Introduction
Legume seed proteins have been generally used as nonmeat ingredients to improve the water holding capacity and water binding ability of meat products, as well as to boost nutrition (Asgar et al., 2010). Several studies have focused on the 7S globulin of soy, red bean, and mungbean to evaluate the functional and structural interactions between food proteins. Physicochemical, functional, and structural characteristics of these proteins and their 7S globulins were investigated by evaluating the heat stability, protein solubility, pH, surface hydrophobicity, emulsion stability, and secondary structures (Tang and Sun, 2011). Mundi and Aluko (2012) extracted globulins from kidney bean protein fractions and reported higher gelling properties and emulsion stability than albumin. This is partially due to the increased surface hydrophobicity of globulin, which might increase the gelling properties induced by protein-protein interactions.
Red bean (Vigna angularis, RB; adzuki bean or pat) protein consisted of 7S and 11S globulins, and contains large amounts of essential amino acids (Chau and Cheung, 1998). Red bean protein isolate (RBPI) has been suggested as a useful substrate for improving the water binding ability and textural properties, as well as emulsion stability in different addition levels, pH values, and salt concentrations to optimize processing conditions (Jang and Chin, 2011) when incorporated into myofibrillar protein (MP) gels mediated by microbial transglutaminase (MTG). Because MP gels can be affected by processing conditions including pH, salt concentration, temperature, and functional ingredients, their optimized application has attracted research interest (Chin et al., 2009a; Chin et al., 2009b; Doerscher et al., 2003; Kerry et al., 1999; Ramirez-Suarez and Xiong, 2003).
Although there have been many studies on the functionality of legume seed proteins, the effects of fractioning (7S-globulin of RBPI) and/or preheating treatment (pre-heating; 95℃/30 min) on the rheological properties of MP gels induced by MTG are unclear. Thus, the objective of this study was to evaluate the effects of RBPI on the rheological properties of MTG-mediated MP gels as affected by fractioning and/or preheat treatment.
Materials and Methods
Approximately 200 g of pork loin meat (crossbred pigs: (Landrace × Large Yorkshire) × Duroc; grade A, 110 kg live weight; Korea) was prepared as cubes (1-2 cm3), which were trimmed of excess fat and connective tissues. The cubes were vacuum-packed and frozen at −20℃ until used. MP was prepared from pork loin cuts by homogenization and extraction with buffer solutions (0.1 M NaCl and 50 mM NaH2PO4; pH 6.25), and then finally obtained after centrifugation (J2-21, Beckman Inc., USA) at 1000×g for 15 min (Xiong, 1993). MP concentration was adjusted to 40 mg/mL using the Biuret method (Gornall et al., 1949). RBPI was prepared from red bean (Saecharmdeul Co., Korea) according to the previous study (Jang and Chin, 2011; Kim et al., 1990). RBPI 7S globulin protein was prepared by fractioning (Hong et al., 2012) and/or preheating at 95℃ for 30 min (pre-heating RBPI and pre-heating/7S-globulin) (Fig. 1). For MTG (ACTIVA TG-TI, 1% enzyme and 99% maltodextrin, 1 U/g activity; USA)-mediated MP gels, 1% of MTG was added to MP samples with 4 h incubation (Sakamoto et al., 1994).
