Introduction
The global population is expected to reach 9.9 billion by 2050, up from 7.8 billion today (Population Reference Bureau [PRB], 2020). The British classical economist Thomas Robert Malthus predicted that a food and ecological crisis is inevitable because the population will grow exponentially, but food resources will only grow arithmetically (Prosekov and Ivanova, 2016). Many countries are focusing on how to overcome food shortages to feed their growing populations (Vignesh et al., 2024). A report by the Food and Agriculture Organization predicts that the demand for animal-derived food will reach 550 million tons by 2050 (Sim et al., 2022). As the incomes of countries increase, consumption of animal protein resources increases; for example, the World Health Organization (WHO, 2023) reported that consumption of animal products in France exceeds international requirements and recommendations (Levasseur et al., 2024). Rachmawati et al. (2024) reported that in Indonesia, the national demand for beef reached 680,000 tons in 2019, but production was only 500,000 tons, resulting in a beef supply shortage of approximately 210,000 tons in 2021. Patil (2023) reported that the population is growing at a faster rate than the number of livestock from which meat resources can be obtained, which will lead to a shortage of meat resources in the future.
Global warming and the search for solutions to the climate crisis are among the most prominent global issues in the international community (So, 2023). The 20th century experienced the strongest warming trend in the last millennium, with an average temperature increase of approximately 0.6°C, which is expected to increase in the future by 0.1°C–2°C per decade (Muluneh, 2021). Large-scale natural disasters, such as floods, heat waves, and droughts, negatively impact food production and cause direct harm, such as food shortages (Carvalho and Spataru, 2023). Rosinger et al. (2023) reported that flooding has become the most frequent event globally over the past 50 years, and as it destroys cropland, reducing food production and leading to indiscriminate hunting of wildlife to replace food sources, which will increase food insecurity. Sambo and Sule (2024) reported that in Nigeria, approximately 70% of farmers rely on rainfall for farming, the effects of climate change are expected to reduce rainfall, leading to food shortages and hunger.
Global warming has decreased food security (Lee et al., 2024a). Meat consumption in most countries has been increasing since the 1960s (González et al., 2020), and Flint et al. (2023) estimated that global meat consumption reached 328 million tons in 2021 and will increase by approximately 70% by 2050. As the demand for meat continues to grow, conventional livestock farming, which utilizes limited resources such as water and land, is struggling to maintain pace with rising meat prices and increasing consumption (Reis et al., 2020). Kombolo Ngah et al. (2023) reported that livestock farming in Africa accounts for one-third of the world’s livestock but efforts to meet the growing demand for meat are strained because of inefficient and unproductive systems and infrastructure-limited slaughterhouses. Singh et al. (2021) reported that there is a growing demand for alternative protein sources as sustainable solutions to the shortage of meat.
Future protein sources are described using a variety of terms, including meat analogs and meat substitutes, which are foods that have a similar taste, texture, appearance, and nutritional value to conventional meat but do not contain livestock protein (Sun et al., 2021). Currently, plant-based analog meat, edible insects, and cultured meat are the most representative future protein resources, with plant-based analog meat accounting for the largest share of this market (You et al., 2020). The main ingredients of plant-based analog meats include soy and wheat proteins, peas, soybeans, sesame seeds, peanuts, cottonseed, and rice (Kurek et al., 2022). However, most plant-based analog meats do not resemble the organoleptic properties of meat, such as its flavor and texture, and therefore require improvement (Godschalk-Broers et al., 2022).
Consumers tend to prefer analog meats that can be cooked and mimic the organoleptic properties of conventional meat (Kim et al., 2024a). Therefore, an important aspect of developing future protein resources is selecting suitable protein raw materials (Mishal et al., 2022). Various studies are being conducted to develop analog meat with improved organoleptic properties, such as burgers made from soy protein, and to evaluate consumer impressions of cultured meat (Milani and Conti, 2024; To et al., 2024). Consumers tend to choose analog meat because of their desire for a healthy diet (Arora et al., 2023). Compared with animal-based protein sources, plant-based protein sources are lower in fat and calories and contain polyphenols and other bioactive substances not found in animal products (Cho and Ryu, 2022).
Global sales of analog meats exceeded $10 billion in 2018 and are expected to increase to $21.23 billion by 2025, reaching $30 billion by 2026 (Xie et al., 2024b). Vural et al. (2023) conducted a study of analog meat acceptance among meat-eating and vegetarian consumers and reported that promoting analog meat as a healthy option could expand the consumption market. Analog meat will primarily benefit consumers who cannot eat traditional meat because of religious beliefs, particularly those with halal and kosher practices (Lee et al., 2020a).
This review describes the types and characteristics of future protein resources, raw material characteristics, current status, institutional challenges, and prospects for sustainable food that can replace conventional meat in the current situation of food shortage due to population growth and global warming.
Cultured Meat
Cultured meat is meat made from in vitro muscle cells that have been grown using stem cells harvested from animals (Bhat and Fayaz, 2011). The production process of cultured meat is shown in Fig. 1. Because regulations are less strict for cultured meat than for cell culture in medical research, developing a safe and efficient large-scale production system can reduce production costs (Zhang et al., 2020). Cultured meat must have characteristics that ensure its naturalness and nutritional value, similar to conventional meat, which can be achieved by altering the culture conditions to optimize the biochemical composition of cells comprising the cultured meat, such as replacing unhealthy saturated fats with healthy omega-3 fatty acids or increasing their content (Chen et al., 2022a; Post, 2012). In addition, the composition and quality of cultured meat can be controlled by altering flavor, fatty acid composition, fat content, or other health-promoting and functional ingredients (Arshad et al., 2017).
Cases of producing cultured meat using various growth factors and various cell types are shown in Table 1. Muscle satellite cells are stem cells found between the myoma and the basal plate, which are normally in a dormant state. When the muscle is traumatized or damaged by external stimuli such as exercise, they divide and differentiate into myotubes, which develop into muscle fibers and play an important role in muscle regeneration (Oh et al., 2023b). However, because muscle satellite cells undergo cellular senescence with a limited number of in vitro divisions, large-scale cultured meat production requires a continuous supply and consistent quality of muscle satellite cells (Skrivergaard et al., 2023). Kim et al. (2023b) reported that to collect satellite cells of consistent quality in sufficient yield, it is necessary to consider several factors such as the donor sex, age, breed, and disease status. Oh et al. (2022) reported that when chicken muscle satellite cells were cultured in lineage culture for 6 days, the cells stopped differentiating because of the limited number of divisions; among the chicken muscle cells, breast satellite cells were less capable of differentiating than were leg satellite cells, suggesting that differentiation capabilities vary by site even within the same breed.
