Introduction
Lactoferrin (Lf) can find in the secondary granules of neutrophils and blood, as well as diverse mammalian secretions such as saliva, rhinorrhea, tears, and milk (Shimazaki, 2000). Antimicrobial activity of Lf was first reported by Reiter and Oram (1967). The antimicrobial effect of Lf is related to its iron-chelating ability and also to a direct effect on the bacterial cell surface (Farnaud and Evans, 2003). The antimicrobial activity of Lf enzymatic hydrolysates generated by pepsin digestion is stronger than that of the undigested Lf (Bellamy et al., 1992a, 1992b). The antimicrobial domains of bovine and human Lf are lactoferricin B (17-41) and lactoferricin H (1-47), respectively. Lactoferricin has broad-spectrum activity against various microorganisms, including gram-negative and -positive bacteria, fungi, and viruses, and has antitumor activity (López-Expósito and Recio, 2006). Antimicrobial activity of the purified lactoferricin B is thought to be due to destabilizing the cytoplasmic membrane and damaging the outer bacterial cell (Sallmann et al., 1999; Vorland et al., 1999; Yamauchi, 1993).
Pseudomonas is a gram-negative rod bacterial genus, where lives in a wide range of soils and waters (Yong et al., 2015). Plant diseases represent a major economic problem because of the devastating damage they cause to cultivated and stored crops (Vidaver, 2002). P. syringae is a plant pathogen that has gained economic importance worldwide owing to the damage caused by necroses and chlorosis on leaves, fruits, and stems (Alfano and Collmer, 1996; Hirano and Upper, 2000). Also, toxins of P. syringae can be produced on leaves, fruits, stems, and in culture media (Bender et al., 1999). Therefore, the agricultural industry employs a wide variety of synthetic antibacterial agents. Antimicrobial peptides are currently receiving increased attention as defense compounds because of their dual action against phytopathogenic bacteria. In the present study, we investigated the antimicrobial effect of bovine lactoferrin hydrolysates on P. fluorescens and P. syringae.
Materials and Methods
Pseudomonas syringae DSM5175 and Pseudomonas fluorescens DSM 50090 were obtained as freeze-dried samples from the DSMZ-German Collection of Microorganisms and Cell Cultures (Germany). Native bovine lactoferrin was purchased from Morinaga Milk Industry Co. Ltd. (Japan). The chemiluminescence assay reagents kit (BactoLumix) was purchased from ATTO Company (Japan). Porcine pepsin was obtained from Sigma (USA). P. syringae and P. fluorescens were cultured in Luria-Bertani (LB) medium at 25℃ and 30℃, respectively.
Bovine lactoferrin hydrolysates (bLFH) were obtained using the method described by Bellamy et al. (1992a). Briefly, bLf was dissolved in a sterile milliQ to a concentration of 5% and the pH was adjusted to 3.0 using 1 N HCl. Porcine pepsin A (Sigma, USA) was added to achieve a final concentration of 3% (w/w of substrate). This mixture was incubated at 37℃ for 4 h. Pepsin was then inactivated by heating at 80℃ for 15 min in a water bath. The hydrolyzed sample solution was brought to pH 7.0 by continuous addition of 1N NaOH. Insoluble solids were removed by centrifugation at 16,000 g for 15 min. The supernatant fluid was freeze-dried and stored at 4℃ until use.
The molecular weight of hydrolysate samples was determined by tris-tricine/SDS-PAGE (Schägger and von Jagow, 1987). A molecular weight marker with sizes ranging from 2.5 to 45 kDa (Amersham Bioscience Europe GmbH, Germany) was used for comparison. The gel was stained with Coomassie Brilliant Blue R-250 (Nacalai Tesque, Inc., Japan).
The antimicrobial ability of bLFH against Pseudomonas spp. was measured using a luminol chemiluminescent assay (LC assay) and a paper disc plate assay. P. fluorescens and P. syringae were preincubated in LB medium at 30 and 25℃, respectively. The solutions of bLFH samples were sterilized by passing through a 0.2 μm filter (Germany).
