Introduction
Physiological dysfunctions in elderly people can be characterized by a reduction of appetite due to changes in the taste threshold and the reduction of digestion and absorption capacity (Zaden et al., 2015). Poor mastication and dysphasia are some of the most common problems and can lead to malnutrition in elderly people. A strong relationship between dysphagia and the negative health outcomes of malnutrition and pneumonia in elderly adults has been reported (Sura et al., 2012). The incidence rate of protein deficiency in the elderly is higher than in younger adults because of the inefficient protein metabolism of elderly individuals. Therefore, older adults require more protein than younger adults (Bauer et al., 2013; Brownie, 2006; Zaden et al., 2015) and a high protein diet improves body function and the quality of life in these individuals (Wolfe et al., 2008). Although a variety of elderly dietary guidelines have been developed, the elderly are still limited with regards to food selection. Moreover, Maitre et al. (2014) reported that eating difficulties have a stronger impact on malnutrition than the choice of food and this malnutrition can have several adverse effects on the body. Beef has a high nutritional value and plays an important role in the human body. Additionally, the nutritive components of beef are easy to digest and the proteins in beef have high biological value (Bruce et al., 1994). However, beef also contains a high quantity of fat (15%), with 30% of the fat comprised of saturated fatty acids. Although beef fat seems to contribute to a softer texture and marbling is an important factor for high quality classification of beef (USDA, http://www.ams.usda.gov/), beef fat is also related to several chronic diseases such as cardiovascular disease, heart disease, diabetes and cancers (Department of Health, 1994; Committee on Medical Aspects of Food Policy, 1984). Consequently, the development of meat tenderization techniques seems to not only produce economically value-added products but also to produce more health oriented food. Mechanical tenderization (Savell et al., 1977), moisture enhancement technology (Robbins et al., 2002), injection of chemical ingredients such as sodium tripolyphosphate, sodium chloride (McGee et al., 2003) and degradation using enzymes (Ashie et al., 2002) are currently applied as tendering techniques. The enzymatic degradation technique involves increasing the tenderness of meat by the degradation of muscle fibers using exogenous enzymes. However, enzymatic degradation results in an undesirable flavor and texture due to broad substrate specificity and the breakdown of major muscle proteins (Ashie et al., 2002; McKeith et al., 1994; Pietrasik and Shand, 2011). Therefore, control of enzyme activity seems necessary to improve the degradation of meat texture and flavour. Nanoparticles are often used to control enzyme activity (Khoshnevisan et al., 2011) by immobilized coating membrane. Liposomes are one of the encapsulation system which are vesicles consisted with phospholipid bilayers membrane (Hsieh et al., 2002; Keller, 2001). The phospholipid bilayer membrane structure of liposomes guarantees to carry hydrophilic and hydrophobic components simultaneously (Hsieh et al., 2002; Keller, 2001) within inner vesicle space and between bilayers membrane. Here, we prepared liposome with nano-scale particles to encapsulate enzyme as hydrophilic material.
This study was performed to develop softening methods for beef to create products that elderly people can easily masticate and swallow. The beef was treated with enzyme encapsulated liposome solution and the chemical properties were compared with non-encapsulated enzyme treated beef.
Materials and Methods
Eyes of round beef (Australia) were obtained from a commercial market (48 h postmortem, pH 5.7-5.9). All the visible fat and connective tissue was trimmed off, and the beef was cut into a rectangular form (3×3×1 cm, 12±1 g) parallel to the direction of the muscle fiber. Protease (protease from Aspergillus oryzae, 500 U/g, Synonym: Flavourzyme® 500L) was purchased from Sigma Aldrich (USA). The soybean lecithin (Lipoid® S75, 68-73% phosphatidyl choline, 7-10% phosphatidyl ethanolamine) used as a coating agent was provided by Lipoid GmbH (Switzerland). All other chemicals used were of analytical grade.
To prepare liposome containing enzyme, 2% (w/v) protease and 2% (w/v) lecithin were dissolved in distilled/deionized water, respectively and then mixed at 1:1 (v:v). Liposomes were produced using a two stage homogenization procedure to obtain small droplets. Pre-liposomes were created with a high speed homogenizer (IKAT25 digital ULTRA-TURRAX®, Germany) at 12,000 rpm for 3 min. Then, the coarse liposomes were treated with an ultrasonificator at 200 W for 3 min. Using the above procedures, liposomes containing 1% protease with fine droplets were produced.
