gilts. Overholt et al. (2016) reported that both HCW and BFT were higher in barrows than in gilts. However, Font-i-Furnols et al. (2023) reported no difference in the HCW between barrows and gilts. Moreover, another study found high BFT in barrows compared to that of gilts, whereas barrows and gilts had similar HCW (Bohrer et al., 2023). The differences between our results and the results obtained in previous studies may be attributed to the differences between the carcasses used in each study. However, previous studies have implied that barrow carcasses are generally fatter than gilt carcasses (Knecht and Duziński, 2016; Overholt et al., 2016). Furthermore, the barrow carcasses and gilt carcasses in this study had similar BFT values, despite the lower HCW for the barrows than for the gilts.
The fat content (v/v) of whole pork belly was 37.65% in barrows and 39.20% in gilts, with no significant difference (p>0.05). This result is similar to that of a previous study. Font-i-Furnols et al. (2023) found similar fat contents of minced belly of barrows and gilts with similar HCW. Uttaro and Zawadski (2010) reported that the fat depth measured at the third/fourth last rib in crossbred pork carcasses showed a strong positive correlation (r=0.86) with the fat content of the minced belly, whereas no significant correlation was observed between HCW and fat content of the minced belly. In addition, a weak correlation (r=0.22) between HCW and fat content of the belly measured by CT has been reported (Albano-Gaglio et al., 2024). In this study, the fat content of whole pork belly was moderately correlated with BFT (rs=0.504) and weakly correlated with HCW (rs=0.202; Table 1). Therefore, the fat content of the whole pork belly in this study may be similar for both sexes because of their similar BFT values. In addition, the fat content of all belly slices did not show differences between barrows and gilts.
The fat content of belly slices ranged from 31.65% to 43.77%, and was highest in the belly slice at the 10th TV and lowest in the belly slice at the 4th LV (Supplementary Table S1). This result was similar to that reported by Trusell et al. (2011). They found that the fat content of the pork belly was higher in the middle section than in the other sections when the whole pork belly was divided vertically into five sections between the cranial and caudal. The fat content of the belly slice on the 12th TV was significantly lower than that on the belly slice at 10th TV (p<0.05). The belly slice at the 6th TV showed fat content similar to that of the belly slices at the 12th, 13th, and 14th TV (p>0.05). However, the fat distribution of the belly slices at the 6th TV was different from that of the other TVs (Fig. 1). The fat layer in the belly slice at the 6th TV was evenly distributed from the dorsal to the ventral regions. By contrast, fat accumulated in the dorsal part of the belly slice on the 10th, 11th, 12th, 13th, and 14th TVs (red box in Fig. 1). Trusell et al. (2011) reported that the fat content of the dorsal part of the vertical middle part (similar to the red box in Fig. 1) of the whole pork belly was 75.2%. Therefore, the consumer preference for belly slices at the 10th, 11th, 12th, 13th, and 14th TVs may be low because of the accumulated fat with the small muscle layer. In addition, the removal of the part containing the accumulated fat from the belly slice may damage the producer.
The correlation coefficients (rs) of HCW and BFT with the fat content of the belly are presented in Table 2. HCW had a correlation coefficient of 0.202 with the fat content of the whole pork belly. Albano-Gaglio et al. (2024) reported a similar correlation coefficient (r=0.22) between HCW and the fat content of whole pork belly. The correlation coefficient between BFT and the fat content of whole pork belly was 0.504, which was higher than the correlation coefficient between HCW and the fat content of whole pork belly. A previous study reported that the correlation coefficient for BFT and fat content of pork belly was 0.86 (Uttaro and Zawadski, 2010). Therefore, BFT was more correlated with the fat content of the whole pork belly than HCW. However, HCW and BFT were not significantly correlated with the fat content of all belly slices. In addition, the fat content of whole pork belly showed a weak correlation (rs=0.209–0.325) with the fat content of the belly slices. The fat contents of the belly slices at the 10th, 11th, 12th, 13th, and 14th TVs were strongly correlated (rs=0.801–0.892). However, the correlation coefficients of the fat content of the belly slice at the 6th TV or 4th LV and the slices at the other TVs were lower than those of the belly slices between the 10th and 14th TVs. These results suggest that the fat content of belly slices varies strongly with location. In addition, neither HCW nor BFT can be used to monitor the fatness of belly slices.
Conclusion
This study aimed to determine whether the HCW and BFT of carcasses are related to the fat content of the whole pork belly and belly slices. There was no significant difference in the fat content of pork belly between the barrow and gilt. Pork belly slices had different fat content and fat distribution depending on the location. HCW and BFT had no significant correlation with the fat content of pork belly. In conclusion, it is difficult to monitor the fatness of belly slices at different locations using HCW and BFT. However, this study is important in that it investigated the correlation by considering the difference in pork belly according to the location. Therefore, further research is needed on factors that can monitor the fatness of pork belly, especially considering differences according to the locations of pork belly.