Introduction
Raw milk contains more amino and fatty acids, volatile compounds, and a diverse microbial population than does pasteurized milk; these components are lost on pasteurization (Grappin and Beuvier, 1997). Hence, raw milk cheeses have a stronger taste and a richer flavor (Fuka et al., 2013). However, raw milk may also contain undesirable microbes, thereby increasing the probability of pathogens surviving in the “ready-to-eat” cheese matrix (Pelaez and Requena, 2005) and are hence considered microbiologically unsafe (Yoon et al., 2016). Moreover, global outbreaks of food-borne disease are attributed to consumption of raw milk cheese. According to a survey in the U.S., consumption of raw milk or its products resulted in 93 illness outbreaks, 1,837 illnesses, 195 hospitalizations, and 2 deaths, from 1998 to 2009. These outbreaks were primarily caused by intake raw milk products contaminated with Escherichia coli O157, Campylobacter and Salmonella species (Committee on Infectious Diseases; Committee on Nutrition; American Academy of Pediatrics, 2014). While limited microbiota in pasteurized milk cheese enabled the manufacture of cheese varieties with unique flavor and texture, the microbiological safety of raw milk cheese is disputable.
Lactic acid bacteria (LAB), a representative subgroup of microbial species in raw milk cheese that inhibit the growth of food-borne pathogens by producing antimicrobial substances such as organic acids, H2O2, and bacteriocin. Previous studies reported that Lactobacillus plantarum appeared to inhibit the growth of Salmonella Typhimurium due to its acid production during ripening of raw milk Montasio cheese (Stecchini et al., 1991). Lactococcus garvieae strains were found to produce bacteriocins against food-related pathogens (Florez et al., 2012; Fortina et al., 2007). Non-starter lactic acid bacteria (NSLAB) such as mesophilic lactobacilli are more diverse in raw than in pasteurized milk Cheddar cheese (McSweeney et al., 1993) and comprise strains that produce the characteristic flavors of traditional cheese during maturation (Shakeel-Ur-Rehman et al., 2000; Wouters et al., 2002) hence being at least partly responsible for differences in flavor between pasteurized and raw milk cheese (Wouters et al., 2002).
Until present, culture-based microbial methodology was employed to explore a dynamics of microbial community in cheese. However, this method has critical limitation on detecting no-cultivable or no-abundant microorganisms during cheese ripening. For overcoming this disadvantages, recent reported advances, such as next-generation sequencing (NGS) technology, are useful because of the enhanced sequencing depth that can be less labor intensive and more informative in characterizing the microbial diversity in given habitats (Fuka et al., 2013). Particularly, the technology has also been used to study the bacterial diversity of cheeses as well, including various artisanal cheeses (Lusk et al., 2012).
The U.S Food and Drug Administration (FDA) requires to sample and test raw milk cheese made aged 60 d. After 60 d, the acids, salts and LAB derived from raw milk cheese naturally prevent Listeria, E. coli and Salmonella from growing. Evidence indicates that raw milk cheese aged for 60 d may not eliminate or adequately reduce E. coli O157:H7 and Salmonella in raw milk cheese (Reitsma and Henning, 1996; Schlesser et al., 2006). In Korea, the distribution of natural cheese made from non-pasteurized milk has been limited to products that have been individually certified through safety tests. From 2016, Korea revised its processing standards to allow the manufacturing and import of raw milk cheese to ripening at least 60 d and 2°C. Currently, there were about 100 artisanal cheese factories in Korea, but most raw milk and its products were rare, as conventional dairy products were pasteurized, and consumers tend to be worried about the raw dairy products. Hence, this study aimed to analyze dynamics in bacterial diversity during the ripening of cheeses manufactured from raw and pasteurized milk. In addition, we evaluate variations in physico-chemical and microbiological parameters in raw and pasteurized milk Gouda cheese during ripening and provide evidence on the potential use of raw milk for the manufacture and ripening of Gouda cheese.