Beef (3×3×1 cm, 12±1 g) was soaked in distilled/deionized water (Control), non-coated protease (protease 1%, NCP) or coated protease (liposome containing protease 1%, CP) at 4℃ for 48 h. Every 12 h, the beef was sampled and the physicochemical properties were analyzed.
The average particle size, size distribution and zeta potential of the enzymes and liposomes were determined by dynamic light scattering using a Zetasizer Nano-ZS90 (Malvern Instruments, UK). All of the samples were properly diluted with distilled water prior to each measurement to avoid multiple light scattering effects. The particle size was indicated by cumulates mean (z-average) diameter and the size distribution was indicated by the polydispersity index (PdI). Since zeta potential is directly related to the electrophoretic mobility of the particles, the analyzer calculated the zeta potential from the measured velocity. All measurements were taken in triplicate.
The pH of the treated (NCP and CP) and control samples were measured with a pH meter (S-220, Mettler-Toledo Co., Switzerland). Samples (5 g) from 3 meat strips were homogenized in 45 mL distilled water for 1 min and the pH of the sample homogenate was determined in triplicate.
The color of the beef surface was determined using a color reader (CR-10, Konica Minolta Sensing Inc., Japan) calibrated with a white standard plate (L*=97.83, a*=0.43 and b*=+1.98). The CIE L*, a* and b* values were determined as indicators of lightness, redness, and yellowness, respectively. The total color difference (ΔE) between the control and the protease treated sample was numerically calculated with the following equation (eq. 1).
The WHC of the beef was determined using the method described by Hong et al. (2012). Approximately 1 g of beef (Ms) was placed in a 15 mL test tube along with gauze as an absorbent and centrifuged at 1,500×g for 10 min at 4℃. The beef pellet was carefully removed from the tube, and the tube was weighed (M1) then weighed again (M2) after drying at 105℃ for 24 h. The WHC of the beef was calculated from the following equation (eq. 2).
The shear force of the treated (NCP and CP) and the control beef was determined in quintuplicate using a texture analyzer (CT3, Brookfield Co., USA) equipped with a v-type plain probe. The conditions used for texture analysis were as follows: compression type, 10 kg force load cell, test speed of 2.5 mm/s, target deformation of 30%, and trigger load of 300 g. The test was repeated at least 10 times. The maximum peak force (kg) was used as an indicator of texture.
Protease activity was determined by Sigma’s Non-specific protease activity assay (Sigma Aldrich, USA) with minor modifications. The beef was immersed in an enzyme- substrate complex solution and protease activity was measured every 12 h. The immersion solutions (0.5 mL) were incubated at 37℃ for 10 min and mixed with 2 mL of TCA reagent to stop the reaction. The samples were filtered using polyethersulfon syringe filters (0.45 μm), and the filtrates (1 mL) were mixed with 5 mL of 0.4 M Na2CO3 and 1 mL of 0.66 N Folin & Ciocalteu phenol reagent. The mixtures were incubated at 37℃ for 30 min and then the absorbance of the samples was measured by a spectro-photometer at a wavelength of 660 nm. For the standard curve, L-tyrosine served as the enzyme-substrate complex solution preceded by the same procedures as above.
The total volatile basic nitrogen (TVB-N) of the beef samples was measured by micro-diffusion analysis (Conway, 1933). Conway reagent was prepared by dissolving 2.5 mL of 0.066% (w/v) methyl red, 2.5 mL of 0.066% (w/v) bromocresol green, 5 g of H3BO3, and 100 mL of ethanol in 350 mL of distilled/deionized water. The samples (10 g) were homogenized at 17,000 rpm for 2 min with 30 mL distilled water. The homogenate was massed up to 100 mL and filtered using Whatman No 2 filter paper. The filtrate (1 mL) was mixed with 1 mL of saturated K2CO3 (60 g K2CO3 in 50 mL distilled/deionized water) as a releasing agent, and 1 mL of conway reagent was used as the trapping reagent. The dish was incubated at 37℃ for 2 h, and titrated with 0.02 N of H2SO4 until the color of the Conway reagent changed to red. As a blank, 5% (w/v) TCA was used, and TVB-N was calculated as follows (eq. 3):
Where VS, VB F, D, and S indicate the titration volumes (mL) of the blank and the sample, titer of 0.2 N H2SO4, dilution factor, and sample amount, respectively.