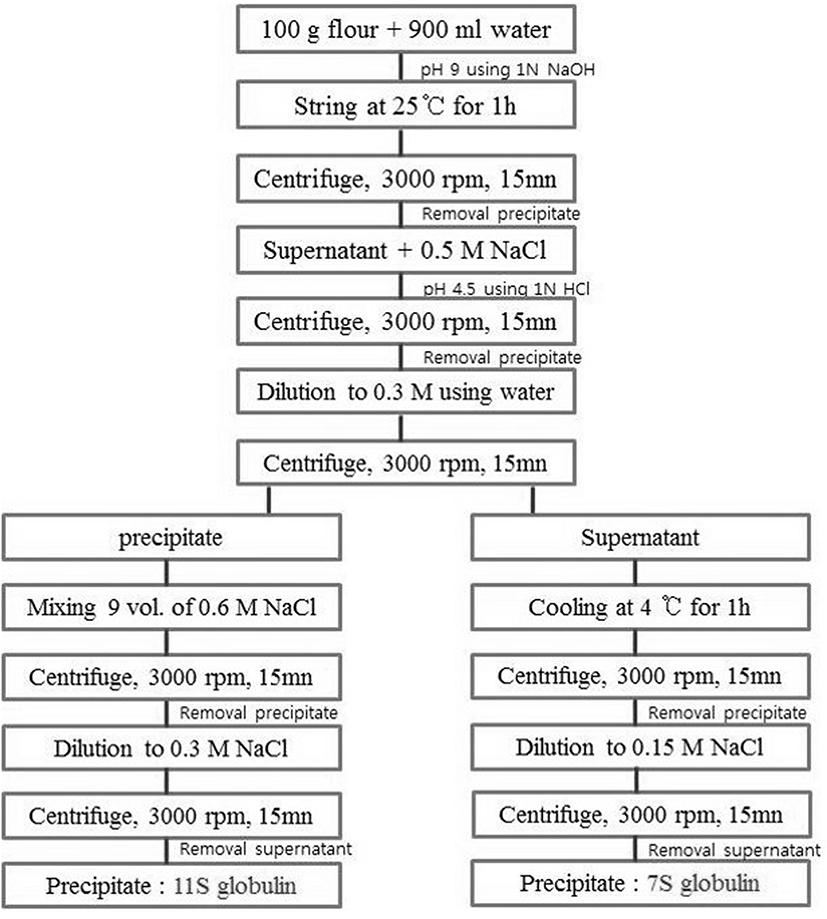
MP (0.45 M salt, pH 6.25) was admixed with/without MTG (1%) and preheat treatment of RBPI in a Bowl RestTM food mixer (Hamilton Beach/Proctor-Silex, Inc., USA) (Table 1). MP mixtures (5 g) were loaded into glass vials, and refrigerated for 4 h to react with MTG (Sakamoto et al., 1994). Heat-induced gels were prepared by cooking with increasing temperatures from 20 to 80℃ at a rate of 3℃/min. After chilling the heat-induced MP gels in an ice/water, the gels were stored at 4℃ until analyzed within 2 d.
Treatments: pork myofibrillar protein (MP) gels mediated by microbial transglutaminase (1% MTG) with or without 1% red bean protein isolate (1% RBPI) as affected by fractioning (7S-globulin) and/or preheating treatments (pre-heating RBPI or pre-heating/7S-globulin).
After heating, cooking yield (CY, %) and gel strength (GS, gf) of heat-induced MP gels were evaluated. CY was measured by weight differences between before and after cooking as:
Cooking loss (CL, %) = (A − B) / A × 100
Cooking yields (CY, %) = 100 − CL
A: weight of uncooked MP; B: weight of cooked MP.
GS was measured by compression test using an Instron Universial Testing Machine and the Merlin program (Instron, USA) with a 500 N load cell at 50 mm/min cross speed. The samples were equilibrated to room temperature for the puncture test using a 9 mm diameter puncture probe. When the heat-induced, chilled, and equilibrated MP gels were ruptured after compression test, the first peak value of breaking force (gf) was determined as the GS (Lee and Chin, 2013).
As shown in Table 1, MP mixtures prepared with RBPI and 7S-globulin, and preheating were used for SDS-PAGE using a Mini-PROTEAN 3 Cell system (Bio-Rad Laboratories, USA) with a 10% separating and a 4% stacking gels (0.375 M Tris, pH 8.8 and 0.125 M Tris, pH 6.8, respectively) (Laemmli, 1970). Electrophoresis was run at 150 V for 1.5 h with raw extracted protein samples diluted by sample buffer (4% SDS, 20% glycerol, 20% mercaptoethanol, and 0.125 M Tris, pH 6.8) to load the protein (1%, μg/μL) per each well. After run, the gels were dyed using Commassie Brilliant Blue R-250 (Bio-Rad Laboratories, Hercules, USA) during shaking for 30 min and then, de-stained 3 times with 1 h interval using a model RK-120 rocker (New Power Co., Korea). Standard marker consisting of pre-stained SDS-PAGE standards (Bio-Rad) was used to calculate the molecular weight of bands induced from each samples.