Materials and Methods
Two types of Gouda cheese, pasteurized (P-GC) and raw milk cheeses (R-GC) were manufactured using raw milk [obtained from five Holstein (cows averaging 5.5 parties and 32.7 kg/d of milk yield) in the National Institute of Animal Science, Wanju, Jeollabuk-do, Korea] and pasteurized milk. For each cheese type independent batches of milk were used resulting in 4 batches of milk (2 times×2 cheese types). From each cheese type per time 3 replicates based on the same batch of milk (100 kg) were analyzed at each time point of ripening (6, 12, 18, and 24 wk) in triplicate. To manufacture pasteurized milk cheese, 100 kg of raw milk was pasteurized at 65°C for 30 min and cooled to 32°C. The pasteurized and raw milk were inoculated with 0.0015% (w/v) of starter culture CHN-11 (Chr. Hansen, Horsholm, Denmark), which comprised four strains of Lc. lactis subsp. cremoris, Lc. lactis subsp. lactis, and Lc. lactis subsp. diacetylactis, and Leuconostoc. Further, 0.019% (v/v) rennet (Naturen, 90% Chymosin, 290 IMCU/mL, Chr. Hansen, New Zealand) was added to induce the formation of curds. The milk was allowed to coagulate for 45 min, and the coagulum obtained was then cut vertically and horizontally into 7 mm cube-shaped particles. After the curds were simultaneously stirred for 20 min, the whey was removed (40% of the volume of the milk) and water (30% of the volume of the milk) pre-heated to 50°C was added, and the mixture was stirred for 20 min to maintain the final temperature at 35°C. Thereafter, 30% (the volume of the milk) of whey was removed and 20% (the volume of the milk) of 50°C water was added and the curds were stirred for 50 min, and the final temperature was 38°C. The whey was drained, and the curd was placed in a mold and prepressed at the low pressure of equal weight as cheese for 60 min, which is then gradually increased from 1.0 to 3.0 bar for 3–4 h. After overnight pressing, Gouda cheese was removed from the mold and soaked in a 20% saturated brine solution at 4°C for 8 h. The cheese was allowed to dry for 3 d, and was ripened for 24 wk or longer at 12°C–15°C. The raw milk cheese was made using the above procedure without pasteurization. Samples of Gouda cheese were collected for analysis every 6 wk post manufacture.
Samples of cheese were analyzed for protein, fat, moisture, and salt content via indirect near-infrared transmittance employing the FoodScan analyzer (FoodScanTM Lab 78810, Foss, Hillerød, Denmark). For each cheese, a representative sample was homogenized, placed in a 140-mm round sample dish, and the dish was placed in the FoodScan analyzer. The pH was determined using a pH meter (B22869, Thermo Scientific, Singapore).
Acetic acid, D-lactic acid, L-lactic acid, phosphate and calcium contents were determined using a discrete photometric Thermo Scientific Gallery analyzer (Thermo Fisher Scientific Oy, Vantaa, Finland). Three grams of each cheese sample was diluted in 27 mL of sterile distilled water and centrifuged at 988×g for 10 min. Subsequently, 2 mL of each supernatant was loaded into combined sample disk with Gallery analyzer. Reagent vials are barcoded, and the barcoded system reagent containers are automatically identified. Reagent volumes are optimized for the system application. The reagent kits were listed as follows; acetic acid kit (Code 984318), D-lactic acid kits (Code 984306), L-lactic acid kits (Code 984308), phosphate kits (Code 984366 and 984368), and calcium kits (Code 984361). Automatic Gallery acetic acid application consists of two reagents (Acet R1 and Acet R2), end-point measurement, with sample blank and linear calibration curve for result calculation. First, 100 μL of acetic acid R1 reagent and 5 μL of sample were incubated for 300 s and absorbance was set to zero. After adding 25 μL of R2 reagent and incubating for 220 s, the reaction was measured at wavelength of 340 nm. The procedure was followed at 37°C. Similarly, D-lactic acid, L-lactic acid, phosphate and calcium were determined per the Gallery reference manual 1.0. These contents were determined automatically via Thermo Scientific Gallery analyzer software 4.0.
The texture properties were assessed through force-deformation curves obtained using a texture analyzer (Instron model 4465, Instron Corp, High Wycombe, UK). For texture analysis, the cheese samples from each treatment was cut into cylindrical measuring 19 mm in diameter by 26 mm (length) at 4°C to prevent barreling and were equilibrated at 20°C for 1 h prior to testing (Hort et al., 1997). The core was axially compressed 5 times to 80% of its initial height each time. The speed of the load cell was set at 120 mm/min and the following parameters were evaluated: hardness (kg), springiness (mm), cohesiveness (%), gumminess (kg) and chewiness (kg×mm).
The cheese samples were analyzed for fatty acid composition during ripening. Total lipids were extracted using the Folch solution with chloroform-methanol (2:1, v/v) (Folch et al., 1957). Each cheese samples (15 g) was homogenized with the solution (45 mL) for 10 min. Then the homogenates were filtered and centrifuged at 988×g for 10 min, and the lipids were extracted using Na2SO4 solution. An aliquot of total lipid extract was methylated Morrison and Smith’s method (Morrison and Smith, 1967). Fatty acid methyl esters thus obtained were analyzed using a gas chromatograph (Star 3600; Varian Technologies, Palo Alto, CA) with a fused silica capillary column (Omegawax 205; 30 m×0.32 mm i.d., 0.2 μm film thickness). The sample injection port was maintained at 250°C; the detector, 300°C. The results are expressed as percentages based on the total peak area.