Growth factors | Cells | Topics | References |
---|---|---|---|
rAlbumin | Small satellite cells | Establishing an effective protocol for establishing bovine satellite cells by adding recombinant albumin instead of serum | Stout et al. (2022) |
Insulin-like growth factor, fibroblast growth factor, transforming growth factors, platelet-derived growth factor | Fish embryonic stem cells | Development of serum-free media reduces global warming potential, production costs, and optimizes cell growth rates | Nikkhah et al. (2023) |
Chlorella vulgaris extract | Bovine myoblasts | Establish a sustainable culture system free of animal serum and grain-derived nutrients | Yamanaka et al. (2023) |
C. vulgaris extract | Bovine myoblasts | Identifying the potential of C. vulgaris to establish an environmentally friendly cultured meat production process | Okamoto et al. (2022) |
Fermented soybean meal, edible insect hydrolysate | Porcine muscle stem cells | Determine proliferation and differentiation capacity and potential as a fetal human serum substitute | Kim et al. (2023a) |
Rapeseed protein isolate | Small satellite cells | Produce media with rapeseed protein isolate to replace albumin, serum to reduce media costs | Stout et al. (2023) |
Insect hydrolysate, marine invertebrate, hydrolysates | Fish embryonic stem cells | Evaluating the potential of black soldier flies, crickets, oysters, mussels, and midges as serum substitutes for aquaculture fish production | Batish et al. (2022) |
C-Phycocyanin | C2C12 | Evaluate the efficiency of mass production with C-phycocyanin to optimize production processes and costs | Park et al. (2021b) |
Egg whites, eggshell membrane, poultry residue, pea hydrolysate, porcine plasma, fibroblast growth factor | Mammalian cells | Environmental impact assessment of fetal bovine serum media versus serum replacement media | Wali et al. (2024) |
Fibroblast growth factor 2 | Small satellite cells | Identify signaling pathways that promote cell proliferation to develop serum-free media that stimulate satellite cell growth | Yu et al. (2023) |
Egg white extract | Chick satellite cells | Develop an efficient egg white extract preparation protocol to replace fetal human serum | Lee et al. (2024b) |
Lupin, peas, rapeseed, birds, yeast | Fish satellite cells | Validated the feasibility of supporting mackerel satellite cell growth in media with reduced production costs using agricultural waste and low-cost raw materials | Lim et al. (2024) |
Chlorococcum littorale | C2C12 | Develop a sustainable circulating cell culture system that nourishes cells and allows waste media to be recycled | Haraguchi et al. (2022) |
Auxenochlorella pyrenoidosa | Fish muscle cells | Evaluating the potential of A. pyrenoidosa to promote cell proliferation and mass production in goldfish | Dong et al. (2023) |
Fermented Okara | C2C12 Pig immortalized myoblasts | Identifying Okara’s potential as a serum substitute | Teng et al. (2023) |
Anabaena sp. PCC 7120 | C2C12 QM7 | Evaluation of the potential of Anabaena sp. PCC 7120 extract as an inhibitor of cell proliferation and growth in medium supplemented with algae extract | Ghosh et al. (2023) |
Embryonic stem cells, which are pluripotent stem cells, are derived from the endoderm, mesoderm, and ectoderm of the embryo, can be isolated from the inner cell mass of the preimplantation blastocyst, and can proliferate unrestrictedly and differentiate into various cell types (Kulus et al., 2023). Skeletal muscle, extracellular matrix, microvasculature, and intramuscular fat are required to recreate the structure of a carnivore, and given the variety of cells required, embryonic stem cells rather than satellite cells from adult animals should be used (Hadi and Brightwell, 2021). Bogliotti et al. (2018) harvested, expanded, and cultured bovine embryonic stem cells and reported that embryonic stem cells are suitable for long-term culture because they proliferate with a stable karyotype and increase in number over time. However, the short lifespan of blastocysts makes it difficult to harvest embryonic stem cells, and the lack of protocols for differentiating and culturing embryonic stem cells necessitates the development of a versatile cell source with high proliferation and yield (Reiss et al., 2021).
Fetal bovine serum (FBS) is a growth-promoting supplement derived from the fetuses of slaughtered pregnant cows and is rich in hormones, antibodies, growth factors, and amino acids, making it a popular choice for cell culture techniques (Lee et al., 2022c). FBS is highly effective for promoting cell attachment, growth, and maintenance (Kim et al., 2023a); however, Andreassen et al. (2020) reported that the cost of serum can account for approximately 95% of the total cost of cell culture media, which contributes to the high price of cultured meat (Celebi-Birand et al., 2023). The price of FBS has increased by approximately 300% in the past few years, but cell culture still relies on FBS (Lee et al., 2024b). To effectively achieve the industrialization of cultured meat, it is necessary to mass produce cultured meat at a low cost, so research is underway to produce sustainable serum replacement media using microalgae, egg whites, rice, and wheat, among other materials (Flaibam et al., 2024; Park et al., 2023a).
To produce serum replacement media for developing cultured meat, insulin-like growth factors (IGFs) have been used as growth-promoting supplements that effectively replace serum because they have a similar structure to serum media (Trinidad et al., 2023). Two types of IGFs, IGF-1 and IGF-2 are observed, which are important in cell proliferation, growth, and maturation and have an insulin-like structure (Venkatesan et al., 2022). IGF-1 promotes both the proliferation and differentiation of myoblasts, which is signaling mediated through two pathways, the PI3K/Akt and MAPK/ERK1/2 pathways (Yu et al., 2015). Ahmad et al. (2023) reported that IGF-1 activates the proliferation of muscle satellite cells and plays an important role in the regeneration and formation of muscle, and in a study of myoblast proliferation in chickens, the number of myoblasts increased as the dose of IGF-1 was increased, suggesting that IGF-1 is an important contributor to cell proliferation and regeneration.