The thiobarbituric acid-reactive substances (TBARs) in the beef were evaluated using the lipid extraction method described by Pikul et al. (1989) with minor modifications. The samples (3 g) were homogenized in 50 mL 20% trichloroacetic acid-2 M phosphoric acid in the presence of 50 μL of 7.2% (v/v) butylated hydroxyl anisole (BHA) in ethanol. After filtering the homogenized sample, 1 mL of the filtrate was mixed with 2 mL TBA/TCA solution (30 mM TBA in 15% TCA). The mixture was placed in a water bath, kept for 15 min at 90℃, cooled, and centrifuged at 2,000×g for 15 min. After cooling at an ambient temperature, the absorbance of the sample was measured at 530 nm. The TBARS level was determined using a standard curve pre-estimated using tetraethoxypropane and calculated based on the amount of malonaldehyde (MA) equivalents (mg MA/kg sample).
All reported values are the average of three (or more) experiments. Analysis of variance and Duncan’s test were carried out at the 95% confidence level (p<0.05) using the SPSS 20.0 software (SPSS, 2011) to determine significant differences in the results.
Results and Discussion
Particle size, poly dispersity index (PDI) and zeta potential were measured for analysis of the particle properties. Non-coated protease (NCP), Blank liposome (BL) and liposome coated protease (CP) are shown in Fig. 1. The size of the protease was 270±177 nm, and that of the blank liposome (BL) was 169±10 nm. After encapsulation of the protease, the particle size became 365±76 nm, which was significantly larger (p<0.05) then BL. NCP had a wide size distribution range (0.43±0.08) while BL had a relatively uniform size (0.26±0.04), However, CP also showed a wide distribution (0.41±0.07) similar to free protease but there was no significant difference (p<0.05) between NCP and CP. The zeta potential of NCP was -9.8±0.4 mV and that of BL was -72±4.1 mV. The zeta potential of CP was -13±1.9 mV and there was no significant difference (p<0.05) from that of NCP. From our results, the net surface charge of CP and NCP was negative although their surface components are different. It was supposed that the carboxyl groups of NCP are negatively charged the low H+ concentration (pH>isoelectric point) because of carboxyl group such as protein properties.
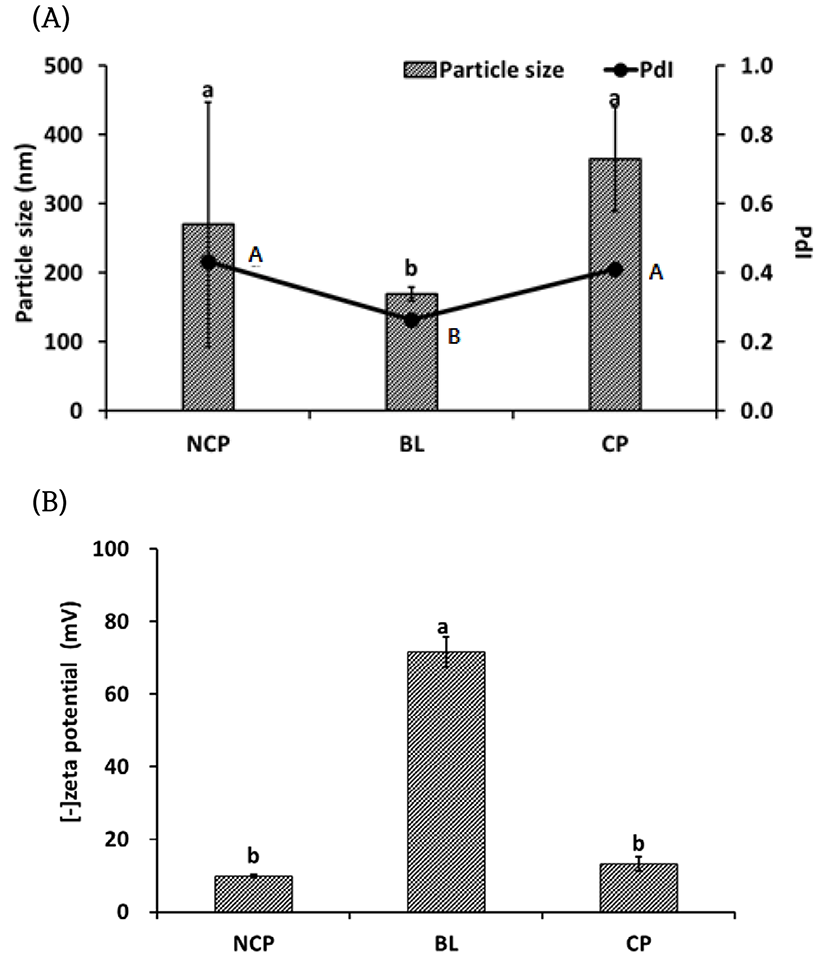
The pH of the beef immersed solution was affected by the type of protease treatment applied as well as the reaction time (Fig. 2). The pH of the control, CP or NCP ranged from 5.72-5.75 at the start and there was no significant difference between the treatments. However, the pH significantly increased with reaction time (p<0.05). In general, both NCP (pH 5.72-5.85) and CP (pH 5.74-5.95) had high pH values