Twenty-five grams of each cheese sample were placed in sterile Stomacher bags and homogenized in 225 mL of 0.1% peptone water, using a stomacher (FR/BagMixer; Interscience, St Nom, France) for 3 min. Serial decimal dilutions of cell suspensions were screened for the following microbial groups: total aerobes quantified using plate count agar (PCA) media incubated aerobically at 37°C for 48 h; total LAB quantified using de Man-Rogosa-Sharpe (MRS) agar with 0.005% (w/v) of bromocresol purple and 0.05% (w/v) of cysteine incubated aerobically at 37°C for 48 h; total E. coli and coliforms quantified using petri-film (3MTM PetrifilmTM 6414, MN, USA) incubated aerobically at 37°C for 48 h; total yeast and molds quantified using petri-film (3MTM PetrifilmTM 6417, MN, USA) incubated aerobically at 25°C for 5 or 7 d.
Genomic DNA of the microbes was extracted using a PowerSoil DNA Isolation Kit (Mo Bio, Carlsbad, CA) per the manufacturer’s instructions. The DNA was quantified its quality determined using Nanodrop 2000 (Thermo Scientific, Waltham, MA).
A 16S rDNA sequencing sample was prepared in accordance with the Illumina 16S Metagenomic Sequencing Library protocols (Illumina, San Diego, CA). The 16S rRNA genes were amplified using specific primers for V3-V4 regions of the 16S rRNA (f: 5’- TCGTCGGCAGCGTCAGATGTGTATAAGAGACAGCCTACGGGNGGCWGCAG-3’ and r: 5’-GTCTCGT GGGCTCGGAGATGTGTATAAGAGACAGGACTACHVGGGTATCTAATCC-3’). The template genomic DNA (12.5 ng) was thus amplified and a subsequent limited‐cycle amplification step was performed to add multiplexing indices and Illumina sequencing adapters. The final products were normalized and pooled using the PicoGreen (Invitrogen, Carlsbad, CA, USA), and the size of libraries were verified using the LabChip GX HT DNA High Sensitivity Kit (PerkinElmer, Waltham, MA). Further, sequencing was performed using the MiSeqTM platform (Illumina) per the standard Illumina sequencing protocols.
After sequencing, the PhiX sequences were removed and the primers were trimmed, and the paired-end reads were joined using the FLASH (Magoc and Salzberg, 2011). Sequences were then clustered at various percent identities using the Cluster Database at High Identity with Tolerance algorithm (Li et al., 2012). A sequence identity cutoff of 97% was used to identify operational taxonomic units (OTUs) and the results thus obtained were used in subsequent analysis. Clustered data were then evaluated for potential chimeric sequences, using UCHIME (Edgar et al., 2011).
Taxonomy was assigned to the representative sequence of each OTU, using Quantitative Insights into Microbial Ecology (QIIME, http://qiime.org/) (Caporaso et al., 2010)’s parallel wrappers for the Ribosomal Database Program (RDP) classifier. The most detailed taxonomic level assigned to an OTU’s representative sequence at a confidence level greater than or equal to 0.80 was considered the taxon of the OTU. Downstream data analysis including alpha-diversity analysis (phylogenetic information and Chao 1, Shannon, and Simpson indices), beta-diversity analysis (Principal Coordinates analysis), and canonical correspondence analysis (CCA) were conducted using QIIME or R software (v 3.0.1).
All experimental data are presented as mean±SD. Differences between cheeses during ripening were assessed using Duncan’s multiple-range tests. Statistical analysis was performed using SAS statistics program (version 9.2; SAS institute, Cary, NC, USA; 2008). Differences with p<0.05 were considered significant.
Results
Protein and fat content increased and moisture content decreased in both P-GC and R-GC during the ripening period (p<0.05). Significant differences among all the maturation periods are presented in Table 1. Protein and fat content increased significantly at 6 and 12 wk in P-GC and R-GC whereas moisture content decreased significantly during ripening, from 35.90 to 28.90 in P-GC and from 39.01 to 27.29 in R-GC (p<0.05). In addition, organic acid formation, such as acetic acid, L-lactic acid, and D-lactic acid, was significantly higher in R-GC than in P-GC (p<0.05; Table 1). As shown in Table 1, pH values increased in both groups during ripening, depending on the levels of organic acids, which decreased significantly during ripening (p<0.05).
a–d Means in the same column within the same cheese condition with different letters are significantly different (p<0.05).