C-Phycocyanin is a water-soluble photosynthetic pigment-protein derived from the blue microalga Spirulina, which is widely used as a nutritional supplement (Rahim et al., 2024). Microalgae have 5–10-fold higher biomass productivity and 15-fold higher carbon dioxide fixation capacity compared with plants; thus, using microalgae can overcome the ethical issues and unstable supply caused by FBS and realize carbon neutrality (Yamanaka et al., 2023; Yoo et al., 2020). Park et al. (2021a) reported that C-phycocyanin performs DNA repair, antiviral, and antioxidant activities in cell culture, and based on these activities, in the development of cell sheets using fish gelatin powder, cell sheets containing 5% FBS with added IGF-1 and C-phycocyanin were more effective in inhibiting cell senescence compared with cell sheets containing 10% FBS. Levi et al. (2022) suggested that reduced serum use can help industrialize the production of cultured meat by making it low-cost and sustainable.
Although antibiotics are used in conventional livestock farming to improve livestock growth, cultured meat does not use antibiotics during the cell culture process, thus avoiding the presence of antibiotic residues and resistance that occurs when consuming meat (Munteanu et al., 2021). Cultured meat is free from consumer health concerns because of the lack of genetic manipulation and the ability to flexibly control the fat content (Bryant and Barnett, 2018; Rolland et al., 2020). However, consumers distrust biotechnology-enhanced foods, which can negatively affect their purchasing behavior (Hwang et al., 2020). Omnivores that consume a wide variety of plants and animals, such as humans, have food neophobia, which is a reluctance to try new foods, but if the nature of the new food is clear in terms of its benefits to society or the individual, food neophobia can be mitigated to increase acceptance (Siddiqui et al., 2022). The main remaining challenges for cultured meat are to scale up the size of cultured meat tissues to that of real meat, with large-scale industrial facilities for mass production with low production costs (Liu et al., 2022), and to scale up and sustain the cultured meat industry while reducing its environmental impact by extracting and developing new cells capable of mass multiplication and non-animal bioinks to help cells survive (Albrecht et al., 2024; Kamalapuram et al., 2021). Another important issue is that genetic modification during the cultured meat production process and food safety certification of cultured meat ingredients that have not yet been accepted are considered risk factors (Zhang et al., 2020). Verbeke et al. (2015) reported that consumers responded positively to cultured meat in terms of its global potential to solve hunger problems in developing countries with insufficient nutritional intake, but they were afraid of cultured meat due to concerns about the ‘unnaturalness’ and potential risks of genetic modification. Regulatory systems such as food safety certification should be promoted rapidly in proportion to the public benefits, even at the cost of potential risks for environmentally and socially sustainable foods (Manning, 2024). Therefore, for cultured meat to be effectively commercialized, it is considered necessary to expedite the development of regulatory systems such as food safety certification and quality control.
Edible Insect
People began consuming insects as food approximately 7,000 years ago; of the more than 2,300 reported species of edible insects, the Diptera, Lepidoptera, Coleoptera, Hymenoptera, Coleoptera, Diptera, Termitidae, Diptera, and Lepidoptera are the most common (Liang et al., 2024; Tang et al., 2019). Approximately 30% of the world’s population consumes edible insects, mainly in Africa, Asia, and Latin America (Raheem et al., 2019). The production of edible insects is environmentally friendly, as water and land use are minimal compared with those used by conventional livestock, and insects show excellent biomass conversion rates because of their easy technology and fast growth rates, enabling a stable food supply for the growing population (Gravel and Doyen, 2020; Pal et al., 2024). In addition, insects with high feed conversion rates require less feed than cattle, pigs, and chickens to produce 1 kg of animal protein, and the carcasses account for a large proportion of the body mass, making them a promising future protein resource (Moruzzo et al., 2021). In Korea, Oxya chinensis sinuosa, Bombyx mori (larva, pupa), Bombycis corpus, Tenebrio molitor (larva), Gryllus bimaculatus, Protaetia brevitarsis (larva), Allomyrina dichotoma (larva), Zophobas atratus (larva), Apis mellifera (pupa), and Locusta migratoria are listed as edible insects that can be used as food ingredients, among which Z. atratus (larva), A. mellifera (pupa), and L. migratoria are listed as limited food ingredients (Cho, 2023). Jang et al. (2022) reported that rice cookies containing T. molitor larva, G. bimaculatus, and P. brevitarsis larva powder showed higher values of ABTS and DPPH radical scavenging activities compared to the control without insect powder; additionally, in sensory evaluation, rice cookies containing 5 g of G. bimaculatus powder showed higher values for taste, texture, and overall palatability than the control, suggesting the potential of using insects as food ingredients.
The general composition of domestic edible insects is summarized in Table 2. Among the general components of dried edible insects, moisture content and protein content were the highest in O. chinensis sinuosa (8.70% and 74.28%), fat content was the highest in Z. atratus (36.30%), and ash content was the highest in P. brevitarsis (8.36%; Baek et al., 2017; Kim et al., 2017; Kim et al., 2019a; Wedamulla et al., 2024). T. molitor, which has a high sales volume among domestic edible insects, is suitable for replacing fish meal in feed because of its high protein and lipid content and abundant essential amino acids such as methionine (Kim et al., 2024b; Shafique et al., 2021). This species contains high-quality protein with a balanced content of essential fatty acids and amino acids and higher calcium and iron contents than in cattle, pigs, and chickens (Pan et al., 2022). Daily consumption of iron-rich insects can help prevent anemia, which is common among preschoolers and pregnant women in developing countries (Zielińska et al., 2015). Most insects are rich in unsaturated fatty acids, which have health benefits for humans in reducing the risk of cancer and cardiovascular disease and improving blood sugar (Zhou et al., 2022). In addition, it has various physiological functions such as anti-obesity, anti-inflammatory, and anti-tumor, with fewer side effects compared to drugs.They are rich in bioactive substances with various functions such as anti-obesity, anti-inflammatory, and anti-tumor effects, and have fewer side effects than drugs, there is a negative perception among consumers regarding consuming insects, and thus they must be extracted or powdered in the form of additives to reach consumers (Zhang et al., 2024).