although CP had higher pH than NCP through most of the reaction. NCP had the highest value (pH 5.90±0.03) at 36 h and subsequently decreased slightly, while CP continuously increased for 48 h. It seems that the increase in the pH of the beef was a result of the proteolysis. In the present study, endo-type and exo-type mixed proteases were used and the peptide bonds within the protein chain were disrupted. Structural changes were simultaneously manifested and basic residues of muscle protein may have been exposed (Berardo et al., 2015; Ma and Ledward, 2004). Consequently, hydrolysis of the muscle proteins was evidenced by an increase in pH.
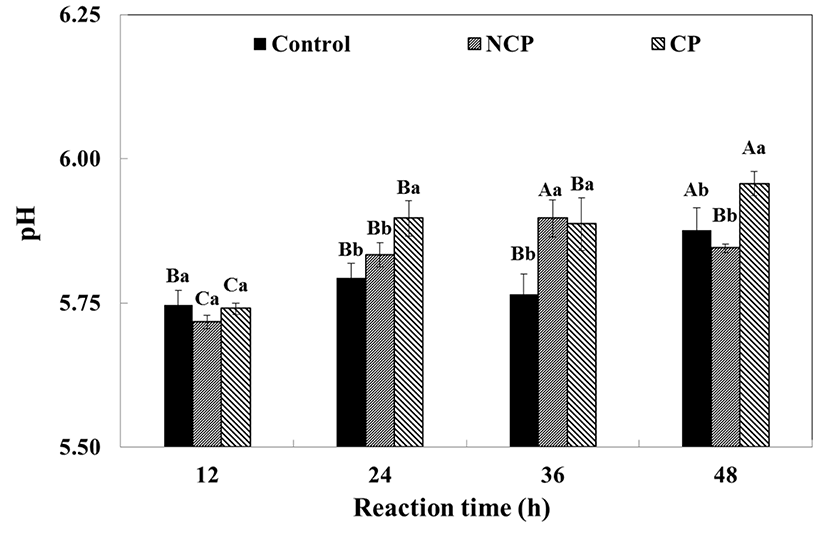
The color properties of beef are shown in Table 1. The beef became darker as the reaction time increased and NCP was relatively darker than CP. The redness of the beef showed no remarkable differences with reaction time or protease treatment. Total color differences in the beef increased slightly within 24 h and increased significantly from 36 h to 48 h. In general, the total color differences appeared to be larger in the control and NCP whereas CP was less affected by the overall reaction time.
1)DW: distilled/deionized water
2)NCP: non-coating protease
3)CP: coating protease
The WHC of the beef decreased with increasing reaction time (Fig. 3). The WHC of the control was 79.39±2.77% at 12 h and decreased to 75.71±0.97% within 48 h while that of CP decreased from 79.16±2.17 to 75.86±1.93%. The control showed no significant difference (p<0.05) from CP. NCP and CP were not significantly different (p<0.05) from the control in the first 12 h, but after 24 h, the WHC of CP and NCP significantly decreased (p<0.05) as the reaction time increased. In general, the WHC of meat was closely related to the muscle pH, and the higher the pH, the higher the WHC of the meat. Meanwhile, the WHC of the beef was decreased by protease treatment, though the partially hydrolyzed beef had higher pH. It is likely that the opposite relationship between pH and WHC was induced by protein degradation (Berardo et al., 2015). Myosin plays a key role in both maintaining the muscle structure and in providing water-binding properties. Upon proteolysis, myosin was partially hydrolyzed, indicating that the muscle had lost its structural integrity, thus losing a larger amount of moisture during centrifugation than the intact muscle (Huff-Lonergan, and Lonergan, 2005). It is possible that the protein hydrolysates had a highly soluble nature. The hydrolyzed peptides were readily resolved in sarcoplasm, and were therefore removed with the exudates during centrifugation. Consequently, the results indicated that the enzymatic hydrolysis resulted in lower WHC.
