Introduction
Muscle fiber characteristics account for meat quality regardless of livestock species, thus numerous studies have been conducted to date (Hwang et al., 2010; Larzul et al., 1997; Ozawa et al., 2000; Ryu et al., 2008). Most of these studies are based on myosin ATPase reaction for fiber typing which is convenient and simple, and allows the muscle fibers to be subdivided into three or four types (I, IIA, IIB or IIX) (Brooke and Kaiser, 1970; Guth and Samaha, 1969). Myosin heavy chain (MHC) distributionbased fiber typing is also a useful method for muscle fiber typing, because it allows more specific delineation of muscle fiber types as well as distinction between pure (fiber consisting of one MHC isoform) and hybrid (fiber consisting of two or more MHC isoforms) fiber types (Acevedo and Rivero, 2006; Lefaucheur et al., 2002). Moreover, myosin ATPase is localized to the MHC and its activity depends on the MHC isoforms (Levitsky, 2004). Previous studies in relation to MHC-based fiber characteristics were mainly conducted in pork (Abreu et al., 2006; Chang et al., 2003; Kim et al., 2013; Lefaucheur et al., 2002), with only a few reports in bovine skeletal muscle (Gonzalez et al., 2009; Greenwood et al., 2009; Jurie et al., 1998). Gonzalez et al. (2009) evaluated the effect of ractopamine hydrochloride supplementation on the MHC distribution in bovine muscles and observed longissimus and SM muscles having ranges of 27.5-32.5% type I fibers and 67.5-72.5% type II fibers. Greenwood et al. (2009) reported changes from 25 to 28% type I fibers and from 55 to 45% type IIX fibers in the longissimus lumborum muscle with a postweaning nutritional treatment. Jurie et al. (1998) investigated the influences of housing type on muscle fiber compositions in LT and ST muscles. MHC-based fiber distribution has been well studied in bovine skeletal muscles; however, defining the fiber types and their distributions in bovine skeletal muscles remains a challenge.
Primal or subprimal cuts composed of one or more muscles have a distinct meat quality due to the muscle fiber composition, which is basically determined by the physiological function of muscle (Arbanas et al., 2009). In the previous report (Hwang et al., 2010), the clear differences in the muscle fiber characteristics of three bovine skeletal muscles and their relationships to beef quality were investigated. Kirchofer et al. (2002) evaluated the muscle fiber characteristics and meat quality of 12 samples of beef round cut and 26 samples of beef chuck cut. However, these were based on the myosin ATPase activity or both succinate dehydrogenase activity and myosin ATPase activity methods. Because MHC-based fiber type IIX can be considered as either IIA or IIX based on myosin ATPase activity (Kim et al., 2014), MHC-based fiber characteristics and their relationships on beef quality could be different from those based on myosin ATPase studies.
Therefore, the purpose of this study is to investigate the different characteristics of MHC-based muscle fiber among four major bovine skeletal muscles and consequently, the differences in beef quality. Immunohistochemistry using commercial monoclonal antibodies specific to bovine MHCs was conducted to classify the MHC-based fiber types. Prior to muscle fiber typing, identification of the MHC isoforms distributed in bovine skeletal muscles was performed by LC-MS/MS analysis.
Materials and Methods
All procedures involving animals were approved by the Animal care and Use Committee of the Gyeongsang National University, Republic of Korea and the cattle were slaughtered according to the standard commercial procedures of the Korean livestock production system. Four bovine skeletal muscles (LT, PM, SM and ST) were obtained from eighteen Hanwoo (Korean native cattle) steers (653±48 kg body weight at 28 mon old) at 24 h postmortem from a commercial slaughter house. LT muscles were taken from the 8th-9ththoracic vertebrae, whereas the other muscles were taken from their central parts. Three pieces (0.5 × 0.5 × 1.0 cm) were cut from the central part of each muscle and promptly frozen using 2-methylbutane cooled with liquid nitrogen for immunohistochemistry (IHC) and LC-MS/MS analysis. The rest was used for analysis of meat quality.