Insect species | Moisture | Protein | Fat | Ash | References |
---|---|---|---|---|---|
Oxya chinensis sinuosa | 8.70±0.10 | 74.28±0.61 | 3.03±0.15 | 4.40±0.06 | Kim et al. (2017); Wedamulla et al. (2024) |
Bombyx mori | 7.92±0.98 | 20.79±2.22 | 17.57±1.15 | 6.34±0.84 | Omotoso (2015); Wedamulla et al. (2024) |
Tenebrio molitor | 2.90±0.04 | 50.32±0.21 | 33.70±0.13 | 3.73±0.03 | Baek et al. (2017) |
Gryllus bimaculatus | 3.86±0.23 | 61.05±1.06 | 19.08±0.16 | 4.41±0.60 | Kim et al. (2020a) |
Protaetia brevitarsis | 6.66±6.40 | 57.86±0.01 | 16.57±1.81 | 8.36±0.10 | Baek et al. (2017) |
Allomyrina dichotoma | 1.63±1.42 | 39.31±1.34 | 25.21±5.02 | 5.26±1.75 | Baek et al. (2017) |
Zophobas atratus | 1.30±0.64 | 52.2±1.29 | 36.30±0.43 | 3.6±0.02 | Kim et al. (2019a) |
Apis mellifera | 8.68±0.17 | 45.70±0.85 | 24.98±0.12 | 3.66±0.19 | Mekuria et al. (2021) |
Locusta migratoria | 0.90±0.40 | 69.80±0.30 | 14.30±1.20 | 3.20±0.03 | Kim et al. (2020b) |
To effectively use edible insects as a protein source, it is important to remove indigestible material such as chitin, which makes up the exoskeleton, and extract the protein (Kim et al., 2019b). Commonly used methods for protein extraction include degreasing, sonication, and dissolution in alkali solution followed by isoelectric precipitation and enzymatic hydrolysis (Mishyna et al., 2021). Degreasing is important during the manufacturing process because it can inhibit off-flavors caused by lipid oxidation in insects that are rich in lipids (Gkinali et al., 2022). This process reduces the lipid content and increases the protein content, and is typically performed using non-polar solvents such as hexane and acetone or polar solvents such as ethanol (Jeong et al., 2021). Amarender et al. (2020) reported that ethanol was effective for extracting lipids from crickets with hexane and ethanol, suggesting that organic degreasing solvents can be used as an alternative to the environmental and health threats posed by residual hexane in food (Kim et al., 2021). Ultrasonication activates protein enzymatic degradation reactions through cavitation caused by shock waves and vibrations, which increases protein yield and improves the structure and safety of the reaction products (Mintah et al., 2019). Choi et al. (2017) reported that sonication increased the protein yield by 34% and 28% after 15 min of sonication in cricket and mealworm pupae, respectively, and by 76% after 5 min in silkworm pupae, indicating that sonication increased the protein yield. Other methods of protein extraction, such as dissolution in alkali followed by isoelectric precipitation and enzymatic hydrolysis, are time-consuming and require significant amounts of energy and water; therefore, eco-friendly and more efficient ultrasonication with a shorter process time is widely used (Pinel et al., 2024; Zhang et al., 2023b).
An example of edible insects use is shown in Fig. 2. Edible insects can be used in a variety of ways, including use in traditional cooking methods (frying, baking, steaming) or processing into additive forms (powders, oils) to be added to foods to make products (bread, biscuits, pasta, tortillas; Mancini et al., 2022; Skotnicka et al., 2021). In China’s multi-ethnic Yunnan province, several species of edible insects exist, and ethnic minority residents commonly consume insects whole, fried, or cooked, including Antheraea pernyi pupae, moth cakes, cricket jam, and ant egg salad (Xie et al., 2024a). In Western countries, where there is still resistance to eating insects, insects are being added to baked goods in powdered form to increase their nutritional value, including fiber, protein, and minerals (Borges et al., 2022). In Korea, Flora Umi Tsukumi restaurant serves pizza and pasta with edible insects, and Grub Kitchen in the UK sells bolognese, burgers, and cookies made with edible insects (Han et al., 2017; Hwang and Kim, 2021). The Swiss company Essento has launched edible insect protein bars, snacks, and burger patties that focus on sustainability based on a nutritional and environmental ideology as well as the packaging and appearance of the products (Daub and Gerhard, 2022). Insects are rich in unsaturated fatty acids, which can meet essential fatty acid requirements and can be utilized in animal feeds as an alternative source of polyunsaturated fatty acids (Kolobe et al., 2023). Rumpold and Schlüter (2013) reported that feed accounts for 70% of the cost of producing livestock and replacing fish meals with larval meals in poultry diets resulted in similar gain and growth rates as fish meal-supplemented diets, suggesting that insects can replace costly fish meals as a protein source.
Proteins from edible insects have a lower molecular weight than do conventional meat proteins, making them easier to digest and absorb (Lee et al., 2023a). Lee et al. (2020b) reported that the in vitro protein digestibility of P. brevitarsis larvae was 4.33% higher than that of beef tenderloin. Furthermore, Hammer et al. (2023) reported that the digestibility of Acheta domesticus and T. molitor larvae and chicken were similar, demonstrating their potential as meat substitutes. Insects have medicinal properties and have been used as entomotherapy in the form of extracts or ointments since ancient times (Devi et al., 2023). According to Zhang et al. (2023a), bee products (honey, propolis, royal jelly, and beeswax) are used as folk remedies for conditions such as colds, wounds, and sore throats, and ant and bee venom is used to treat rheumatoid arthritis, an autoimmune disease. Just like microorganisms and plants that have been used as drugs, insects are also rich in active ingredients for use as drugs and have anticancer properties, so they could be one of the drug resources with medical value that can help humans safely avoid diseases (Chen et al., 2022c).
Żuk-Gołaszewska et al. (2022) estimated that the edible insect market is worth approximately KRW 600 billion in Korea, and in the EU, 260,000 tons of edible insect food is expected to be produced by 2030, reaching a value of approximately KRW 3 trillion. The use of edible insects as a protein source, food, feed additive, and medicine is increasing globally, and to meet this demand, mass production is needed, but scaling up insect production requires significant facilities and costs to build automation systems that can reduce labor, waste treatment facilities, and other components (Siddiqui et al., 2023). Tang et al. (2019) reported that the establishment of a collaborative system between farms and industry would improve productivity by increasing cultivation efficiency, with the additional benefits of developing insects as health supplements and medicines. In addition, the insect farming industry, which is still in its early stages, could increase regional income and create employment opportunities in response to the demand for large-scale production (Tang et al., 2019). Industrialization of edible insects requires cooperation at the national level, which will enable solving the problem of future protein resource shortages and coexistence and development with local communities.