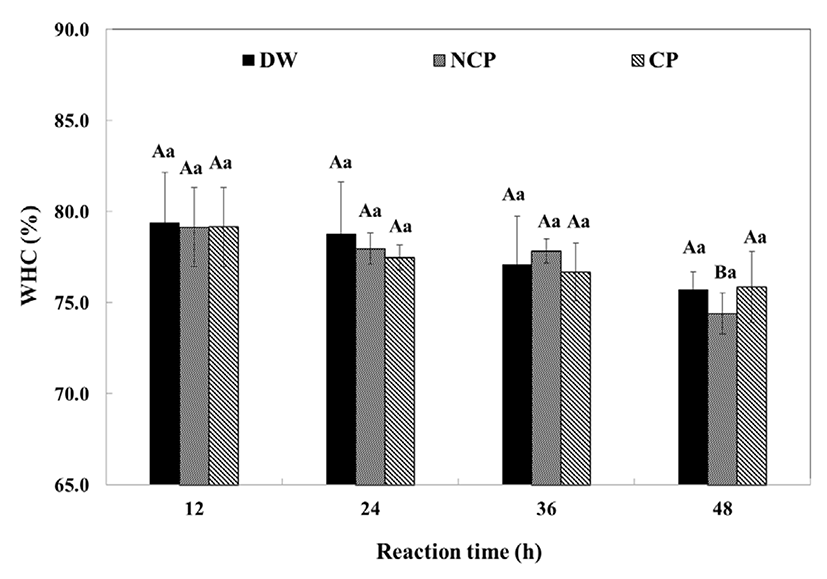
The protease-mediated partial hydrolysis of beef resulted in tenderization of the beef (Fig. 4). The shear force of the control ranged from 2.1-2.4 kg while that of NCP and CP was significantly lower than the control (p<0.05) from 24 h. The control showed no significant differences (p<0.05) during 48 h of storage, whereas the shear force of the protease treated samples significantly decreased from 2752±537 kg to 1595±421 kg (NCP), or from 3042±593 kg to 1716±758 kg (CP) within 24 h. After the first 24 h, the shear force did not change till the end of the reaction. Although NCP had a slightly lower shear force than CP, the difference was not significant. The results indicated that protease treatment was effective for tenderizing beef within 24 h regardless of coating and no differences were observed between CP and NCP.
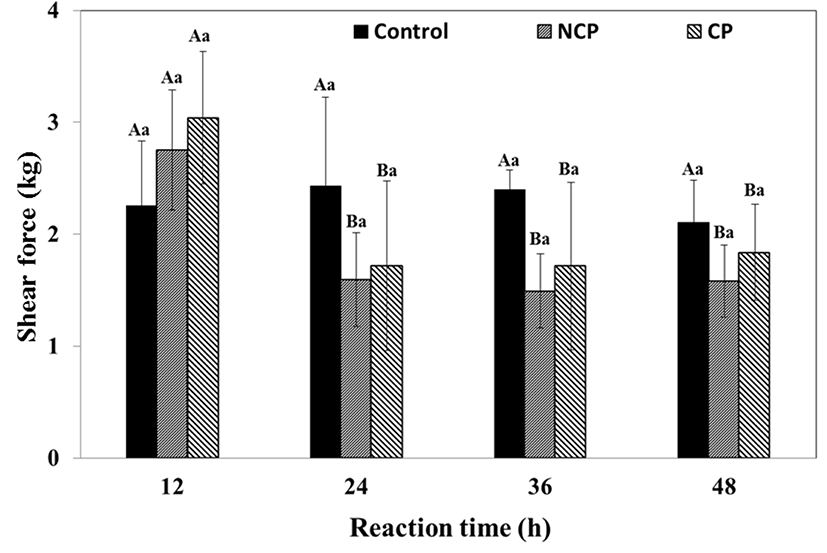
The protease activity of NCP and CP after immersion of the beef was compared (Fig. 5). Tyrosine was not detected in the control initially, but appeared to have increased slightly to 0.04 ppm after 48 h. The NCP or CP contained 0.12 ppm of tyrosine at the beginning, and, over time, these amounts increased to 0.18±0.04 ppm (NCP) and 0.09±0.03 ppm (CP), respectively. The NCP had a significantly high quantity (0.21±0.03 ppm, p<0.05) at 24 h, but the amount of tyrosine at NCP and CP had the same amount after 24 h.