In each test tube, prepared bLFH samples were mixed with LB medium to a final volume of 3 mL and then inoculated with 1% of the precultured test strain. bLFH were added at concentrations of 15, 7.5, 3.75, 1.88, 0.94, 0.47, 0.23, 0.12, 0.06, 0.03, 0.015, or 0.007 mg/mL.
A series of 1- or 2-fold bacterial cell suspension dilutions were prepared in 0.1% peptone broth. The diluted cell suspension (50 μL) was placed in each test tube (10 × 75 mm), and 50 μL of menadione solution was added. After incubation at 37℃ for 10 min, luminol chemiluminescent intensity (LCI) was determined 2 s after injection of 100 μL of the chemiluminescence reagent (ATTO) using Luminescencer AB-2200 (ATTO). All results presented in this paper represent the means of three repetitions for each assay. Furthermore, Disc assays were performed according to the standard method (Bruhn et al., 1985) with some modifications. Ten milliliters of agar containing LB medium was mixed with 1% of the precultured target bacteria in a petri dish (diameter 10 cm), and then overlaid with agar containing LB medium. Sterile filter paper discs were placed on the agar surface. bLFH were prepared in different concentrations of 15, 7.5, 3.75, 1.88, 0.94, 0.47, 0.23, 0.12, 0.06, 0.03, 0.015, or 0.007 mg/mL, and then 30 μL of each solution was spotted onto the filter disc. Then, the petri dishes containing agar and paper discs were maintained in an upright position for drying at room temperature before being inverted and incubated at the appropriate temperature for 24 h. Antimicrobial activity was evaluated by measuring the zones of growth inhibition.
P. syringae was pre-incubated in LB broth at 25℃. A selected concentration (0.94 mg/mL) of bLFH was added at various bacterial growth stages and incubated at 25℃. Samples were taken at different time intervals to measure the absorbance at 620 nm using an MPR-A4i microplate reader (TOSOH, Japan).
bLFH samples were analyzed by reverse-phase chromatography on an Asahipak C4P-50 column (150 × 4.6 mm i.d.; Showa Denko, Japan) connected to a LC-Net II/ADC HPLC system (Jasco, Co., Japan). The solvents consisted of 0.1% (v/v) trifluoroacetic acid (TFA) in water (A) and 0.1% (v/v) TFA in acetonitrile (B). The sample was injected 10 mg/mL. The flow rate was 1 mL/min, and absorbance was measured at 220 and 280 nm to detect the eluted compounds. Elution of samples obtained from the peaks was adjusted to pH 7.0, and their antimicrobial activity against P. syringae was measured using a 96-well plate method (Nunc, Co., Denmark). The absorbance at 620 nm was taken as a control. All assays were performed in parallel at least three times. The amino acid sequence of the purified antimicrobial peptide was determined by the Edman degradation method.
Results and Discussion
The antimicrobial activity of bLFH against P. fluorescens and P. syringae was assessed by LC assay and paper disc plate assay (Figs. 1 and 2). An antimicrobial effect against P. fluorescens was not observed either by the paper disc plate assay (A) or by the LC assay (B) at any concentration (Fig. 1). Therefore, bLFH did not exhibit antimicrobial activity against P. fluorescens. However, growth of P. syringae was significantly inhibited in the presence of bLFH (Fig. 2). The addition of bLFH into liquid (B) and solid medium (A) resulted in growth inhibition of P. syringae in a dose-dependent manner (Fig. 2). As shown in Fig. 2B, the minimum inhibitory concentration in the LCI assay was over 0.06 mg/mL. Antimicrobial halo size in the paper disc plate assay was observed at higher bLFH concentrations; the minimum inhibitory concentration in this assay was 0.12 mg/mL (Fig. 2A).