SEM was performed to observe the differences of three-dimensional structure among treatments using a JSM-7500F microscope (JEOL Ltd., Japan) as previously described (Jang and Chin, 2011). According to prior methods for sample preparation (Haga and Ohashi, 1984), cubic samples (3 mm3) were fixed by soaking for 24 h in 2.5% glutaraldehyde buffer solutions (0.1 M sodium phosphate buffer with glutaraldehyde, pH 7.0) at 4±1℃. A post-fixation procedure was followed with osmium tetraoxide (OsO4) buffer solution. Post-fixed samples were washed and dehydrated by dipping in increased levels of ethanol (50, 60, 70, 80, 90%, and three times with 100%) and acetone for 10 min per each step (Jang and Chin, 2011). To observe the microstructure (magnification, ×2000), samples were gold-coated and then measured using a SEM analyzer at 15 kV.
All experiments were performed in triplicate (n=3). SPSS version 20.0 (SPSS, USA) was used to analyze the statistical differences among treatments by Two-way ANOVA (Lee and Chin, 2013). Post-hoc testing was performed to compare the means using Duncan’s multiple range test when statistical significances were found at p<0.05.
Results and Discussion
CY of MP gels was decreased with the addition of 1% MTG (p<0.05; Fig. 2). The addition of MTG decreases CY due to the MTG catalyzed protein-protein interactions rather than protein-water interaction (Hong and Chin, 2010). However, presently the addition of 1% RBPI improved the CY of the MP gels, while fractioning (7S-globulin) decreased the effects of RBPI on the CY of MP gels (p<0.05; Fig. 2); however, preheat treatment (95℃/30 min) did not affect the CY of MP gels (p>0.05; Fig. 2). These results indicated that the water-soluble proteins of RBPI may affect the increased protein-water interaction, resulting in the improving CY, while the preheating procedure did not improve the cooking yield, since the hydrophobic interaction induced by preheating did not significantly change the CY of MP gels (Fig. 2). Hongsprabhas and Barbut (1999) reported that the addition of whey protein prepared with preheat treatment increases the water holding capacity of cold-set gels and reduces the cooking loss of poultry meat products. Thus, pre-heating may or may not be advantageous for the water holding capacity of processed meat during heating, depending on the conditions. The increased temperature or heating time might be improved the water binding capacity during heating or storage.
