Introduction
The oxidation of lipids and myoglobin generates quality deterioration in muscle foods during refrigerated storage, such as off-flavor formation and color changes. Furthermore, the oxidation causes some nutrition loss such as polyunsaturated fatty acids and the generation of free radicals (Morrissey et al., 1998). Antioxidant enzyme activity and iron are certain endogenous factors that affect the oxidation of lipids and protein in meat. Meat antioxidant enzymes, such as catalase, superoxide dismutase (SOD) and glutathione peroxidase (GSH-Px), are naturally developed to inhibit the potential oxidation from endogenous pro-oxidants, which are mostly derived from reactive oxygen species (ROS), such as superoxide anion radical (O2-) and hydrogen peroxide (H2O2), through complex chain mechanisms. These enzymes are considered major antioxidant enzymes found in muscle (Chan et al., 1994).
Iron is the most probable catalyst for the initiation of lipid oxidation by catalyzing the generation of most hydroxyl radicals (•OH) (Buettner and Jurkiewicz, 1996). Approximately two-thirds of the iron in the body is found in hemoglobin, with smaller amounts found in myoglobin, iron-containing enzymes and transferrin. However, not all iron contained in meat promotes lipid oxidation. Free ionic iron released from heme pigments and ferritin is considered one of the major catalysts in lipid oxidation (Min and Ahn, 2005).
Oxidation also varies depending on the animal species, in addition to the effects of the level of antioxidant enzyme activity and free iron content. Min et al. (2008) confirmed this finding by comparing meat from different species, such as beef, chicken and pork. Little is known regarding the effects of animal breeds or lines within similar species on lipid oxidation and the level of its endogenous factors, especially in chicken. Moreover, the correlation between lipid oxidation and several endogenous factors may differ among animal species. Additional studies on species of native animals from specific locations may add new information to meat antioxidant capacity-related study.
Recently, KNC has gained popularity among Korean meat consumers. Their meats are exclusively served and are believed to have special flavor and texture. Ahn and Park (2002) reported that KNC has more desirable taste compounds than broiler. Yeonsan ogye, Hyunin black, Hoengseong yakdak and Hwangbong have been selected as candidates for developing a new chicken line by the Korean governmental institute. Because lipid oxidation is one of the meat quality parameters, the objectives of this study were to observe the antioxidant enzyme activity of four lines of KNC meat and confirm whether lipid oxidation correlates with free iron content and the activities of SOD, catalase and GSH-Px in meat from native animals.
Materials and Methods
HPLC-grade chloroform was purchased from Daejung Chemical and Metals Co. Ltd. (Korea). Distilled water (DW) was purified with a glass still (Mega-PureTM System MP-11A, Corning Inc., USA). All other reagents were purchased from Sigma-Aldrich Co., LLC. (USA).
Four lines of KNC (Yeonsan ogye, Hyunin black, Hoengseong yakdak and Hwangbong) were used as samples in this study and raised at Seoul National University animal farm (Korea) under similar condition. Animals were allowed to free access to feed and water. All the experimental procedures were approved by the Institute of Laboratory Animal Resources, Seoul National University (SNU-070823-5). A total of 16 roosters were randomly sampled and transferred to a chicken slaughter house (Well-cock Hyunjin Co. Ltd, Korea) within group cages for 2 h. After 2 h resting with free access to water, animals were mechanically slaughtered at the age of 12 mon (n=4 birds) using a standard procedure. The breast and thigh meat of each animal were then obtained and separately packed within oxygen-permeable bags (low-density polyethylene, 25 × 30 cm; Clean-wrap, Korea). The samples were kept chilled in an icebox and were transported to the laboratory. The samples were then stored at 4±1℃ for 10 d for oxidation studies. Analyses were performed in triplicate using samples on the initial, middle, and final days.
Lipid oxidation was measured using the 2-thiobarbituric acid reactive substances (TBARS) method by Sinnhuber and Yu (1977) with slight modification. A total of 0.5 g of sample was vortex-mixed with 0.1 g of antioxidant mixture (consisting of propylene glycol, Tween 20, butylated hydroxytoluene, and butylated hydroxyanisole), 3 mL of 1% TBA in 0.3% NaOH, and 17 mL of 2.5% trichloroacetic acid in 36 mM HCl. The sample was heated in a water bath (BW-20G, Biotechnical Services, Inc., US) at 100℃ for 30 min and then immersed in ice water for 15 min. Five milliliters of aqueous sample was then mixed with 3 mL of chloroform. The optical density (OD) of the upper layer was recorded at 532 nm against blank after centrifugation at 2,400 g for 30 min at 4℃ (1248R, Labogene, Denmark). The results were expressed in mg of malondialdehyde (MDA) per kg of meat. Each sample was analyzed in triplicate.