The textural properties of raw milk cheese varied considerably from those of pasteurized milk cheese. Table 2 showed that levels of texture properties such as harness, cohesiveness, gumminess, and chewiness were significantly increased during ripening (p<0.05). Furthermore, the levels of these texture properties were higher in P-GC than in R-GC in the early ripening period, except for cohesiveness. During cheese ripening, the change in hardness indicated that significantly decrease in moisture content did not sufficiently compensate for the increased casein content. At the 6 wk of ripening, raw milk cheese became softer and smoother and hence, the values of hardness and gumminess, chewiness in R-GC was lower than that of P-GC in the early ripening period, due to the high proteolysis in the former type; however, the level of two groups tended to equalize as ripening progressed. The mean value of cohesiveness increased (p<0.05) from 1.46% to 1.94% in P-GC and from 1.52% to 2.07% in R-GC during ripening. Springiness refers to the height that the sample recovers between the first and second compressions after eliminating the deforming force (Patel et al., 1993). Changes in gumminess were similar to changes in hardness for both P-GC and R-GC for similar reasons deduced for reduction in moisture content during ripening. Chewiness, defined as the energy required to masticate a solid food item to a state ready for swallowing, was the product of hardness, cohesiveness and springiness (Patel et al., 1993). The mean value of chewiness displayed a significant rapid increase for both P-GC and R-GC during ripening (p<0.05).
a–d Means in the same column within the same cheese condition with different letters are significantly different (p<0.05).
Fatty acid content in P-GC and R-GC was similar. During ripening, EPA (C20:5n3), nervonic acid (C22:4n6), and DHA (C22:6n3) were low in both P-GC and R-GC (Table 3). However, major fatty acids displayed significant differences during ripening. Myristic acid (C14:0) ratio was 11.87% in R-GC and 11.14% in P-GC on week 6 of ripening. As ripening progressed, a significant reduction (C14:0) of 0.19 units was observed in R-GC, whereas P-GC displayed a significant increase of 0.78 units (p<0.05). Palmitic acid (C16:0) ratio in the cheese was determined to be 40.78% in R-GC and 37.96% in P-GC in the 6 wk of ripening, and there were significant increases of 0.68 and 3.47 units in R-GC and P-GC, respectively, after ripening (p<0.05). Stearic acid (C18:0) in R-GC was 14.59% and 13.92% in P-GC at the beginning of ripening (6 wk), and increased to 15.24% and 15.01%, respectively, at 18 wk of ripening (p<0.05). Oleic acid (C18:1n9) ratio increased from 27.13% at 6 wk of ripening to 30.43% at 12 wk and finally to 27.42% after ripening in R-GC, and decreased gradually from 31.53% to 27.38% in P-GC, during ripening. Interestingly, the changes in saturated fatty acid (SFA) ratio between 64.34% and 68.04% in R-GC, and between 63.02% and 67.96% in P-GC during ripening (p<0.05). Conjugated linoleic acid (CLA) ratio was 2.17%–2.32% in P-GC and 2.20%–2.45% in R-GC; however, this ratio did not differ significantly between the two cheese groups. Medium- and long-chain fatty acids in R-GC and P-GC were 51.23%–54.85% and 52.09%–54.88%, and 45.15%–48.77% and 45.12%–47.91%, respectively.
a–d Means in the same row within the same cheese condition with different letters are significantly different (p<0.05).
Results of aerobic bacteria count (APC), LAB count, coliforms count, E. coli count, and yeast/mold counts are shown in Table 4. The viable cells of LAB in R-GC, with the highest levels observed at the beginning (6 wk), were significantly decreased at the end of the ripening period (p<0.05). The counts of LAB were higher in R-GC than in P-GC (p<0.05), owing to presence of NSLAB derived from raw milk. All cheese samples contained detectable amounts of coliforms; however, as ripening progressed, the counts of total coliforms were gradually decreased until at 24 wk of ripening. Importantly, E. coli was detected in only R-GC at the beginning of ripening (6 wk) and not throughout; E. coli were undetectable after 12 wk of ripening. The counts of yeasts and molds in P-GC during ripening significantly decreased until 12 wk and significantly increased at the end of the 24-week period (p<0.05). The counts of these microbial populations were similar in R-GC and P-GC.
a–d Means in the same column within the same cheese condition with different letters are significantly different (p<0.05).