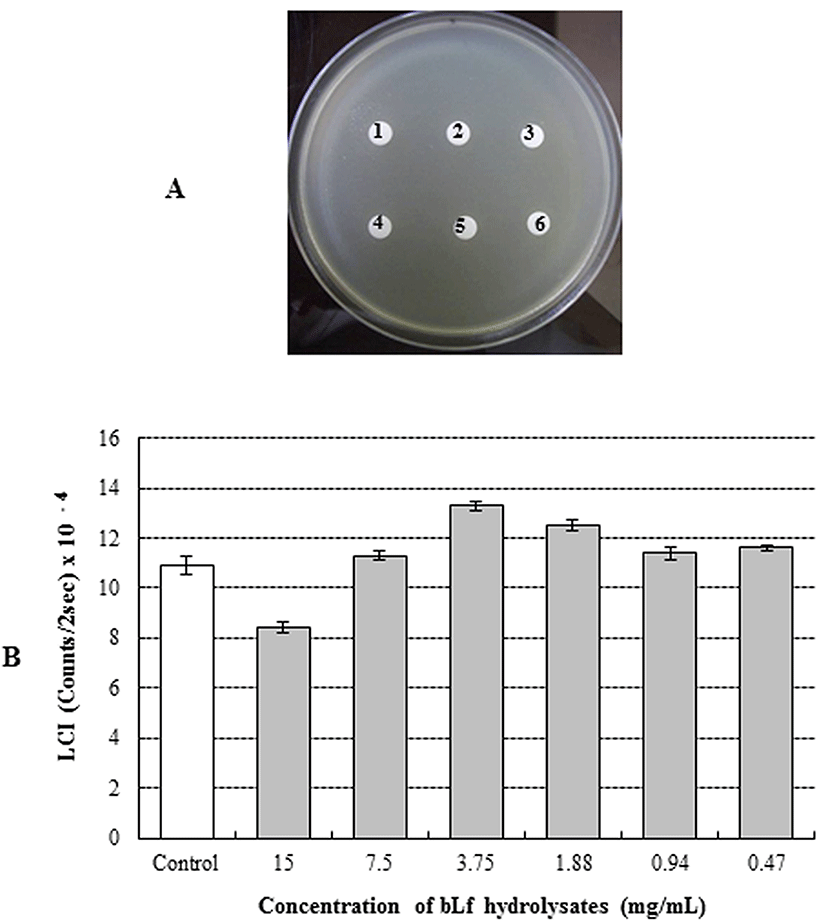
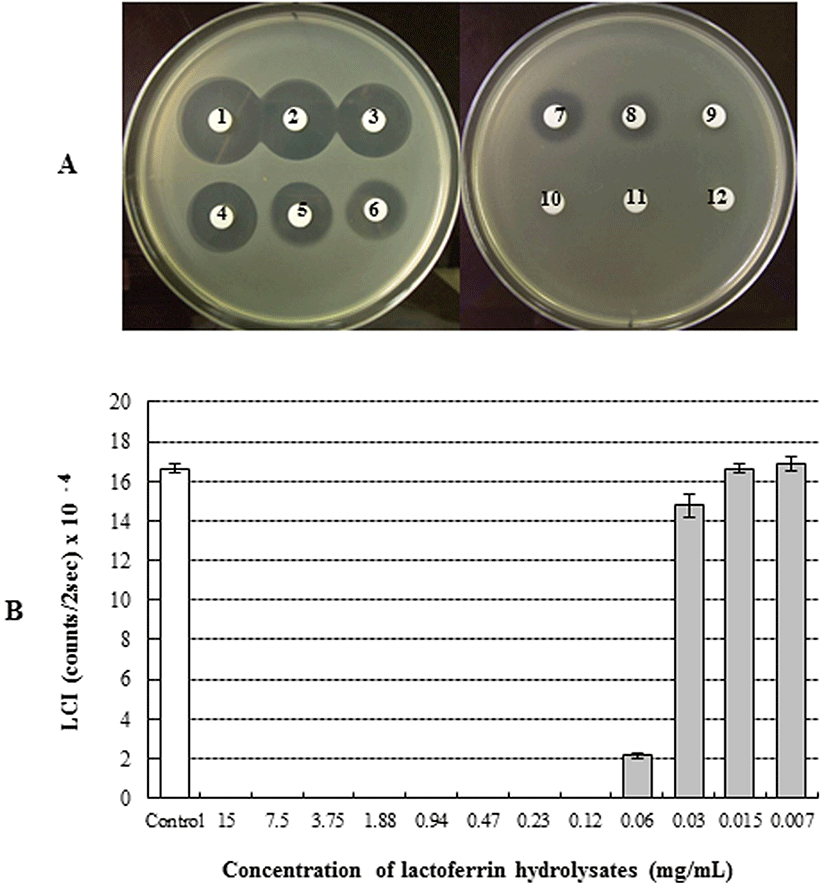
In order to investigate activity of selected bLFH at bacterial growth stages, bLFH were added to the liquid culture of P. syringae. The addition of bLFH to P. syringae culture in the logarithmic phase showed a decrease in optical density at 620 nm at the expected time interval (Fig. 3). Therefore, bLFH exhibited an antimicrobial activity against P. syringae.