SOD, catalase and GSH-Px were measured in aqueous meat extract dissolved in 50 mM phosphate buffer (pH 7.0 at 25℃) according to a previous study by Renerre et al. (1996). Catalase activity was measured using a method described by Aebi (1984). First, 100 μL of filtered supernatant was mixed with 2.9 mL of 30 mM H2O2 within a crystal cuvette (light path: 1 cm). The decrease in absorbance at 240 nm was recorded every 10 s for 2 min. Catalase activity was expressed as units/g sample. SOD activity was determined according to the pyrogallol autoxidation method described by Marklund and Marklund (1974) with modification. The optical density of a mixture consisting of 50 μL of filtered supernatant, 3.025 mL of 50 mM Tris-cacodylate-DTPA buffer (pH 8.2 at 25℃) and 50 μL of 24.8 mM pyrogallol was recorded at 420 nm every 15 s for 2 min against a blank. One unit of SOD represents the amount of SOD required for the auto oxidation of 50% of the pyrogallol in the meat extract at pH 8.2 and 25℃. The GSH-Px activity measurement was performed according to the enzymatic protocol by Flohé and Günzler (1984) with slight modification. A total of 100 μL of filtered supernatant was mixed with 0.5 mL of phosphate buffer-0.001 M EDTA-0.1 M NaN3, 100 μL of the assay mixture containing 5 units mL−1 glutathione reductase in 50 mM phosphate buffer, 100 μL of 10 mM L-glutathione reduced, 100 μL of 1.5 mM NADPH in NaHCO3, and 100 μL of 1.5 mM H2O2 within a 10-mm precision cell (104-QS, Hellma Analytics, Germany) and incubated for 5 min. GSH-Px activity was measured by recording the decrease in absorbance of the incubated mixture at 340 nm over 2 min. GSH-Px activity was expressed as units g−1 sample. One unit represents the amount of meat extract needed to alter 1 μmol of NADPH with a mM extinction coefficient of 6.3 mM−1 cm−1 at 340 nm.
The amount of free iron in the meat was measured based on a modified version of the ferrozine method (Ahn et al., 1993; Carter, 1971). A 5 g sample was homogenized with 45 mL of citrate phosphate buffer (pH 5.5) at 13,500 rpm for 30 s using a homogenizer (Ultra-Turrax T25 basic, Ika Werke GmbH and Co., Germany). The homogenate was centrifuged at 3,200 g for 30 min at 4℃ (1248R, Labogene, Denmark). Next, 1 mL of the supernatant was mixed with 0.5 mL of freshly prepared 2% ascorbic acid in 0.2 N HCl and incubated at room temperature for 5 min. Then, the mixture was mixed thoroughly with 1.5 mL of 11.3% trichloroacetic acid followed by centrifugation at 10,000 g in an Eppendorf Micro 17R+ centrifuge (Hanil Science Industrial, Korea) for 10 min. Finally, 1 mL of supernatant was mixed with 0.4 mL of 10% ammonium acetate and 0.1 mL of ferroin indicator followed by 5 min incubation. The absorbance was read at 562 nm against a blank. The free iron value was expressed as μg/g meat.
The one-way analysis of variance (ANOVA) was used for the effect of different meat parts. Thigh meat was observed to have higher TBARS value, antioxidant enzyme activity and iron content than breast meat. A 4 × 3 factorial design with three replicates each meat part was employed with chicken lines and storage times as the main effects, using two-way ANOVA. No significant interactions were found. The statistical significance of the differences between the means from different lines and storage times was determined by Duncan’s multiple range test. P values of less than 0.05 were regarded as significant. The Pearson’s correlation coefficients and linear regression between TBARS values and other parameters were measured. Data used for regression analysis were pooled according to chicken lines, meat parts and storage times (N=24). Analyses were performed using R-version 3.2.0 with the “Agricolae” library (The R-foundation for Statistical Computing, Austria).