Preparation of myofibrillar proteins for LC-MS/MS analysis was conducted by the Talmadge and Roy (1993) method with modification. In brief, eighty transverse sections (10 μm thickness) were collected using a cryostat microtome (CM 1860, Leica Biosystems, Germany) at -21℃ and myofibrillar proteins were extracted in 1.0 mL of lysis buffer consisting of 75 mM KCl, 10 mM K2HPO4, 2 mM MgCl2 and 2 mM EGTA (pH 7.0) by sonication (VCX750, Sonics & Materials, Inc. USA; five ultrasonic bursts of 2 s each with 2 s interval). The myofibrillar pellet was obtained by centrifuging at 10,000 g for 60 min followed by removing the supernatant. The pellet was resuspended in 500 μl of buffer (7 M urea and 2 M thiourea) and centrifuged at 10,000 g for 30 min. The protein concentration was determined by the Bradford (1976) method. Reduction and alkylation were conducted with 5 mM DTT at 37℃ for 45 min and 25 mM iodoacetamide at RT for 1 h, respectively. In-solution digestion was performed using trypsin (Promega Co., USA) at 37℃ for 16 h. The tryptic digest was analyzed using a nano-LC coupled to a LTQ-Orbitrap mass spectrometer (Applied Biosystems, USA). The digest was loaded onto a capillary column (150 mm × 0.1 mm) packed with Magic C18 resin (3 μm, 100 Å pore, Michrom BioResources, USA). Mobile phase A consisted of 3% acetonitrile and 0.1% formic acid in deionized water while mobile phase B consisted of 0.1% formic acid in acetonitrile. Peptide separation was conducted by a linear gradient (0% to 5% phase B for 1 min, 5% to 30% B for 45 min, 30-60% B for 5 min, 95% B for 5 min and 0% B for 5 min). The LC-MS/MS raw data was submitted to the MASCOT algorithm (ver 2.1, Matrix Science, UK) and MHCs were identified by searching the peak list against the NCBInr database (91558 entries for Bos taurus downloaded on January 2015). The database search criteria were as follows: carboxyamidomethylation of cysteine as a fixed modification; oxidation at methionine as a variable modification; maximum missed cleavage, 2; peptide MS tolerance, 1.2 Da; fragment MS tolerance, 0.6 Da.
The transverse sections of each sample were cut at 10-μm-thickness using a cryostat microtome (CM 1860, Leica Biosystems, Germany) at -21℃. The sections were blocked with normal goat serum (Santa Cruz Biotechnology, Inc., USA) and incubated with primary antibodies (BA-F8, SC-71, BF-35 and BF-F3) purchased from DSHB (USA) for 60 min at room temperature. Biotinylated antimouse IgG and IgM (Santa Cruz Biotechnology, Inc., USA) were applied for 30 min and avidin-biotin complex (Thermo Fisher Scientific Inc., USA) was used for immuneperoxidase staining. The sections were visualized by incubating with diaminobenzidine tetrahydrochloride (Sigma-Aldrich Co., USA) with 0.3% H2O2 for 3-5 min. The representative stained transverse sections are presented in Fig. 1. MHC-based fiber types were classified into three types as follows: type IIX, fibers which were detected with SC-71 (anti-MHC 2x and 2a); type IIA, fibers which were detected with SC-71 and BA-F8 (anti-MHC slow); type I, fibers which were detected with BA-F8. Approximately 600 fibers per muscles were counted and image analysis was conducted using Image-Pro® plus 5.1 (Media Cybernetics Inc., USA). Three traits of the muscle fibers were estimated. Muscle fiber density was defined as the fiber number distributed in 1 mm2 of muscle and muscle fiber size (μm2) was defined as the mean crosssectional area (CSA) of counted fibers. Muscle fiber area composition (%) was defined as the ratio of CSA of each fiber type to the total CSA.