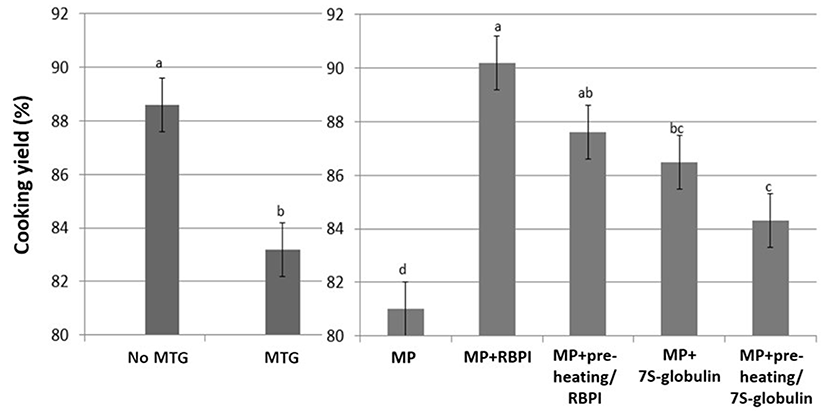
For the gel strength (GS, gf), RBPI addition may negatively affect the GS of MP without 1% MTG, while RBPI increased the GS of MTG-mediated MP (Fig. 3; p<0.05). Although RBPI was used as a substrate for MTG-mediated MP gels by increasing the protein-protein interactions, the hydrophilic fraction of RBPI may negatively affect the gelling property of MP alone. Sun and Arntfield (2012) reported that MP with 1% pea protein mixtures induced by MTG has a higher GS than MP alone. Vicilin-rich protein isolates with MTG improves crosslinks between inter- and intra-molecular interactions (Tang, 2008). In the same manner, pre-heating 7S treatment increased the GS of MP induced by MTG; however, pre-heating treatments decreased the GS of MTG-mediated MP with RBPI or 7S-globulin (Fig. 3; p<0.05), even though MTG-mediated MP control was similar to preheated treatments (pre-heating RBPI or pre-heating/7S-globulin) (Fig. 3; p>0.05). These results indicated that the protein-protein interactions and hydrophobic interactions induced by folding proteins during gel setting process might improve gelling properties; however, the changes of RBPI residues induced by preheat treatment might affect the GS in MTG-mediated MP. Chanarat and Benjakul (2013) reported that MTG increases the GS of fish meat products; however, the addition of formaldehyde negatively affected the cross-links induced by MTG. Since the formaldehyde decreased the amounts of amino groups, the cross-links between protein residues catalyzed by MTG were decreased, while the fractioning might contribute the increased protein-protein interactions of MP gels, regardless of MTG treatment (Chanarat and Benjakul, 2013).
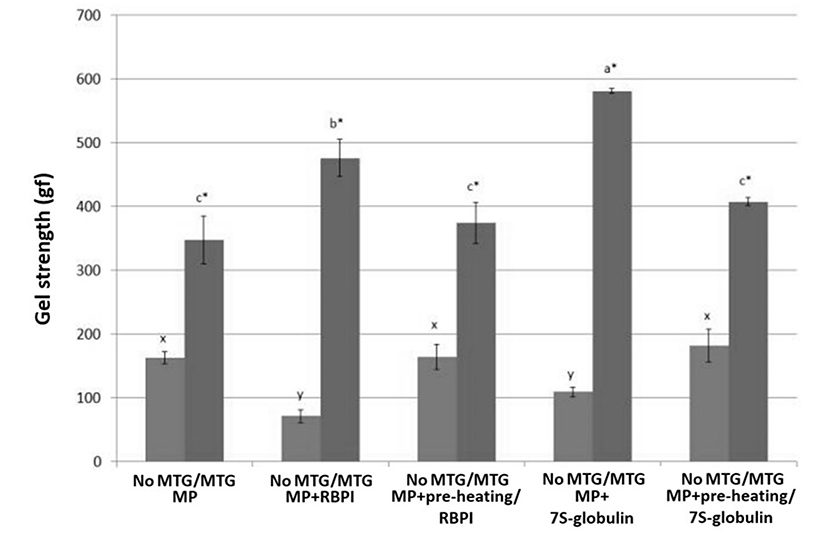
The addition of 7S showed the highest GS with addition of MTG-mediated MP mixtures. These results indicated that 7S-globulin could contribute to the increased protein-protein interaction when MP was catalyzed by MTG during gelling process. Tang (2008) reported that 7S-globulin had two different endothermic denaturation temperatures (87.7 and 94.1℃) and that disulfide bonds did not contribute to increase the gelling property; however, hydrophobic and hydrogen bonds mainly affected the gelation. Mundi and Aluko (2012) reported that globulin of kidney bean had higher gel forming ability and emulsion stability than albumin. The exposures of hydrophobic residues induced by heating might increase the protein-protein interactions in globulin of kidney beans and increased the gel forming ability. However, legume seed proteins generally decreased the textural properties of salt-soluble protein gels (Sun et al., 2012). Based on the results, chemical modifications of RBPI should be done to optimize the gelling property of MP mediated by MTG. However, the preheating procedure decreased the gel strength of both MP with RBPI or 7S-globulin.