For exploring dynamics of microbial community, we choose on sampling points with initial time point (6 wk) and aged time point (24 wk) during ripening. Samples of R-GC and P-GC at 6 and 24 wk of ripening were analyzed for microbial diversity, via sequencing analysis (Supplementary Table S1). A total of 628,952 bacterial sequence reads with an average of 78,619 sequence reads per sample were obtained from P-GC and R-GC. As an estimator of sampling completeness, goods coverage index for each data set was >99% in all samples.
The 16S rRNA gene sequencing reads were classified into different taxonomies. The relative abundance of R-GC and P-GC samples during ripening periods are indicated as phylum, order, family, genus, and species levels (Supplementary Table S3 and Fig. 1). At the phylum level, no significant differences in the proportion of phyla between the center and the surface of the two cheeses were observed. All cheese samples were dominated by Firmicutes, with >96.36% (P-GC) and >95.57% (R-GC). At the family level, all cheese samples were dominated by Streptococcaceae and Leuconostocaceae. The center of P-GC was dominated by >76.04% and >76.33 Streptococcaceae and Leuconostocaceae, respectively, and the surface contained >19.77% and >18.19%, respectively. Streptococcaceae increased from 76.04% to 76.33% (center) and decreased from 77.99% to 67.92% (surface) during ripening, and the proportion of Leuconostocaceae increased from 19.77% to 28.96% (center) and 18.19% to 20.42% (surface) in P-GC. R-GC was dominated by Streptococcaceae, with >87.15% (center) and >87.37% (surface), and the proportion of the family decreased during ripening. The