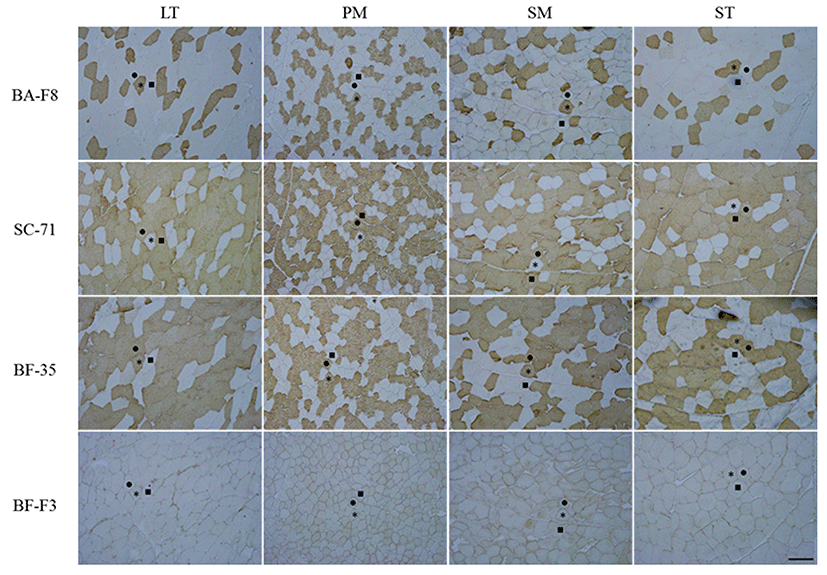
Muscle pH was measured using a pH-meter (MP230, Mettler-toledo, Switzerland) with 3.0 g muscle homogenates in 27 mL of deionized water. Moisture content (%) was determined according to the AOAC (2000) method. Fat content was determined by Folch et al. (1957) method with modifications. In brief, 5 g muscle was homogenized with 25 ml of solution A (chloroform:methanol, 2:1, v/v) and stirred for 2 h at 4℃. The homogenate was filtered with Whatman paper (No. 1) in a cylinder and the filtrate was separated into two layers with 0.88% NaCl. The cylinder well was washed with 10 ml of solution B (chloroform:methanol:H2O, 3:47:50) and the upper layer was removed. The lower layer was transferred to a glass dish and dried at 50℃. The remaining fat was wei- ghed and fat content was presented as a percentage (%) of the sample. Meat color was measured using the Minolta Chromameter CR-300 (Minolta Co., Japan) standardized with a white plate (Y=93.5, x=0.3132, y=0.3198) and presented as the Commission Internationale de l’Eclairage (CIE) L*, a*, and b* values. Drip loss (%) was determined using the Honikel (1987) method. For analysis of cooking loss, the muscle samples were packed with plastic bags and cooked in a water bath at 75℃ to an internal temperature of 70℃. Cooking loss was presented as a percentage of the initial weight. 1.3 cm diameter cores obtained from the cooked samples were cut vertical to the myofiber axis using an Instron (Model 4400, Instron Corp., USA) for Warner-Bratzer shear force (WBSF) (N). Sarcomere length was determined according to the Cross et al. (1981) method. In brief, muscle samples were placed in solution I (0.1 M KCl, 0.39 M boric acid, and 5 mM EDTA in 2.5% glutaraldehyde) for 2 h and solution I was replaced with solution II, consisting of 0.25 M KCl, 0.29 M boric acid, and 5 mM EDTA in 2.5% glutaraldehyde. After 18 h, the torn muscle fiber was placed horizontally in the path of a vertically oriented laser beam to obtain an array of diffraction bands on a screen. Sarcomere length (μm) was calculated by the following formula: ({632.8 × 103 × D × SQRT [(T/D)2 + 1]}/T) × 100, where D equals the distance (mm) from the specimen-holding device to the screen (D = 98 mm) and T equals the separation (mm) between zero and the first maximum band.
All the experimental data obtained from bovine skeletal muscles with experimental triplications are presented as means ± SE. An analysis of variance test and Duncan’s multiple range tests were performed to determine the differences in the muscle fiber characteristics and meat quality among the muscles using the Statistical Analysis Software (SAS, ver. 9.4, SAS Institute Inc., USA). Significant differences in muscle fiber characteristics and meat quality among the muscles were considered at the p<0.05 level. The relationships among the muscle fiber characteristics and between muscle fiber characteristics and meat quality were analyzed using Pearson’s correlation coefficients (r). r values with p<0.05 were accepted for determination of significant correlations between the two traits.
Results and Discussion
To confirm the distribution of MHCs at the protein level, LC-MS/MS analysis was performed and the result is presented in Table 1. Three isoforms, 2x, 2a, and slow, were identified with 6, 5, and 13 unique peptides and their significant ion scores (> 39), respectively. These isoforms had 34-37 matched peptides and 17-20% of sequence coverages. The previous reports support this finding of three MHC isoforms in bovine skeletal muscles except for rectus lateralis and retractor bulbi at the gene and protein levels (Baxa et al., 2010; Chikuni et al., 2004; Gonzalez et al., 2009; Kim, 2014; Maccatrozzo et al., 2004).
1)Accession numbers were taken from the NCBI database. 2)Theoretical molecular weight and pI. 3)Ion score >39 indicates identity (p<0.05). 4)Unique peptides specific to each MHC and commonly expressed in bovine LT, PM, SM and ST muscles.