Plant-Based Meat Analogues
Plant-based analog meat is made by extracting proteins from plants to produce a meat-like taste, form, and texture (You et al., 2020). Plant proteins are suitable as a future protein source because they are inexpensive, have a high protein content, and provide a balanced amino acid profile, and wheat gluten, soy protein, and others are commonly used to make plant-based analog meats (Joshi and Kumar, 2015). Since the mid-1900s, manufacturing techniques using plant-based proteins have evolved, with tofu and tempeh prepared using wheat gluten and soybeans to create a meat-like texture, and now fungi (mushrooms, yeast, mycoproteins) and legumes (lupins, chickpeas, etc.) are currently being used to create analog meat (Bohrer, 2019; Zahari et al., 2022). Various types of plant-based analog meat are being developed such as sausages, steaks, nuggets, and patties in response to consumers preference for meat-like texture and organoleptic properties, and is being manufactured by adding soy protein, pea protein, gluten, potato protein, and other proteins with emulsifying and water-holding properties like fiber protein in meat (Kyriakopoulou et al., 2021). In addition, binders, flavor enhancers (fats, oils, etc.), and colorants are often added during the manufacturing process of plant-based analog meat to give it a meat-like texture, flavor, and color (Tang et al., 2024). The first patent for soy protein was issued in the U.S. in 1955, and the market has grown steadily since 1960, with France, Germany, Italy, and the U.K. currently leading the analog meat market, and in Spain, sales of plant-based analog meat, yogurt, and milk increased by approximately 20% between 2021 and 2022 (Costa-Catala et al., 2023). Melville et al. (2023) reported that the market for plant-based analog meat products is growing significantly as a sustainable food because of environmental concerns such as water scarcity and greenhouse gas emissions, along with health concerns such as diabetes and cardiovascular disease.
The types of proteins and their pros and cons mainly used to produce plant-based meat analogues are shown in Table 3. With approximately 350 million tons of soy produced annually, soy shows a high potential for providing a reliable source of protein for the growing population (Messina et al., 2022). Soy has a high nutritional value based on its rich content of essential amino acids (except methionine) and isoflavones involved in bone health and blood pressure regulation and is widely consumed because of its low cost; the number of food products containing soy protein has steadily increased, currently exceeding approximately 10,000 (Cai et al., 2021; Zhu et al., 2020). Plant-based analog meat made from soybeans is low in fat and calories and is cholesterol-free, which has beneficial health effects, including cholesterol-lowering effects and preventing low blood pressure and obesity (Bakhsh et al., 2022). Caponio et al. (2020) reported that the peptide IAVPGEVA (Ile-Ala-Val-Pro-Gly-Glu-Val-Ala), which is obtained when soybeans are hydrolyzed, reduces cholesterol in the blood; in a clinical trial in which patients with hypercholesterolemia consumed a diet containing soy protein for one month, blood cholesterol levels decreased by 123 mg/mL, demonstrating the suitability of soy protein as a functional food. Kang et al. (2022) compared chicken sausage and sausage with soy protein and observed that sausage with soy protein had a more stable structure than did chicken sausage because of the improved emulsification due to water-soluble proteins in soybeans, and a softer texture because of improved water retention and heating yield due to the stable structure, suggesting that soy protein can replace meat in various products.
Plant protein | Pros | Cons | References |
---|---|---|---|
Wheat gluten | - Low price - High protein content - Widely used as composite agent to improve fiber structure |
- Not soluble in water - When applied to meat products, chewiness is reduced due to low water retention capacity - May cause allergic reactions |
Bogueva and McClements (2023); Sun et al. (2024); Zhang et al. (2023c) |
Soy protein | - High water absorption and water holding capacity - Good gelling properties - Low price |
- Rejection due to the smell of soybeans - Side effects on masculinity when consumed excessively (infertility, erectile dysfunction) - May cause allergic reactions |
Bogueva and McClements (2023); Lee et al. (2022b); Schreuders et al. (2019); Sun et al. (2024); Zhang et al. (2021a) |
Pea protein | - Less associated with genetic manipulation - Not subject to allergen labeling |
- Lower gelling ability than soy protein - May cause allergic reactions |
Bogueva and McClements (2023); Schreuders et al. (2019) |
Peanut protein | - Low in anti-nutritional factors - Excellent amino acid profile |
- Poor gel and emulsification properties - May cause allergic reactions |
Boukid (2022); Zhang et al. (2023c) |
Rice protein | - No unpleasant taste - Hypocholesterolemic - Highly digestible compared to wheat gluten |
- Requires supplementation with soy protein due to limiting amino acid (lysine) | Cho and Ryu (2022); Lee et al. (2022a) |
Mung bean protein | - High content of functional substances (flavonoids, etc.) - High digestibility - Better gelling properties than soy and pea proteins |
- Characteristics vary depending on protein extraction method, salt concentration, pH, etc. - Hard and cohesive structure, resulting in lower gelation and surface properties than egg protein |
Cho and Ryu (2022); Feng et al. (2024); Hwang et al. (2023); Wang et al. (2022b) |
Potato protein | - Good foaming and emulsifying properties - Highly soluble - High digestibility - Nutritionally similar to animal protein |
- Gluten-free, difficult to form gel | Kumar et al. (2022); Lv et al. (2023); Okeudo-Cogan et al. (2024) |
Mycoproteins | - Similar to meat in nutritional value, fiber texture, flavor, and taste - High digestibility fibrous texture - Sustainable protein source |
- Iron content is about 35% or less of meat | Kumar et al. (2022); Okeudo-Cogan et al. (2024) |
Mushroom protein | - High protein content (23.80 g per 100 g) - Fast yield - High thermal and pH stability - Contains branched chain amino acids (leucine, isoleucine, valine) like animal proteins |
- Due to the high fiber content, the Maillard reaction may occur during digestion, resulting in a decrease in essential amino acids (lysine, methionine, tryptophan) - Phenolic substances and tannins contained in mushrooms inhibit digestive enzymes |
Ayimbila and Keawsompong (2023) |
Wheat gluten is the protein component that is isolated by kneading wheat flour with water to remove non-protein components and starch and has high viscoelasticity. Baking, noodles, pasta, and other products have been produced using wheat gluten (Schopf et al., 2021; Shewry, 2019). Wheat gluten, which is responsible for protein storage in wheat, is composed of glutenin and gliadin, which increase viscosity and softness and are added during the production of analog meat to improve texture (Zhang et al., 2023c). However, Sun et al. (2024) showed that wheat gluten is difficult to apply to analog meat production because of its low solubility and water retention; thus, pretreatment combining pH cycling and heat treatment was used to increase the solubility and water retention of wheat gluten to improve the texture of analog meat. Hou et al. (2023) examined the production of analog meat using white pollock fillets with high gel strength, wheat gluten, and soy protein and reported that increasing the content of wheat gluten made the fiber structure of the analog meat clearer and increased its elasticity, whereas excessive addition decreased the elasticity, chewability, and fiber structure, determined the appropriate ratio of wheat gluten and soy protein required to produce analog meat.