proportion of the family decreased from 92.34% to 87.15% (center) and 92.58% to 87.37% during ripening. The second most abundant family, Leuconostocaceae increased from 3.18% to 11.19% (center) and 3.41% to 11.47% (surface), identified 3-fold higher than Leuconostocaceae at 6 wk.
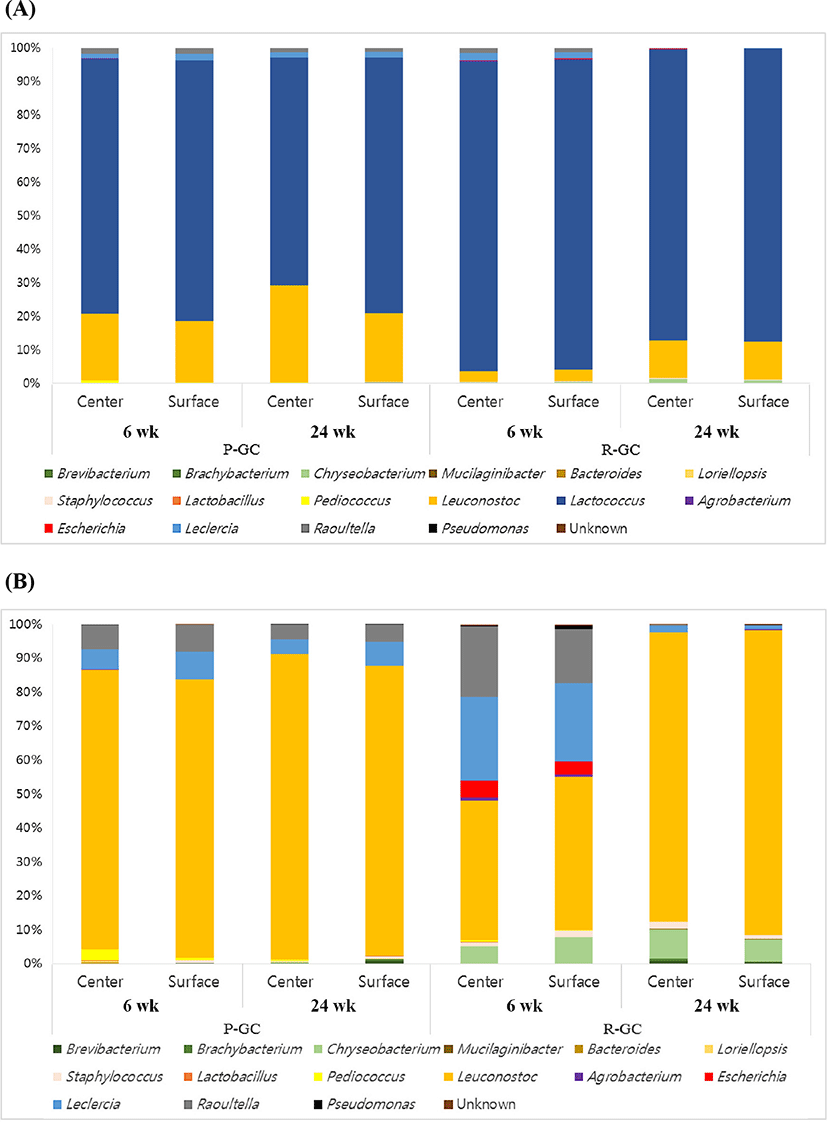
Pyrosequencing revealed that 16 genera (relative abundance >0.01%) of Lactococcus, Leuconostoc, Lactobacillus, Pediococcus, Staphylococcus, Brevibacterium, Brachybacterium, Chryseobacterium, Mucilaginibacter, Bacteroides, Loriellopsis, Agrobacterium, Escherichia, Leclercia, Raoultella, and Pseudomonas were identified in Gouda cheese. The most abundant genus was Lactococcus in all cheese samples. The type of starter culture used for the manufacture of Gouda cheese was Leuconostoc, the second most dominant genus. The taxonomic classification of bacteria in R-GC and P-GC at species level is presented in Supplementary Table S3 and Fig. 2. The Lc. lactis, Lc. laudensis, and Leuconostoc pseudomesenteroides comprised most of the microbial community in Gouda cheese. Lc. lactis and Lc. laudensis tended to decrease with ripening, except that Lc. laudensis slightly increased from 0.31 to 0.36 on the surface of R-GC. P-GC had a higher proportion of L. plantarum than R-GC, which decreased during ripening. However, Leuconostoc pseudomesenteroides increased as ripening progressed.
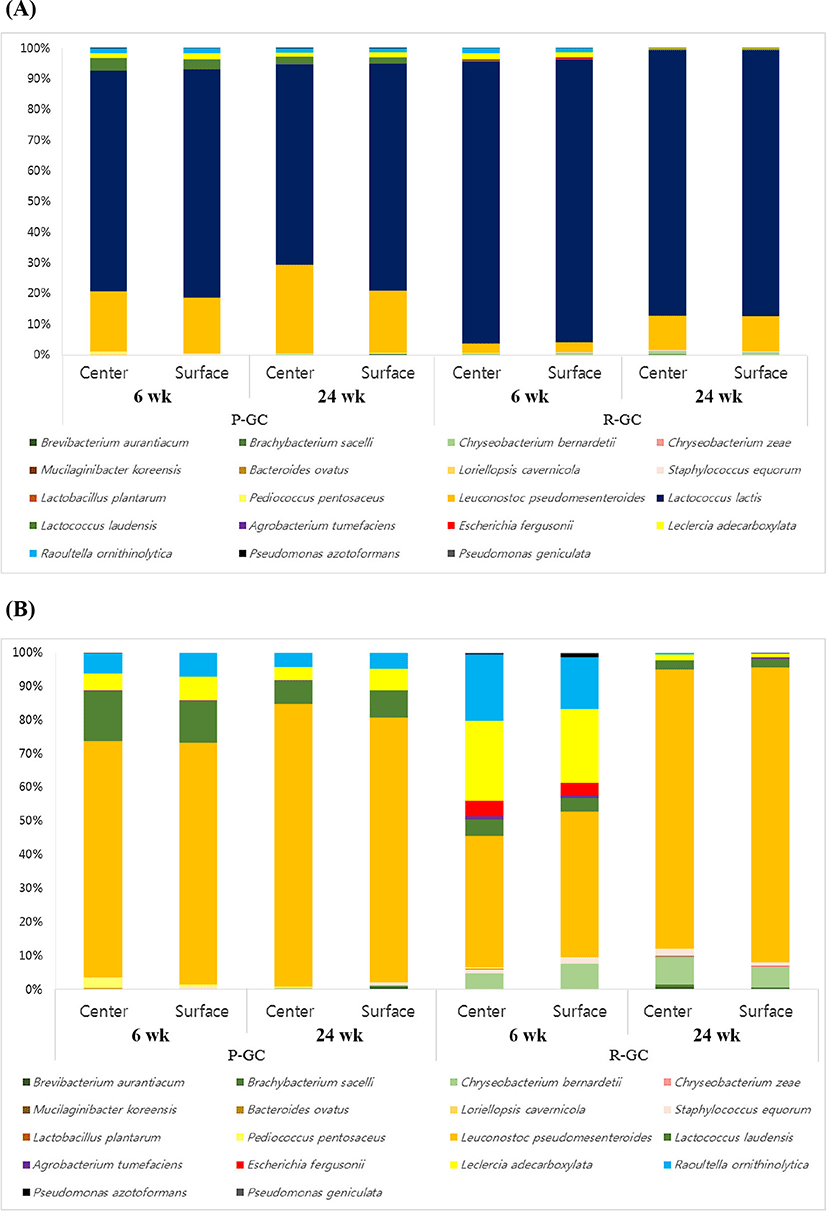
In addition, as shown in Fig. 3, the CCA for bacterial communities depending on the region of Gouda cheese showed no discernible trend during ripening. However, microbial diversity of P-GC and R-GC differed significant during ripening.