The effects of RBPI on the SDS-PAGE profiles of MP with or without 1% MTG as affected by fractioning and modifications are shown in Fig. 4. RBPI can be divided into acidic (38-45 kDa) and basic subunits (11-24 kDa) having 7S vicilin and 11S legumin, respectively. The major bands of 7S vicilin was clearly found around at 38-45 kDa (Fig. 4A). Mundi and Aluko (2013) reported that the vicilin of kidney beans was found at 43 or 45 kDa in SDS-PAGE profiles. The major band of 7S-globulins was condensed as compared to that of RBPI, regardless of preheat treatment in MP (Fig. 4B). Without incubation, MHC and major band of 7S-globulin clearly remained and there was no polymerisation to form biopolymer on the stacking gel, which could be formed by interactions between MP and functional proteins catalyzed by MTG treatment (Fig. 4C). MHC disappeared when 7S-globulin was added to MTG-mediated MP, while MHC of other treatments remained; however, the major band of 7S-globulin was observed when it prepared with preheat treatment (Fig. 4D). These results indicated that interactions between 7S-globulin and MTG-mediated MP gels was highly activated, resulting in highest GS; however, preheating might diminish the effects of 7S-globulin, resulting in lower GS of MTG-mediated MP gels.
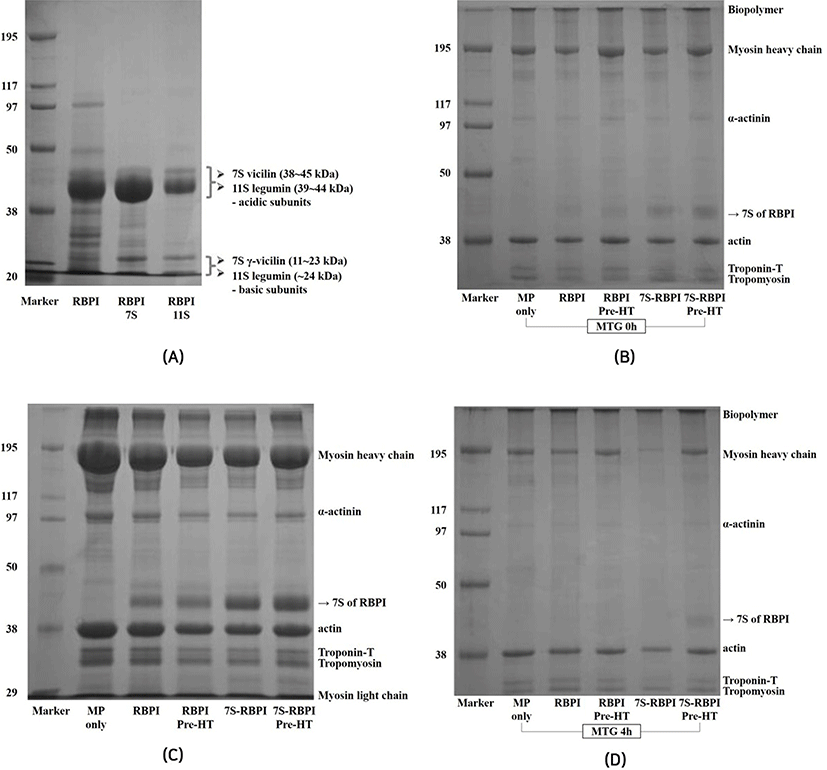
Microstructures of heat-induced MP gels with or without 1% MTG treatment as affected by the addition of 1% RBPI and modification are shown in Figs. 5 and 6, respectively. When RBPI was incorporated into MP gels, the microstructure became more compact regardless of modifications (fractioning and/or preheating treatments) of RBPI (Fig. 5). These results indicated that the modification of RBPI might change the role to form a gel structure of MP. Sun et al. (2012) confirmed the strengthened gel structures by the addition of peanut proteins in chicken salt-soluble protein gels. When both RBPI and 7S-globulin were incorporated into the MTG-mediated MP gels, void structures became filled and more uniform, and flat surface structures formed; however, preheating changed the surface structure and broke the strongly flat structures of MTG-mediated MP gel prepared with RBPI or 7S-globulin by changing the active sites of proteins (Fig. 6). Meng and Ma (2002) also reported that red bean globulin protein was changed by different heating temperatures between 90 and 95℃. When the preheating temperature was close to the endothermic denaturation temperature of 95℃, the gel structure became more compact and a strongly cross-linked structure was observed (Meng and Ma, 2002). This result also supported the appearance of more cross-linked structures were observed when RBPI was prepared with preheating at 95℃ to improve the protein-protein interaction during gel setting of MP mixtures.