Results
The degree of lipid oxidation is represented by the increasing amount of malondialdehyde content during storage. Refrigerated storage significantly increased the production of malondialdehyde for both the breast and thigh meat of each KNC (p<0.001) line. The highest malondialdehyde contents were observed on the final day of storage, ranging from 0.40 to 0.45 mg/kg meat for breast meat and 0.53 to 0.79 mg/kg meat for thigh meat (Table 1). Among KNC, the highest TBARS values were found in the thigh meat of Yeonsan ogye and Hoengseong yakdak (p<0.05). However, no significant chicken lines effects were found in breast meat.
Means in the same column (x, y, z) and row (a, b) followed by different letters are significantly different (p<0.05).
SEM, standard error for the means.
The decline of antioxidant enzyme activity in KNC meat as affected by refrigerated storage is shown in Table 2. The SOD activity on the initial day of storage ranged from 124 to 148 units/g meat for breast and 144 to 172 units/g meat for thigh. This value then decreased on the final day of storage, ranging from 61.71 to 73.14 units/g meat and 68.57 to 98.29 units/g meat for breast and thigh, respectively. At day 0, the activity of catalase ranged from 69.14 to 73.77 units/g meat for breast and 118.07 to 133.07 units/g meat for thigh. At day 10, this value decreased to 36.29-48.55 units/g meat and 50.97-81.97 units/g meat for breast and thigh, respectively. The activity of GSH-Px decreased as well, ranging from 0.27 to 0.37 units/g meat for breast and from 0.54 to 0.62 units/g meat for thigh at day 0, dropping to 0.11-0.13 units/g meat and 0.12-0.14 units/g meat at day 10 for breast and thigh, respectively. Chicken lines affected the activity of SOD and GSH-Px in thigh meat (p<0.05), whereas no effects were observed on antioxidant enzyme activity in breast meat. The highest activity of SOD was found in thigh meat of Hwangbong and Hyunin black had the highest GSH-Px activity in its thigh meat.
Means in the same column (x, y, z) and row (a, b) followed by different letters are significantly different (p<0.05).
SEM, standard error for the means.
The amount of iron released during refrigerated storage from KNC meat is shown in Table 3. At day 0, the amount of released iron ranged from 0.30 to 0.39 μg/g meat for breast and from 0.76 to 1.15 μg/g meat for thigh. After 10 d of storage, this value elevated to 0.41-0.49 μg/g meat and 1.21-1.25 μg/g meat for breast and thigh, respectively. No significant differences were found among chicken lines.
Means in the same column (x, y) followed by different letters are significantly different (p<0.05).
SEM, standard error for the means.
The correlation between TBARS values and the activity of antioxidant enzyme is shown in Fig. 1A, 1B and 1C for SOD, catalase and GSH-Px, respectively. The SOD and GSH-Px activities were negatively correlated with TBARS values in the breast and thigh meat of KNC during refrigerated storage, whereas catalase activity was not significantly associated with lipid oxidation. The correlation coefficients for SOD and GSH-Px were −0.655 and −0.493, respectively.
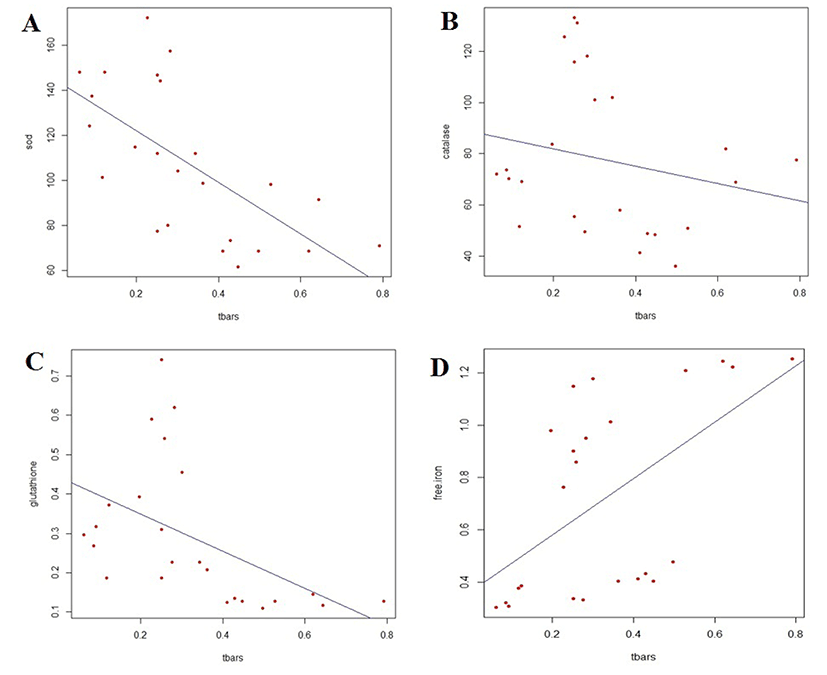
The association of TBARS values with the amount of released-iron of KNC meat during refrigerated storage is shown in Fig. 1D. A significant correlation was found between free iron content and lipid oxidation (p<0.01). The free iron content was positively correlated with the increasing amount of malondialdehyde content in KNC meat, with a correlation coefficient of 0.552.