As shown in Fig. 1, three MHC-based fiber types, such as type I, which is represented by BA-F8 (MHC slow) and BF-35 (MHCs slow and 2a), type IIA, which is represented by SC-71 (MHCs 2a and 2x) and BF-35, and type IIX, which is represented by SC-71, were classified in four bovine muscles by IHC and four monoclonal antibodies. BF-F3, which corresponds to MHC 2b, did not stain any muscles. The three fiber types observed in the present study are pure types (consisting of one isoform), whereas hybrid types (consisting of two or more isoforms) were not found in the four bovine skeletal muscles. In the previous studies conducted in porcine longissimus muscle, densities of 2.8-14.7 (number/mm2) and relative areas of 1.7-11.9% for the hybrid types (IIAX and IIXB) were observed (Kim et al., 2013; Kim et al., 2014). Greenwood et al. (2009) also investigated hybrid types (type I to type IIA intermediate and type IIA to type IIX intermediate) and found them to be approximately 7% of the composition in bovine longissimus lumborum (LL) muscle. It seems that the different results are caused by the differences in species (pig vs. cattle) and breed (Hanwoo vs. Belmont Red).
Representative characteristics such as fiber density, fiber area composition and CSA are presented in Fig. 2. When comparing fiber density within each muscle, LT, PM and SM had the highest density of type IIA (224.5 for LT, 495.1 for PM and 231.6 for SM) (p<0.05), whereas ST had higher density in type IIX (210.6) than in types I and IIA (p<0.05) (Fig. 2A). The fiber density of types I and IIX in PM and SM did not show significant differences (p>0.05). The remarkable finding is that all the fiber types of PM had the highest density compared to other muscles (p<0.05). This means that the muscle fiber size regardless of fiber types is smaller in PM than in other muscles. As expected, PM had the smallest CSA values for the three fiber types among bovine muscles (p<0.05) (Fig. 2B). The CSA for all types were similar between LT and ST (p>0.05). Significantly higher CSA was observed in type IIX than in types I and IIA in LT, PM and SM muscles (p<0.05), whereas SM muscle had no significant difference in CSA among the fiber types (p>0.05). Fiber area composition within muscles showed a similar trend to fiber density (Fig. 2C). Similar to the fiber density, LT, PM and SM muscles had the highest composition of type IIA fibers (58.9% for LT, 46.3% for PM, and 44.3% for SM), whereas ST muscle had a higher composition of type IIX fibers (62.3%), compared to the other fiber types (p<0.05). A comparison of the fiber compositions among the muscles revealed that the LT and ST had the highest compositions of types IIA and IIX fibers, respectively (p<0.05). However, the composition of type I fibers did not differ between PM and SM or between LT and ST (p>0.05). Fiber area composition in SM muscle showed a similar result to that reported by Gonzalez et al. (2009), who found that SM muscle had 27.5-27.9% of type I, regardless of treatments. However, LL muscle had higher type I composition (30.5-32.5%) than our results (12.4%). Greenwood et al. (2009) observed 25-28% of type I in LL muscle which is also higher than the results in the present study. According to Kirchofer et al. (2002), who investigated the muscle fiber characteristics of beef chuck and round muscles, red types (β- and α-Red) had a similar size (diameter) in SM and ST, however, the size of the white type (α-White) was higher in ST than in SM. In the present study, ST had larger type IIX than SM (p<0.05). In a previous report that compared fiber size between the ST and LT of 16 mon-old Montbéliard cattle, LT muscle had large fibers than ST regardless of fiber type. However, there was no significant difference in the CSA of the three fiber types between LT and SM (p>0.05). The differences in fiber size and composition between our result and the previous reports are due to differences in breed, age, diet and breeding environment (Herpin and Lefaucheur, 1992; Lefaucheur, 2010; Solomon and Lynch, 1988).
