Introduction
Although approved nitrite levels are considered safe, many consumers want to ban its use (Jeong, 2016; Kang and Lee, 2003) because nitrite can be converted to nitrosamine, which has been identified as a potential carcinogen (Ahn et al., 2002; Bedale et al., 2016). For this reason, synthetic nitrite alternatives, derived from natural sources thought to be healthier, are being developed for use in processed meat products. Synthetic nitrite is used in the manufacturing of meat products as a curing agent (Honikel, 2008; Sebranek and Bacus, 2007). During curing, nitrite is added to enhance the color and flavor of the meat and to extend its shelf life because it inhibits the growth of many pathogenic microorganisms and imparts antioxidant activity (Honikel, 2008). The consumer preference for natural foods has a strong influence on the meat processing industry's practices (Lorenzo et al., 2014), because these natural food products are healthier, and thus, consumer demand for these natural products has resulted in substantial increases in their production (Bedale et al., 2016; Choi et al., 2016).
One of the methods that is being developed to avoid the direct addition of synthetic nitrite to processed meat products involves the use of pre-converted nitrite, derived from natural sources, by a starter culture (Wojciak et al., 2014). Djeri and Williams (2014) showed that pre-converted nitrite from celery powder had properties that are similar to those of synthetic nitrite. However, celery powder contains several allergens, which raises additional concerns (Ballmer-Weber et al., 2002). Therefore, the meat processing industry is actively searching for novel, natural compounds that can improve meat color, flavor, and stability.
Many vegetables contain high levels of natural nitrate and nitrite (Chang et al., 2013; Choi et al., 2015). This is particularly true for red beets, which have been reported to possess nitrate levels up to 3,288 ppm (Djeri and Williams, 2014; Walker, 1990). Djeri and Williams (2014) found that celery powder contains nitrates that are utilized in lactic acid starter cultures in the production of natural turkey bologna. Krause et al. (2011) showed that pre-converted nitrite from incubated vegetable sources can effectively maintain the pink color of meat products. Furthermore, Kim et al. (2017) confirmed that fermented vegetable extracts were effective substitutes to synthetic nitrite for color development in cured meats. All of these studies showed that fermented vegetable extracts contain preconverted nitrite that could be used to replace synthetic nitrite and to maintain color development in cured meats.
Ascorbic acid is also used to extend the shelf life of meat products. It acts synergistically when used in combination with other antioxidants and promotes their antioxidant activity (Hwang et al., 2013). Grobbel et al. (2006) reported that ascorbic acid is “generally recognized as safe (GRAS)” and can be applied to the surface of meat products to inhibit discoloration.
In this study, we investigated the synergistic effects of pre-converted nitrite from fermented red beet extract and ascorbic acid on quality characteristics using several meat emulsion treatments in order to evaluate the combinatorial benefits of pre-converted nitrite from red beet extract and ascorbic acid on color development, residual nitrite content, and the physicochemical properties of meat emulsions.
Materials and Methods
Commercial red beets, purchased from a local market, were freeze-dried and crushed into a powder. Ten grams of powder were mixed with 100 mL of distilled water for 30 min, then 0.025% of an active nitrate reductase culture containing Staphylococcus carnosus (S-B-61 BactofermTM, Chr. Hansen Inc., USA) was added, and the mixture was incubated with shaking at 30°C for 24 h. The mixture was then filtered using Whatman No. 1 filter paper, and evaporated in a rotary evaporator (EYELA N-1000, Rikakikai, Japan) at < 50°C. The concentrated samples were stored in amber flasks in the dark at 4°C and used within 24 h of preparation (pre-converted nitrite sources from red beet: pH = 4.65, L*-value = 10.13, a*-value = 8.24, b*-value = 13.52, nitrite content = 729.28 ppm). The nitrite contents in fermented red beet extract was measured using diazo coupling method (KFDA, 2013).
Fresh pork ham and back fat from castrated boars (Landrace × Yorkshire × Duroc; M. biceps femoris, M. semitendinosus, M. semimembranosus), approximately 110 kg in weight, was purchased from a local processor approximately 48 h postmortem. All subcutaneous and intramuscular fat and visible connective tissue was removed from the muscle, and the lean material and back fat was ground using an 8-mm plate. The ground tissue was placed in polyethylene bags, vacuum-sealed, and stored at 0°C. The compositions of the control and treated samples are shown in Table 1. The sample without added nitrite (synthetic or red beet extract) was used as a control, and the samples treated with added synthetic nitrite were used as T1 and the samples treated with added synthetic nitrite and 0.05% ascorbic acid were used as T2. The remaining four treatments consisted of meat emulsion samples treated with pre-converted nitrite from the fermented red beet extract and ascorbic acid as follows: 5% pre-converted nitrite source from red beet (T3), 5% pre-converted nitrite source from red beet and 0.05% ascorbic acid (T4), 10% preconverted nitrite source from red beet (T5), 10% pre-converted nitrite source from red beet and 0.05% ascorbic acid (T6). The lean tissue was homogenized and ground for 1.5 min in a silent cutter (Nr-963009, Hermann Scharfen GmbH & Co., Germany). The back fat, NaCl, and 0.15% sodium tripolyphosphate was added, and mixed in for 1.5 min. The meat batter was then homogenized for 3 min. A temperature probe (KM330, Kane-May, UK) was used to monitor the temperature of the batter to ensure the temperature remained below 10°C throughout batter preparation. Following batter preparation, the meat batter was stuffed into collagen casings with a diameter of 25 mm (#240, NIPPI Inc., Japan) using a stuffer (IS-8, Sirman, Italy). The meat batter was heated at 80 °C for 60 min in a chamber (MAXi3501, Kerres, Germany), and cooled at 21°C for 3 h. This procedure was performed in triplicate for each meat emulsion treatment (Choi et al., 2009).