Discussion
Muscle fibers can be categorized into two different metabolic types, oxidative (red) or glycolytic (white), based on their chemical composition and enzyme activities (Warris, 2000). In this study, the oxidative and glycolytic muscles were represented by thigh and breast meat, respectively. Because oxidative muscle fibers are found relatively higher in thigh meat than breast meat. Our results are in line with Renerre et al. (1996), in which, oxidative muscle was found to exhibit higher antioxidant enzyme activities than glycolytic muscle. Although previous results support increased antioxidant enzyme expression in the highly oxidative environments of living tissues, it does not substantiate the role for these enzymes in postmortem muscle tissues or so-called meat (Bekhit et al., 2013). Moreover, Lee et al. (1996) reported a higher antioxidant enzyme activity in the thigh meat of turkey than in its breast meat.
Catalase and GSH-Px are considered the major peroxide-removing enzymes located in cytosol, whereas SOD plays a role in the protection against damage resulting from superoxide anion radicals (Chan et al., 1994). SOD and catalase are coupled enzymes, in which SOD scavenges superoxide anions by forming hydrogen peroxide, and catalase safely decomposes hydrogen peroxide to water and oxygen; GSH-Px can decompose both hydrogen peroxide and lipoperoxides formed during lipid oxidation (Gatellier et al., 2004; Terevinto et al., 2010). Antioxidant enzyme activity differs in the meat from different animal species (Pradhan et al., 2000). Hernandez et al. (2004) and Chen et al. (2012) noted that antioxidant enzyme activity also varies in pork from different lines, which agrees with our results, particularly that of thigh meat. However, the antioxidant enzyme activity of breast meat in present study contrast previous results because breast meat is composed of relatively low oxidative muscle fibers compared to thigh meat.
As expected, the decline of the activity of antioxidant enzyme was affected by the duration of storage. Storage temperature and the presence of oxygen might also be responsible for this decline. Our results contrast with Kang et al. (2014), who reported that the activity of GSH-Px in Hanwoo beef increased during refrigerated storage after 7 d of freezing within vacuum-packaging. However, no freezing condition was applied in this study and the samples were stored aerobically. Because these enzymes are protein complexes, they can also be degraded through oxidation processes into carbonyls. Soyer et al. (2010) used the increasing amount of carbonyls as a biomarker for protein oxidation in chicken meat. Moreover, the decline of antioxidant enzyme activity may be resulted by the utilization of the enzyme to protect cells from post slaughter autooxidation. Thus, their antioxidant ability declined during storage under aerobic conditions. Only the catalase activity was not significantly associated with lipid oxidation as strong as other antioxidant enzymes observed in this study. Previous study by Chen et al. (2012) mentioned that the activity of catalase has lower association with lipid oxidation in pork loin than the activity of SOD and GSH-Px. Therefore, we suggest that SOD and GSH-Px significantly influence the inhibition of lipid oxidation in KNC meat.
Lombardi-boccia et al. (2002) reported that the oxidative meat (thigh, drumstick and wing) contained more iron than the breast meat of the broiler and turkey because oxidative muscles have higher myoglobin content. Furthermore, Min et al. (2008) reported that the heme and non-heme iron contents markedly differed among the meat types; the iron content was higher in chicken legs and wings than that in breast meat. In this study, the amount of free iron content was consistently higher in thigh meat from different lines of KNC.
In contrast to antioxidant enzyme activity, the free iron content increased when lipid oxidation occurred during storage, resulting in a significant correlation. However, our results did not corroborate with those of Min et al. (2008), who reconfirmed that ferrylmyoglobin and hematin are the major catalysts particularly during oxidation processes rather than free ionic iron, and found a declining trend of free ionic iron during chilled storage in broiler breast and thigh. The decrease may be attributed to the differences in slaughter age and pre-treatment of the samples.