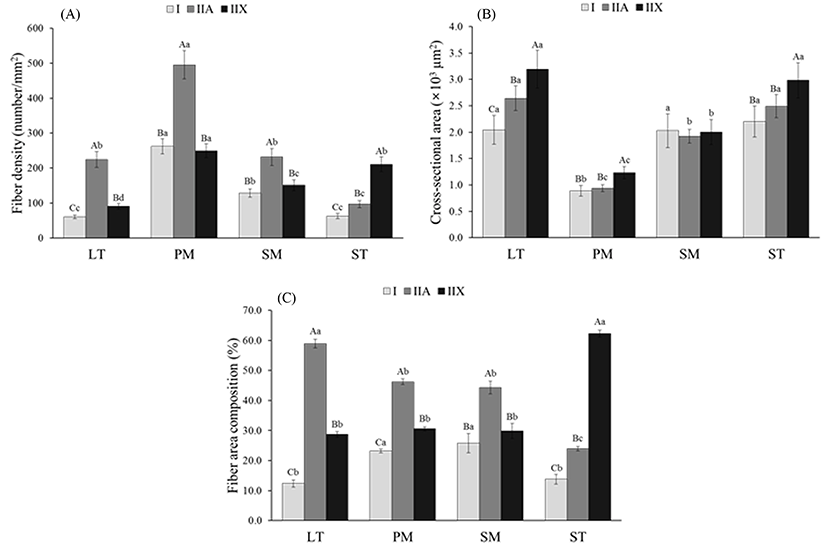
To estimate the relationships between muscle fiber density, CSA and fiber area composition, Pearson correlation coefficients were evaluated (Fig. 3). Fiber density negatively correlated with CSA regardless of fiber type (p<0.05), as expected. The fiber density of types I (r=0.48) and IIA (r=0.32) had positive correlations with the fiber area composition of type I (p<0.05), but negative correlations were observed with type IIX (r=-0.22 and p>0.05 for type I, r=-0.42 and p<0.05 for type IIA) in fiber area composition. The fiber density of type IIX was negatively correlated with the fiber area composition of type IIA (r=-0.36, p<0.05), whereas there was no significant correlation with the fiber area composition of type I (p>0.05). Negative correlations were observed between the fiber area composition of type I and the CSA of types IIA (r=-0.50, p<0.05) and IIX (r=-0.59, p<0.05), whereas the other traits did not significantly correlate with each other (p>0.05). Muscle fiber types I and IIA generally showed strongly negative correlation with type IIX. In other words, higher composition and large sizes for type IIX reduce the ratio of fiber types I or IIA. On the contrary, type IIA with higher density and small size increase the area composition of type I. Although type IIA (fast-twitch oxido-glycolytic) is generally classified as an intermediate type between type I (slow-twitch oxidative) and type IIX (fast-twitch glycolytic) (Acevedo and Ribero, 2006; Kim et al., 2014), type IIA showed a contrasting and strongly negative relationship with type IIX in the present study.
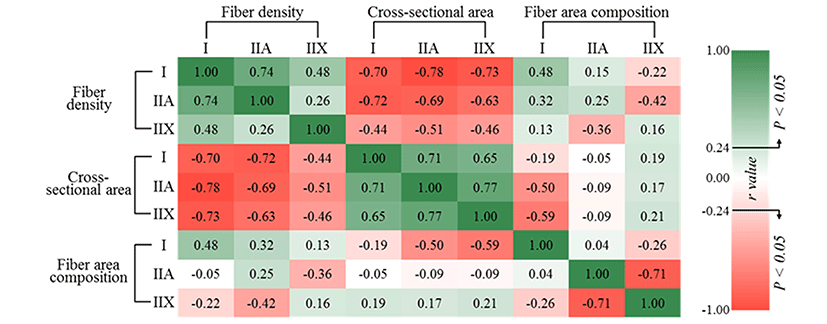
The results for the meat quality characteristics of bovine muscles are presented in Table 2. All the meat quality traits except for cooking loss showed significant differences among the muscles (p<0.05). The pH value of ST was the lowest (5.48) among the muscles (p<0.05), however the pH vales for LT (5.59) and SM (5.60) as well as for PM (5.55) and LT did not significantly differ (p>0.05). Moisture and fat contents showed opposite results for LT and ST muscles. LT had the lowest moisture content (67.08%) and the highest fat content (12.46%), whereas ST had the highest amount of moisture (73.01%) and the lowest amount of fat (7.27%) (p<0.05). Myoglobin content and all color values (CIE L*, a* and b*) were significantly higher in PM than in other muscles (p<0.05), however, CIE L* was not significantly different with ST (p>0.05). The lowest drip loss was observed in LT muscle, while the lowest WBSF and the highest sarcomere length were found in PM among the muscles (p<0.05). The ultimate pH value reflects the postmortem metabolic rate and consequently affects other quality traits such as meat color (especially lightness), water-holding capacity (WHC) and tenderness (Ryu and Kim, 2005). In the present study, ST, which had the lowest pH, showed low WHC (high drip loss) and tenderness (high WBSF and low sarcomere length), whereas PM had the reddest and tenderest meat among the muscles. McKenna et al. (2005), who compared discoloration characteristics between 19 bovine muscles, observed higher pH in LT (5.78), as well as redness (20.26) and myoglobin content in SM (4.90 mg/g) than in the other three muscles at 72 h postmortem. In another study (Rodriguez et al., 2014), PM was observed in much more tender muscle than LL, ST and gluteus medius of bulls and steers. Kołczak et al. (2003) reported that the sarcomere length of PM is shorter than that of ST 3 h after slaughter but longer 6 d later. The previous report support the finding that PM muscle is tenderer than LT and SM (Hwang et al., 2010). Although the different results from the previous reports are due to different breed, age and sex, most of the meat quality traits are clearly different among the muscles similar to the results for muscle fiber characteristics.