Edible fungi, also known as mushrooms, are human-edible macrofungi with highly palatable textures, tastes, and flavors that can be used as food and medicine (Wei et al., 2022). More than 2,000 species of mushrooms worldwide can be consumed by humans. Agaricus spp., Pleurotus spp., Lentinula edodes, and Ganoderma spp. are cultivated commercially as edible mushrooms in their raw form or as products (Mahari et al., 2020). Mushrooms are mainly harvested following cultivation or from the wild, have high yields because of their fast growth rate, and can grow in small spaces, making them a sustainable food (Pérez-Montes et al., 2021). Mushrooms are rich in bioactive substances such as proteins, peptides, vitamins, polysaccharides, polyphenols, flavonoids, saponins, and terpenoids, and are considered a health food based on their antioxidant, antibacterial, and antiviral properties (Sun et al., 2020). Yan et al. (2023) reported that mushrooms can be used as food preservatives to maintain freshness, liquid fermentation products with unique flavors and tastes that can be added to food and beverages as flavor enhancers, or as analog meat using monosodium glutamate, which is similar in taste to the amino acids in mycelium and meat. The mycelium, the lower part of the mushroom, is mainly composed of protein, cellulose, and chitin and has a rich protein content, and thus can be used as livestock feed or as a substitute for drugs, flour, and meat (Zhang et al., 2021b). Mycoprotein, a protein and fungal mycelium made from a fibrous fungus, the mushroom fungus, is a food-grade fungus and high-protein source, and the British company Marlow Foods introduced mushroom-based foods under the brand Quorn in 1895 and now sells products such as mycoprotein-based steaks, nuggets, and patties (Park et al., 2023b). Shahbazpour et al. (2021) reported that mycoprotein-added sausages were nutritionally superior to beef sausages because of their higher essential amino acid and unsaturated fatty acid contents, lower carbohydrate and fat contents, and lack of microbial growth after cooking; however, they exhibited lower hardness, springiness, gumminess, and cohesiveness, indicating that further research on additives is needed to achieve an optimal texture.
Vegetable proteins can be made to mimic the fibrous structure of meat using techniques such as extrusion, shear cell technology, and ohmic heating (Jung et al., 2022). A schematic diagram of the extrusion and shear cell technology and ohmic heating system is shown in Fig. 3. Extrusion is a rapid process in which vegetable proteins are subjected to shear forces and pressure at high temperatures to produce a meat-like fibrous structure, with two types of extrusion processes are observed: low-moisture extrusion processing (LMEP), which uses a single-screw extruder to form at moisture contents below 30%, and high-moisture extrusion processing (HMEP), which uses a long cooling die to form at moisture contents above 50%, with HMEP as the most commonly used technology (Cho et al., 2023; De Angelis et al., 2024). Choi and Ryu (2022) compared the physicochemical properties of LMEP and HMEP vegetable analog meats and observed that low-moisture analog meats exhibited a spongy structure because of their large number of internal air layers, whereas high-moisture analog meats exhibited a delicate structure because of swelling-prevented by a long cooling die and the high-moisture analog meats exhibited superior values of chewability, cohesion, elasticity, and tissue residual modulus, supporting the greater utilization of high-moisture extrusion.
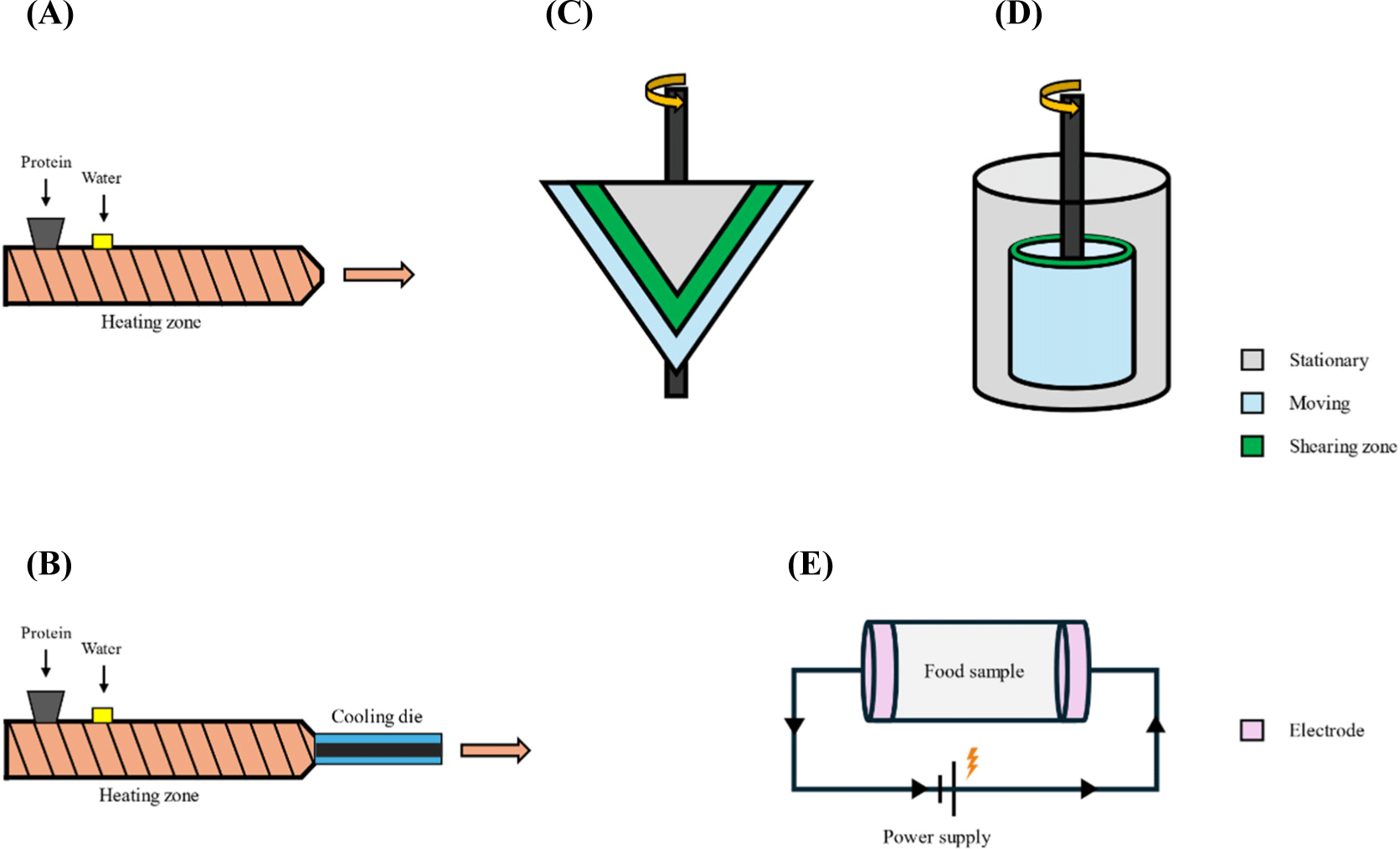
Shear cell technology produces fibrous structures by modifying the flow of shear based on the concept of flow-induced structuring and can produce a variety of product structures by controlling the shear temperature and speed (Nowacka et al., 2023). Two types of shear cells are observed, nested cone-shaped and nested cylindrical couette cells, and when water and vegetable protein raw materials are added to the shearing zone, which exists in the middle between the fixed top cone and outer cylinder and the heated and rotating bottom cone and inner cylinder, the fiber structure is layered by heat and shear force and has a meat-like structure (Nowacka et al., 2023; Su et al., 2024). Krintiras et al. (2015) examined the production of analog meat using soy protein and wheat gluten in a couette cell and showed that the analog meat was produced over a process time of 15 min, a rotation speed of 30 RPM, and a process temperature of 95°C exhibited repeatable and consistent fiber formation throughout, and the anisotropy index was similar to that of meat, the potential of couette cell technology for producing plant-based analog meat.