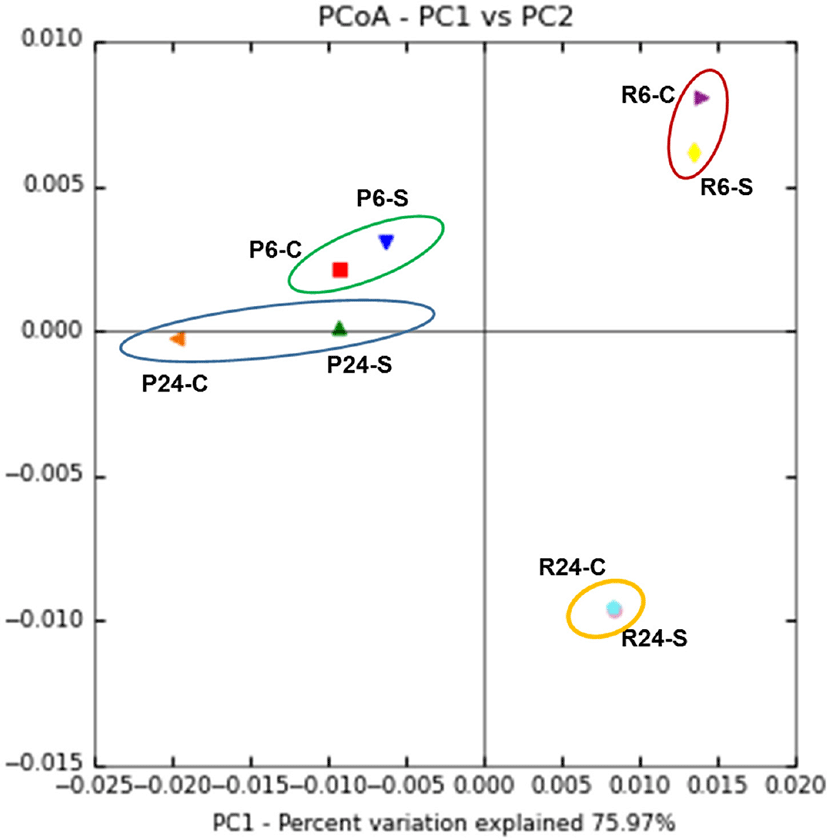
Discussion
Pasteurized milk cheese (P-GC) revealed slightly higher moisture than raw milk cheese (R-GC) during ripening period (Table 1). The moisture content decreased in all cheeses throughout the storage period. The fat content was slightly lower in P-GC than in R-GC, while it increased during ripening period as a result of the decrease in moisture content (Table 1). Similar to these results, Salwa and Galal (2002) reported similar trends in raw milk cheese in comparison with the heat treated and pasteurized milk cheese in the manufacture of Egyptian Domiati cheese. A moisture decrease by evaporation led to a progressive weight decline and afterwards there was a progressive dehydration during the cheese maturation (Papetti et al., 2012). In addition, it probably owing to proteolysis and lipolysis (Zaki et al., 1975). At early ripening, L-lactic acid prevailed due to the predominance of LAB belonging to the used starter, which transforms lactose, mainly into L-lactic acid (Diezhandino et al., 2015; Thomas and Crow, 1983). Normally, a reduction in pH is expected at the initial stage of ripening because of residual lactose being metabolized to lactic acid, followed by an increase in pH depending on the type of cheese (Fox et al., 1993). Generally, values for theses parameter of texture properties gradually tended to increase during ripening depending on the moisture loss (Table 2). As ripening progressed, hardness was lower in R-GC than in P-GC, in particular, at the first 6 wk of ripening (p<0.05). This could be mainly due to the higher proteolysis in R-GC than in P-GC. Proteolysis is the most complex process, contributes to the development of cheese texture from hydrolysis of the protein matrix and an increase in the pH due to liberation of ammonia from amino acid. Hardness have been reported to have a good correlation with proteolysis (Fedrick, 1987).