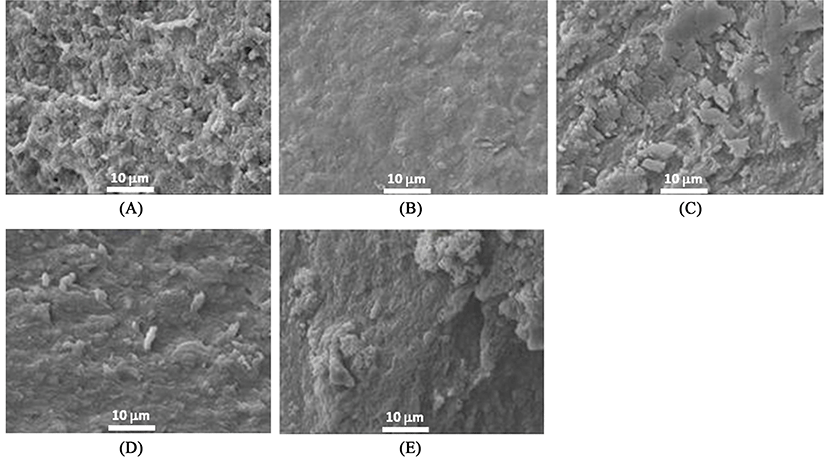
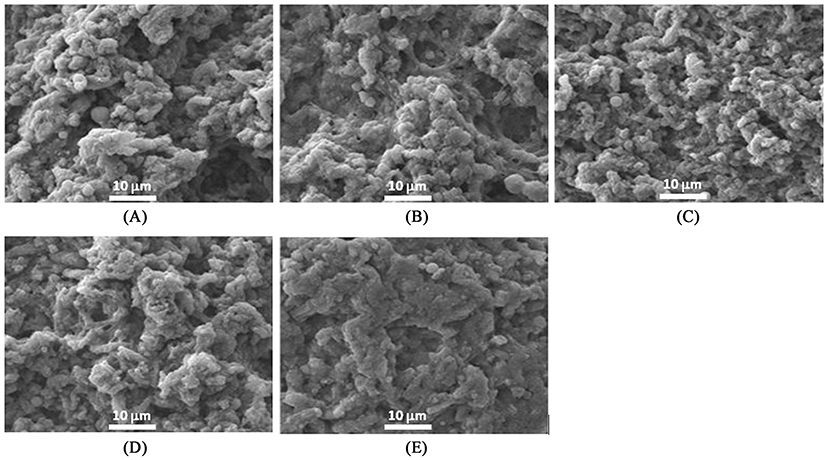
Conclusions
RBPI and 7S-globulin (fractioning) improved the GS of MTG-mediated MP, while RBPI improved the CY, regardless of modifications. These results was confirmed by compacted surface structures in SEM, and major band disappearances of RBPI in MP gels. However, the stable surface structures of MTG-mediated MP gels were changed and broken by the addition of RBPI or 7S-globulin treated by pre-heating. Thus, RBPI and 7S-globulin are suggested to improve the rheological properties of MTG-mediated MP gels as a substrate for MTG to function as a water and meat binder.