Ohmic heating, also known as electro-conductive heating, electrical resistance heating, and joule heating, is an electromagnetic-based technology in which an electric current is passed through food to achieve uniform heating (Varghese et al., 2014). Ohmic heating was first used in the United States to pasteurize milk at low temperatures and has since been used to blanch and sterilize foods such as meat, fruits, and vegetables, and has the advantage of avoiding increases in heating time and overheating depending on the characteristics of the food (Jaeger et al., 2016). In addition, Jung et al. (2022) reported that adding pressure to ohmic heating technology, which has a simple temperature control and fast temperature increase rate, can be used to improve the adhesion of vegetable analog meat and realize the appropriate texture of meat. Chen et al. (2023) reported that when ohmic heating was applied in the production of analog meat using peanut protein, a uniform and high-density structure was formed; chewability, cohesion, elasticity, and hardness were improved; texture was enhanced; and volatile substances that produce fatty flavors were increased, indicating that ohmic heating is suitable for enhancing the structure and flavor of vegetable analog meat. Examples of the production of plant-based meat analogs using advanced processing technologies are shown in Table 4.
Technology | Pros and cons of technology | Plant protein | Subject | References |
---|---|---|---|---|
High-moisture extrusion processing (HMEP) | - Pros: Dense fibrous structure - Cons: Short shelf life due to high moisture content (Choi and Ryu, 2022) |
Pea protein, amylose, amylopectin | Analyzing the effect of protein interactions with amylose and amylopectin on fiber structure | Chen et al. (2022b) |
Soy protein | Compare morphological development of analog mittens as temperature changes | Wittek et al. (2021) | ||
Pea protein, peanut protein, soy protein, wheat gluten, rice protein | Comparing the water-binding capacity of protein sources to improve the juiciness of analog meats | Hu et al. (2024) | ||
Pea protein isolate (PPI), pea protein concentrate (PPC) | Compare the quality and organoleptic characteristics of PPI and PPC when mixed with ground beef. | Pöri et al. (2023) | ||
Soy protein, pea protein, wheat gluten | Improve the texture of analog meat and provide quality control techniques | Flory et al. (2023) | ||
Low-moisture extrusion processing (LMEP), HMEP | LMEP - Pros: Easy handling, long shelf life - Cons: Expanded structure with porous layers |
Soy protein, wheat gluten | Comparison of analog mitt chemistry by LMEP and HMEP | Choi and Ryu (2022) |
Shear cell | - Pros: Formation of fibrous structure - Cons: Testing is limited to laboratory scale (Krintiras et al., 2015) |
Soy protein, pea protein, wheat gluten | Comparison of mixing and hydration effects of different protein sources and analysis of how mixing time affects the structure of analog meat | Köllmann et al. (2024) |
Soy protein, pea protein, wheat gluten | Analyze the texture of analog meats made with shear cells with different strengths of vibration. | Giménez-Ribes et al. (2024) | ||
Soy protein | Analyzing how the addition of salt affects the texture and structure of analog meat | Dinani et al. (2023) | ||
Ohmic heating | - Pros: High efficiency in converting electrical energy into heat - Cons: Insufficient research on producing meat analogues (Jung et al., 2022) |
Soy protein, wheat gluten | Analyzing the effect of cooking time and temperature on the texture and physicochemical properties of analog meat during ohmic heating | Jung et al. (2022) |
Peanut protein | Confirming the effectiveness of ohmic heating as a technique to improve the structure and flavor of analog meat | Chen et al. (2023) | ||
Freeze structuring | - Pros: Unique fibrous structure - Cons: High production costs due to high energy consumption (Du et al., 2023) |
Pea protein, wheat gluten | Developing vegetable protein composites with improved nutritional and textural properties using cryostructuring technology | Yuliarti et al. (2021) |
Fiber-spinning | - Pros: Micron-level protein fiber formation - Cons: High requirements for protein solutions, heavy contamination (Wang et al., 2023) |
Soy protein | Developing soy protein-based analog meat with improved nutritional, physicochemical, and structural properties | Joshi et al. (2023) |
3D Printing | - Pros: Control of fiber structure arrangement and distribution of adipose tissue - Cons: Plant-based meat analog inks are difficult to extrude, making it difficult to mimic the texture of animal meat |
Pea protein | Rheology and extrusion testing to develop printable, print process-optimized formulations | Wang et al. (2022a) |
Mung bean protein, wheat gluten | Improving the functionality of mung bean protein, wheat gluten mixtures, and adding L-cysteine to improve the quality and sensory characteristics of analog meat | Chao et al. (2024); Wen et al. (2023) |
Currently, plant-based analog meat lacks fat and flavor, and sometimes has off-flavors and soy flavor, but plant-based oils such as coconut oil and medium chain triglycerides (MCT) oil can be used to express fatty flavors similar to meat; plant-based spices such as pepper, basil, and turmeric can be used to produce analog meat with specific flavors, and enzymatic treatments can be used to suppress soy flavors, and it is necessary to improve the quality of analog meat using these various approaches to gain an advantage in the alternative food market is necessary (Jung et al., 2024; Su et al., 2024). Overseas brands selling plant-based analog meat products include Impossible Food and Beyond Meat, which are popular among consumers because their products reproduce the appearance, flavor, and blood similar to meat, and Impossible Food, which has demonstrated the sustainability and scalability of the plant-based analog meat market with its burger patties containing leghemoglobin extracted from soybean root hairs to create the blood taste of meat (Arora et al., 2023; Muhlhauser et al., 2021). Oh et al. (2023a) reported that Eat Just in the U.S. created powdered artificial eggs to provide a new option for people with egg allergies, and in Korea, Nongshim’s Veggie Garden, CJ CheilJedang’s Plant table, and Shinsegae Food’s Berry Meat brands were launched, expanding the diversity of the domestic plant-based analog meat market by launching tteokgalbi, dumplings, and