The results of major fatty acids content (Table 3) are similar to those of a previous study, wherein myristic acid ratio in Tulum cheeses made from pasteurized milk was 9.74% at the beginning of ripening and 10.54% on the 180th day of storage, while that in raw milk cheese was 9.59% and 10.36% (Sert et al., 2014). The value of medium- and long-chain fatty acids in R-GC and P-GC were not differ significantly and hence they do not essentially contribute to the aroma of the cheese (Buffa et al., 2001; Freitas and Malcata, 1998). These results corroborate the fact that lipases (either from milk, rennet, or microbes) involved in cheese ripening display higher specificity towards short- and medium-chain fatty acid residues than towards long-chain residues (De la fuente et al., 1993). Short-chain fatty acids are known to have an important effect on cheese aroma (Attaie and Richter, 1996). Especially, lipolysis in milk preferentially releases short- and medium-chain fatty acids, because in milk triglycerides, short-chain fatty acids are esterified predominantly at the sn-3 position (Singh et al., 2003). Milk lipase appears to hydrolyze the fat selectively and is able to act on triglycerides, while lactococcal lipases seem to be active mainly on mono- and di-glycerides (Stadhouders and Veringa, 1973). The lipases of Lactobacillus and Lactococcus are specific for short-chain fatty acids, which accounts for the greater abundance of the free forms of such acids in cheeses (El Soda et al., 1986). Lipase and esterase activities have been detected in cell-free extracts of numerous Lactobacillus and Lactococcus species and a preference for short-chain fatty acids has been observed for lactococcal and lactobacilli lipases (El Soda et al., 1986; Kamaly and Marth, 1989; Singh et al., 1973). Lipolysis is of great importance for cheese ripening, and profiles of short- and medium-chain free fatty acids (FFA) are often analyzed during chemical characterization of the extent of cheese ripening (De la fuente et al., 1993; Downey, 1980; Woo et al., 1984).
Throughout the manufacturing and ripening process, especially in the late steps, LAB such as lactobacilli prevailed and played an important role in cheese production and could be part of the starter culture (de Souza et al., 2003). The reduction of LAB during ripening may have resulted from brine processing to inhibit the growth of these microorganisms or putrefactive bacteria such as coliform bacteria. The previous study reported that pasteurization, pH, water activity, milk type, and rind type were significantly associated with detection of coliforms in cheese, e.g., a higher coliform prevalence was detected in raw milk cheeses (42% with >10 CFU/g) than in pasteurized milk cheese (21%) (Trmcic et al., 2016). Also, the counts of molds and yeasts were similar to those reported previously (Centeno et al., 1994; Menendez et al., 2001), however, other studies reported that the counts of molds and yeasts from artisanal Serrano cheese during from 7–60 d were 0.67–4.70 Log CFU/mL for molds and 3.72–6.04 Log CFU/mL for yeast, decreasing during ripening period (de Souza et al., 2003). As ripening progresses, cheese matrix compaction increases, which reduces the diffusion of the oxygen required for the growth of these microbial populations (de Souza et al., 2003).
Interestingly, the proportion of coliform bacteria such as E. fergusonii, Leclercia adecarboxylata, Raoultella ornithinolytica, Pseudomonas azotoformans, and P. geniculate tended to decrease during ripening regardless of the center or surface in P-GC and R-GC, compared to those until 6 wk (Supplementary Table S2 and Fig. 2). In particular, E. fergusonii was only identified in R-GC; however, E. fergusonii was rarely identified as ripening progressed. Detection of coliform bacteria in finished products, including cheese, has traditionally been used to indicate unsanitary manufacturing conditions. The coliform bacteria include some thermotolerant coliforms genera, such as Serratia, Hafnia, Rahnella, and Leclercia. The coliforms detected represented environmental coliforms and were shown recently to include at least some strains able to grow in milk at refrigeration temperatures (Masiello et al., 2016; Trmcic et al., 2016), and Escherichia, Hafnia, and Enterobacter were significantly more common in raw milk cheese (Trmcic et al., 2016).
This study presents a first look at the bacterial communities of Gouda cheese manufactured from raw and pasteurized milk during cheese ripening through next-generation sequencing. Our findings offer insight into cheese microbiota composition, with raw and pasteurized milk cheese exhibiting unique bacterial diversity at 6 and 24 wk of ripening. However, more effort is needed to be taken into account to address best production process of raw milk cheese where no pasteurization related to the dynamics of microbial communities for the cheese quality during ripening. In conclusion, our result provides significant evidence on the potential use of raw milk in manufacturing and ripening of Gouda cheese and evaluation for risks and benefits of raw milk cheese during manufacturing. Further studies are required to investigate the optimal minimum ripening period by cheese types for assurance of consumer safety.