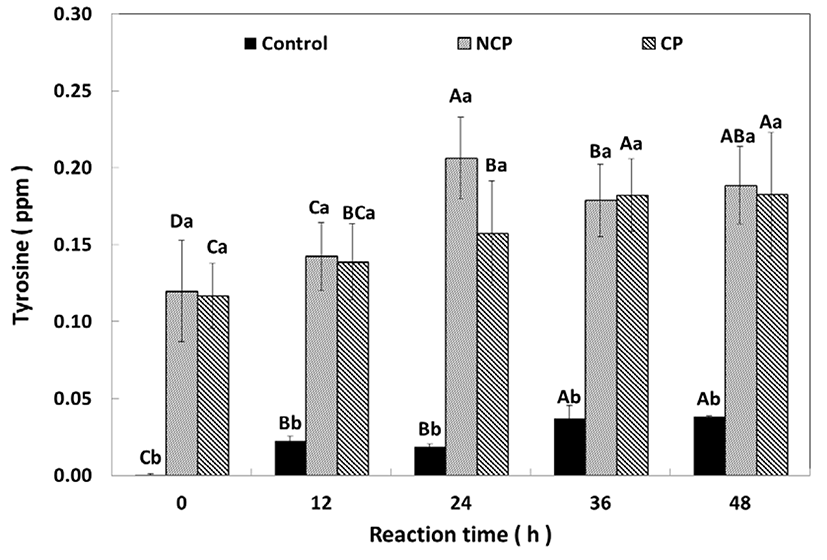
Freshness of meat is usually determined by the amount of total volatile nitrogen (TVB-N) and lipid oxidation (TBARs). The TVB-N and TBARs of the protease immersed meat were measured as a function of time and protease coating. The TVB-N of the immersed beef is shown in Fig. 6. All beef samples showed a significant increase in TVB-N during incubation. The protease treatments had higher TVB-N than the control. During the first 24 h, NCP had a significantly higher value (19.89-25.42%, p<0.05) than the control (13.01-21.04%) and CP (15.38-22.22%). However, the values changed after 36 h of reaction, with CP showing significantly higher values (27.06±3.44%, p<0.05) than the control (20.40±3.83%) and NCP (25.17±3.25%). The TVB-N of the control and NCP increased during the first 24 h and subsequently stabilized, whereas the TVB-N of CP continued to increase for 36 h. The TVB-N of the beef was related to protein hydrolysis. The result is likely because NCP was more efficient at hydrolyzing muscle proteins. The TVB-N of CP probably continued to increase due to the release of protease.
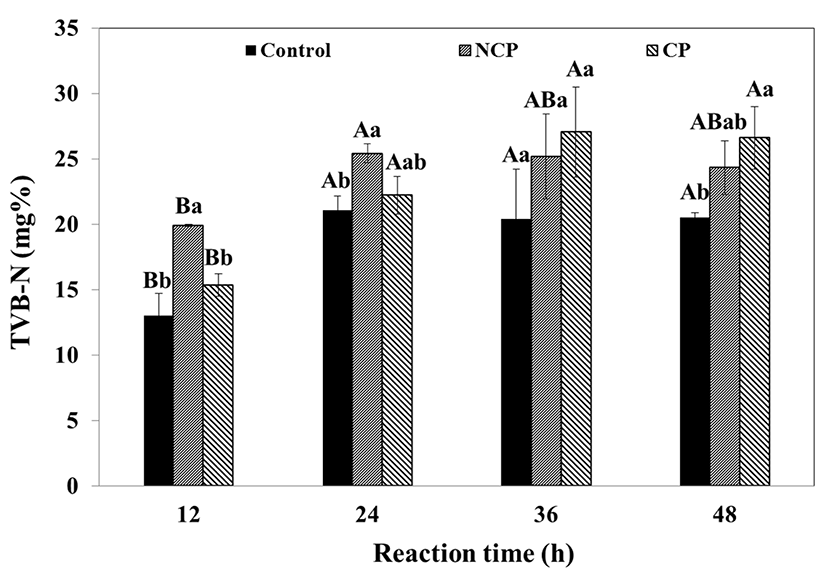
The lipid oxidation of the immersed beef was measured by TBARs analysis and the results are shown in Fig. 7. During the reaction, the TBARs of the protease treatments tended to increase slightly, with significant differences (p<0.05). The TBARs values of the control showed no difference (0.018-0.020 mg MA/kg) over 48 h whereas both protease treatments increased significantly (p<0.05), indicating that protease treatment increases the lipid oxidation of beef. For the NCP treatment, significant increase in TBARs was observed after 24 h, compared to after 48 h for the CP treatment. The TBARs value of CP was significantly higher (0.026±0.002 mg MA/kg, p<0.05) than those of the control and NCP after 12 h. However, it showed no difference until 36 h and then significantly increased at 48 h (0.031±0.004 mg MA/kg, p<0.05). In contrast, the TBARs value of NCP was 0.022±0.002 mg MA/kg at 12 h and significantly increased (p<0.05) at 24 h and also at 48 h. The CP treatment had a higher value, but the rates of CP and NCP increase after 36 h were not different. The higher value of the CP treatment seems to be due to the