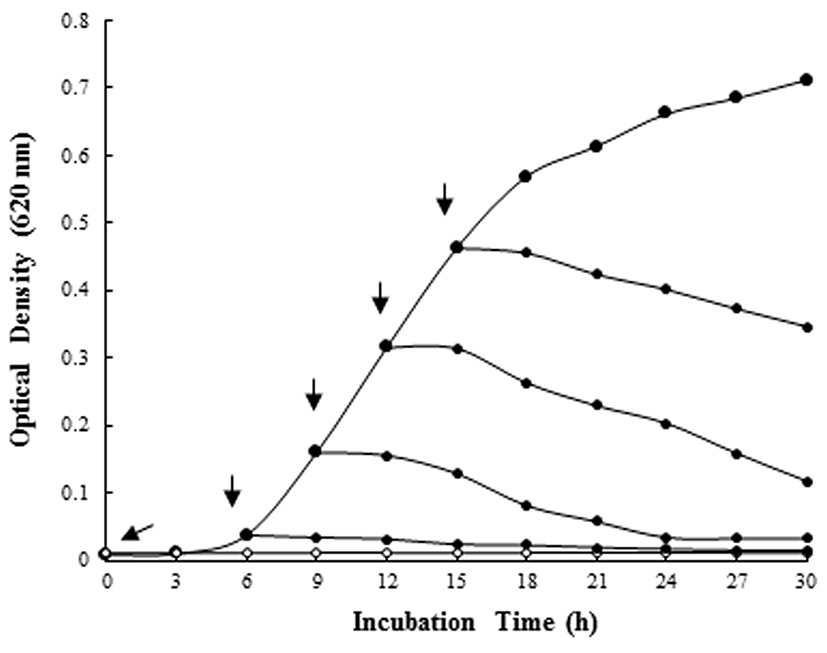
We also determined the antimicrobial peptide of bLFH against P. syringae. Tricine-SDS PAGE analysis of bLFH was performed (Fig. 4A). bLf has been digested with a molecular weight < 10,000 Da by pepsin (Fig. 4A). bLFH was analyzed by reverse-phase chromatography on an Asahipak C4P-50 column connected to a LC-Net II/ADC HPLC system. The elution profile of bLFH on an Asahipak C4P-50 column is shown in Fig. 4B. Collected individual peaks were adjusted to pH 7.0 and their antimicrobial activity against P. syringae was measured using 96 well plate methods. To investigate the antimicrobial activity against P. syringae, 10% (v/v) of elution peaks were added to the culture media. As shown in Fig. 4C, the highest inhibitory activity was shown by fractions 1, 2, 10, 11, and 12, whereas the other fractions did not inhibit cell growth. N-terminal amino acid sequences of antimicrobial bLf peptides were analyzed by a protein sequencer. The N-terminal 10 residues of the peptides in the bLf were Leu-Arg-Ile-Pro-Ser-Lys-Val-Asp-Ser-Ala for fractions 1 and 2, and Phe-Lys-Cys-Arg-Arg-Trp-Gln-Trp-Arg-Met for fractions 10, 11, and 12. The position of each peptide in bLf is shown in Fig. 5. Fractions 10, 11, and 12 were suggested to have an antibacterial domain, termed lactoferricin. On the other hand, fractions 1 and 2 also were in the N-lobe fragment. Thus, we have identified a new antimicrobial peptide within the N-terminal domain against P. syringae. In order to ensure the activity of this new peptide, the peptide was synthesized and its activity was investigated. We experimentally confirmed that the synthesized peptide showed antimicrobial activity against P. syringae (data not shown).