a-dMeans ± SE with different superscripts differ significantly at p<0.05 level.
1)LT, longissimus thoracis; PM, psoas major; SM, semimembranosus; ST, semitendinosus. 2)Warner-Bratzler shear force.
The clearly different characteristics of the muscle fibers in bovine muscles are basically a result of the differences in their physiological functions, and are consequently related to beef quality. As presented in Table 3, a lot of significant correlations were found between muscle fiber characteristics and meat quality traits. The pH, moisture and fat contents as well as CIE L* values were mainly correlated with the fiber density of type IIX and the area compositions of types IIA and IIX. pH and fat content had negative correlations with the fiber density and area composition of type IIX, whereas moisture content and CIE L* value showed positive correlations (p<0.05). The opposite trends were found between fiber density and CSA in myoglobin content, CIE a*, CIE b*, WBSF and sarcomere length regardless of fiber types. WBSF was positively correlated with CSA (p<0.05), whereas the others were negatively correlated with CSA (p<0.05). The fiber density of type IIX did not significantly correlate with CIE a*, CIE b* or WBSF (p>0.05). In fiber area composition, the contrary correlations were found between type IIA and IIX with all beef quality traits. Type IIX showed significant correlations in fiber area composition with all beef quality traits except for cooking loss (p<0.05).
1)Warner-Bratzler shear force. *p<0.05; **p<0.01; ***p<0.0001.
These results indicate that the muscle fiber composition of type IIX is closely related to poor beef quality, especially color (negatively correlated with myoglobin content and CIE a*), WHC (positively correlated with drip loss) and tenderness (positively correlation with WBSF and negatively correlated with sarcomere length). However, the fiber area composition of type IIA is closely related to high redness (CIE a*) and WHC (negatively correlated with drip loss). Muscles that consist of more small-sized fibers, such as PM, regardless of fiber type could be redder and tenderer. Ordway and Garry (2004) reported that myoglobin was expressed in the order of type I > IIA > IIX in skeletal muscle while Hwang et al. (2010) reported that the fiber size (diameter) is negatively correlated with myoglobin content regardless of fiber types. These observations support our findings regarding the myoglobin content and its relationship with muscle fiber density and CSA. Nevertheless, meat color did not follow myoglobin content with regard to muscle fiber characteristics. In other words, different relationships were observed for myoglobin and meat color with muscle fiber characteristics when estimating within the muscle and among the different muscles. In a previous study on bovine LT muscle (Ozawa et al., 2000), redness was positively correlated with the muscle fiber diameter of oxidative types (βR and αR). Tenderness traits such as WBSF and sarcomere length were closely related with the fiber size of type IIB (fast-twitch and glycolytic fiber) in previous reports (Karlsson et al., 1993; Renand et al., 2001). In the present report, the fiber size of type IIX (fast-twitch and glycolytic fiber) as well as types I and IIA were closely related with tenderness traits.
Conclusions
Three MHCs (2x, 2a and slow) were identified from bovine LT, PM, SM, and ST muscles and the muscle fibers were classified into three types (IIX, IIA, and I corresponded to MHC 2x, 2a and slow, respectively). Different muscle fiber characteristics were observed among the muscles and clear differences were also found in meat quality traits. Among the muscles, PM which consisted of small-sized fibers and in which fiber density was highest regardless of muscle fiber type was the most red and tender meat. However, ST muscle had the highest composition of type IIX and had poor quality (low pH, redness and sarcomere length and high drip loss). In conclusion, the differences in meat quality between muscles can be explained by the differences in muscle fiber characteristics, and especially, the muscles with good quality are composed of more small-sized fibers regardless of fiber type.