canned ham using plant-based analog meat. According to Blue Horizon Corporation and Boston Consulting Group, the alternative food market will reach $290 billion before 2040, and the key to growing the alternative food market is to produce analog meat with similar price and organoleptic properties as meat (Maningat et al., 2022). Currently, various plant-based meat analogues are succeeding as future protein resources, and they are expected to expand the market even to consumers who do not consume meat due to ethical or religious beliefs, leading to an anticipated increase in demand for future protein resources (Lee et al., 2020a). Mushrooms are a nutrient-rich source of protein, including essential amino acids, essential fatty acids, vitamins, and minerals, and analog meat utilizing mycelium, which can produce protein more rapidly than the fruiting body, is gaining traction in the food industry as an alternative to the raw materials used in traditional plant-based analog meat or as a plant-based protein that can be mass-produced (Strong et al., 2022). If plant-based analog meat is developed using mycelium, which enables rapid protein production, it is believed that future food security issues can be addressed through the production of future protein resources that have a taste and texture similar to meat.
Future Protein Resource Challenges
In the U.S., new, previously unused ingredients must be evaluated and approved as Generally Recognized as Safe (GRAS) by the Food and Drug Administration (FDA) before they can be used in food production, and the soy rhizobium leghemoglobin used in our plant-based burger patties was evaluated and approved as GRAS before launch, whereas for cultured meat, food safety is regulated by the United States Department of Agriculture-Food Safety and Inspection Service for labeling and processing monitoring, and by the FDA for harvesting cells or tissues (Kołodziejczak et al., 2021; Lee et al., 2023b). Previously in Korea, even if a product did not contain meat, it could be labeled as plant-based alternative meat if it was labeled as “plant-based” or “vegan” thus, the Hanwoo Board issued a statement calling for a ban on the use of the word “meat” and in response, the Ministry of Food and Drug Safety (MFDS) established guidelines for the labeling of alternative foods to prohibit the use of the word “meat” in 2023 (MFDS, 2023; Park et al., 2023b). To commercialize analog meat, it is necessary to reach an amicable agreement with the existing livestock industry to prohibit the use of the term “meat” for cultured meat and plant-based analog meat products, as in 2018, the use of expressions such as “steak” and “sausage” was banned in the U.S. and Europe to prevent misleading consumers by indicating that meat is added to plant-based analog meat products (Lee et al., 2023b).
The choice of protein source to be added to produce analog meat is an important consideration because it affects the organoleptic properties of the finished product, which in turn is directly related to consumer acceptance of the meat-like appearance, texture, and flavor (Fiorentini et al., 2020). However, products containing soy and gluten, which are predominantly used in plant-based products, may be less desirable to consumers because soy and wheat cause allergic reactions, and wheat gluten poses a health risk to people with gluten intolerance (Szpicer et al., 2022). In addition, glyceraldehyde 3-phosphate dehydrogenase, arginine kinase, and tropomyosin cause allergic reactions when insects are encountered or consumed, with tropomyosin acting as the allergen that causes cross-reactivity in edible insects and shellfish, and consumers with shellfish allergies should be wary of consuming edible insects (Aguilar-Toalá et al., 2022). Processing techniques to reduce these allergic reactions include heat treatment such as blanching and frying, extrusion, and enzymatic hydrolysis (Hall et al., 2018), and Mejrhit et al. (2017) reported that heating and enzymatic treatment reduced allergic reactions because when tropomyosin from patients with shellfish allergy was collected, heated, and treated with an enzyme (pepsin), the structure of the antigenic determinant was modified, resulting in the inhibition of the binding reaction between tropomyosin and IgE. However, because of the small number of studies on allergens in edible insects, there may be toxic and allergenic substances that have not been identified, further research is needed to ensure the safe consumption and use of edible insects (Kim et al., 2019c). In addition, as global warming has prompted the production of eco-friendly materials that can reduce carbon, mushroom mycelium is increasing in value as an eco-friendly material that can replace various industrial materials such as analog meat, leather, and plastic, but the production process is complex and production costs are high, so further research on processing technology is needed before these methods can be applied for industrial use (Im et al., 2023).
Conclusion
As the world’s population continues to grow, the demand for animal food increases, but livestock resources are limited compared to the growing number of people. With global warming creating a climate crisis, future food shortages are thought to be inevitable. Therefore, cultured meat, edible insects, and plant-based analog meat have gained attention as future protein resources that can overcome the shortage of meat as a sustainable food. The choice of raw materials and processing technology is important for ensuring that future protein sources can mimic the sensory characteristics (texture, flavor, appearance, etc.) of conventional meat. Industrialization of future protein sources will be possible and sustainable if the raw materials are affordable, in good supply and demand, and can be mass-produced. However, as the sensory characteristics and safety concerns of analog meat do not yet satisfy consumers’ needs, research on processing methods and the safety of raw materials, such as toxic substances and allergens, are needed to improve analog meat. Consumer resistance to new technologies and foods and concerns about potential risks can be mitigated by promoting the environmental and health benefits and sustainability of analog meat to increase consumer acceptance. Particular attention should be paid to developing new forms of future protein sources, such as combining plant-based mushroom mycelium with cultured meat.