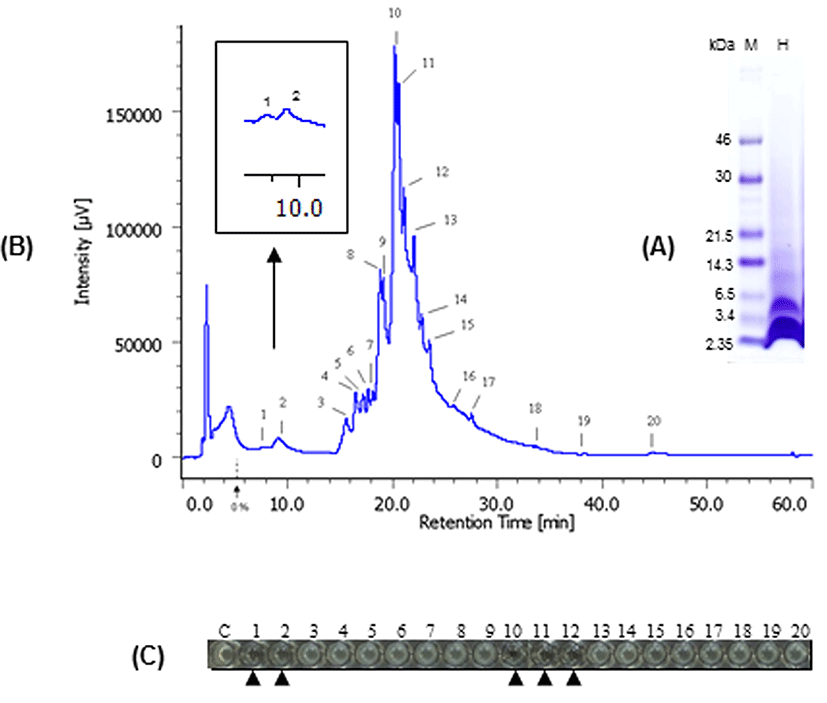
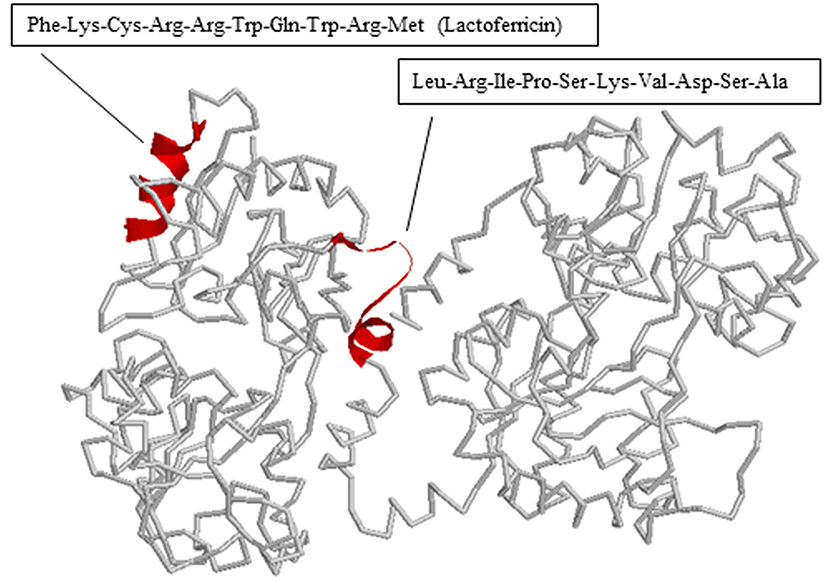
The antimicrobial effects of bLf against P. syringae can be explained by several mechanisms. Antimicrobial peptides from the N-terminal region of bLf have been reported. Lactoferricin B is a peptide that was first isolated by Tomita et al. (1991) from the gastric pepsin cleavage of bLf. Murdock and Matthews (2002) reported that bLFH obtained by pepsin hydrolysis exhibited antimicrobial activity against Salmonella stanley, Escherichia coli, Listeria monocytogenes, and Staphylococcus aureus under low pH and refrigeration conditions. The antimicrobial mechanism involves binding to techoic acid (gram-positive bacteria) and lipopolysaccharide (LPS: gram-negative bacteria) (Vorland, 1999). However, several researchers have observed bacteria resistant to peptic bLFH. Bellamy et al. (1992a, 1992b) demonstrated that the strains resistant to Lactoferricin B are Pseudomonas fluorescens, Enterococcus faecalis, and Bifidobacterium spp. Kawai et al. (2007) also reported that S. aureus, Enterococcus faecalis, and E. coli are resistant to bLFH. In this study, bLFH exhibited resistance to P. fluorescens (Fig. 1). However, growth of P. syringae was inhibited by bLFH (Fig. 2). Therefore, our result for P. fluorescens is in agreement with previous studies reporting bacteria resistant to lactoferricin B.
Lactoferrampin (LFampin) was identified in the N1-domain (residues 268-284) of bLf by Van der Kraan et al. (2004). LFampin showed some antimicrobial activity, such as lactoferricin. Antimicrobial activity of LFampin was higher than bLf (Van der Kraan et al., 2004). The antimicrobial effect of Lf is related to have an iron-chelating ability (Lonnerdal and Iyer, 1995; Weinberg, 2001). Arnold et al. (1977) reported that apo-Lf is inhibited the growth of Vibrio