lipid composition in the liposome coating. Protease immersion seems to affect lipid oxidation, but coating the protease seems to have no effect. Nonetheless, the TBARs range for the control and the treatments was 0.018-0.031 mg MA/kg, which is less than 1 mg MA/kg and has no effect on the taste or flavor of meat. Actually, the relationship between proteolysis and lipid oxidation was unclear. It is possible that the CP treatment used phospholipids as the coating material, which would account for the higher TBARs value. (Cruz-Romero et al., 2008). However, the high TBARs of NCP compared to the control in the present study was not fully understood. It was presumed that the higher TBARS of the protease treatments was a result of the applied enzyme preparation.
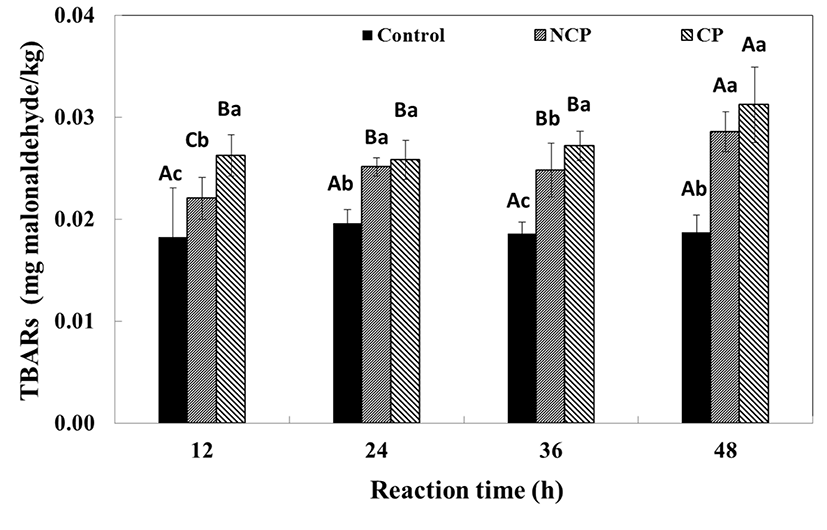
In both TBARs and TVB-N, excessively high reaction time was unfavorable to the quality of the beef. Hence, other strategies such as reaction in cold temperatures or increasing the amount of protease are required to obtain proteolysis activity in the beef, which warrants further investigation.
Conclusion
The effect of liposome coating of protease on beef tenderization was analyzed. Encapsulated protease was compared with non-coated protease according to the size distribution and enzymatic activity. Protease-reacted beef was analyzed to determine the pH, water holding capacity, shear rate, TVB-N and TBARs. Generally, the pH of the beef increased during the reaction and the pH of CP was higher than that of NCP. The total color difference remarkably increased after 36 h. The total difference for CP was relatively lower than that for NCP. WHC significantly decreased within 24 h, but there was no difference between NCP and CP. Protease activity significantly increased over 48 h and no effect was observed from the presence of a protease coating. The TVB-N value of NCP increased over 24 h while that of CP increased after 36 h. The TVB-N value of the protease treatments increased after 36 h and no significant difference between CP and NCP was observed. Consequently, liposome encapsulated protease shows similar properties as NCP. Application of liposome appears to be an interesting option for injecting various functional materials without changing the properties of meat.