cholera and Streptococcus mutans. In addition, the low concentration of iron in medium may have the effect of inhibiting the growth of bacteria (Ellison et al., 1988; Pahud and Hilpert, 1976). Furthermore, the bLf binding protein could play a biological role in growth stimulation or inhibition, because such binding proteins have been found on the cell surface of variety of bacteria, i.e., Bordetella pertussis (Redhead et al., 1987), Mycobacterium pneumoniae (Tryon and Baseman, 1987), Helicobacter pylori (Dhaenens et al., 1997), Staphylococcus spp. (Naidu et al., 1990), Streptococcus uberis (Moshynskyy et al., 2003), Streptococcus pneumoniae (Hammerschmidt et al., 1999), and Moraxella spp. (Yu and Schryvers, 2002). Previously, we first detected bLf binding proteins in the membrane fraction of bifidobacteria (Kim et al., 2004). Interestingly, B. longum ATCC 15707 did not detected bLf binding protein. Thus, the addition of bLf did not promote the growth of B. longum ATCC 15707. bLFH also shows bactericidal activity against P. syringae but not P. fluorescens (Fig. 1 and 2). Therefore, further study is needed the interaction between Pseudomonas strains and lactoferrin.
Conclusions
The antimicrobial activity of bovine lactoferrin hydrolysates was measured in vitro against Pseudomonas syringae and P. fluorescens. Antimicrobial effect against P. fluorescens was not observed by either assay, suggesting that bLFH did not exhibit antimicrobial activity against P. fluorescens. However, a significant inhibition of P. syringae growth was observed in the presence of bLFH. bLFH peptide with antimicrobial activity toward P. syringae was isolated and identified. We identified a new antimicrobial peptide against P. syringae, present within the N-terminus and possessing the amino acid sequence of Leu-Arg-Ile-Pro-Ser-Lys-Val-Asp-Ser-Ala.