The pH values of the various treated meat emulsion samples were measured in a homogenizer (UItra-Turrax T25, Janke & Kunkel, Germany) using 5 g of each sample and 20 mL distilled water with a pH meter (Model 340, Mettler-Toledo GmbH, Switzerland).
The color characteristics of the treated meat emulsions were evaluated using a colorimeter (Chroma meter CR-210, Minolta, Japan) with an 8-mm diameter measuring area and a 50-mm diameter illumination area. The color characteristics are expressed as L* (100 = white, 0 = black), a* (positive = redness, negative = greenness), and b* (positive = yellowness, negative = blueness). Color measurements were taken from ten random areas of each emulsion treatment. Hue angle (H°), color difference (ΔE*), and chroma difference (ΔC*) were calculated using the following equations: H° = arctan (b*/a*), ΔE* = (ΔL*2+Δa*2+Δb*2) 1/2, and ΔC* = (Δa*2+Δb*2)1/2 as described by Grigelmo-Miguel et al. (1999) in accordance with the American Meat Science Association guidelines for color measurements (Hunt et al., 1991).
Volatile basic nitrogen (mg%) was measured using a modified micro diffusion assay as described by Pearson (1968).
Where, a = titer for sample, b = titer for blank, f = factor of reagent, N = normality, S = sample weight (g)
Lipid oxidation was assessed using the TBARS method (Tarladgis et al., 1960), with minor modifications and is expressed in mg of malondialdehyde (MD) per kg sausage. Ten grams of the sample was blended using a homogenizer (AM-7, Nihonseiki Kaisha Ltd., Japan) with 50 mL distilled water for 2 min and transferred to a distillation tube. The cup used for blending was washed with 47.5 mL of distilled water and added to the distillation flask with 2.5 mL of 4 N HCl and a few drops of antifoaming agent (KMK-73, Shin-Etsu Silicone Co. Ltd., Korea). The mixture was distilled, and 50 mL of the distillate was collected. Five milliliters of 0.02 M TBA in 90% acetic acid was added to each test tube containing 5 mL of the distillate and mixed. The tubes were capped and heated in a boiling water bath for 30 min to develop the chromogen and cooled to room temperature. Absorbance was measured at 538 nm using a blank prepared with 5 mL distilled water and 5 mL TBA reagent in an UV/VIS spectrophotometer (Optizen 2120 UV plus, Mecasys Co. Ltd., Korea).
To determine the total viable count (TVC), and E. coli, and coliform bacteria in each sample, 25 g of each sample was aseptically transferred to a sterile stomacher bag containing 225 mL of 0.1% peptone water, and the samples were pummeled using a stomacher (Masticater-Paddle-Blender, IUL Instrument, Spain) for 3 min. The homogenates were then serially diluted with 0.1% peptone water. The serially diluted samples (1 mL) were placed in Petri dishes, and 20 mL plate count agar (PCA; Difco Sparks, USA) was poured over the serially diluted samples. Following solidification, the plates were incubated at 37°C for 48 h and colonies were counted manually.
A trained 12-member panel consisting of researchers from the Food Processing Research Center of Korea Food Research Institute in the Republic of Korea evaluated each treatment. Each sample was evaluated for color, flavor, off-odor, juiciness, and overall acceptability using the Hedonic test (Choi et al., 2008). The samples were heated at 80°C for 60 min in a chamber (MAXi3501, Kerres, Germany) and cooled at 21°C for 3 h. Panelists were instructed to cleanse their palates between samples with water. The color, flavor, off-odor, juiciness, and overall acceptability of the cooked samples were evaluated using a 9-point descriptive scale (1 = extremely undesirable, 9 = extremely desirable).
The residual nitrite content was determined according to the AOAC guidelines (1990), and is expressed as ppm per kg of meat emulsion. All samples were analyzed at the same time to minimize variation. Briefly, 10 mL 0.5 N NaOH and 10 mL 12% (NH4)2SO4 solution was added to each sample, mixed with 150 mL distilled water, and heated in a boiling water bath to 80°C. After cooling, 20 mL CH3COONH4 buffer (pH 9.00) and 10 mL distilled water were added to the samples. After 10 min, the residual nitrite content was measured using the diazo coupling method. The residual nitrite content was calculated using a standard curve (KFDA, 2013).
Each experiment was completely randomized with three independent replications. pH, color, TBARS, VBN, TVC, E. coli and coliform bacterial counts, sensory properties, and residual nitrite content was examined using one way analysis of variance (ANOVA). Differences between the means were compared using Duncan's Multiple Range test. A significance level of p<0.05 was set for all evaluations. The data were analyzed using SAS 9.4 software (SAS Institute Inc., USA).
Results and Discussion
Table 2 shows the effects of various concentrations of fermented red beet extract and ascorbic acid on pH and color in the raw meat emulsions. The measured pH values ranged from 6.25 in the T6 treatment containing 10% fermented red beet extract and 0.05% ascorbic acid, to 6.41 in the control treatment. The pH values of the raw meat emulsions containing fermented red beet extract were lower to that of the control and the sample treated with synthetic nitrite (p<0.05); furthermore, they had significantly higher pH values than the samples containing both fermented red beet extract and ascorbic acid (p<0.05). The pH values of the raw meat emulsions containing fermented red beet extract were lower than those of the raw meat emulsions with synthetic nitrite, with or without ascorbic acid (p<0.05). Our results are similar to those of Kim et al. (2017), who showed that treatments with the different concentration of fermented spinach extract yielded different pH values. They postulated that this may be explained by the low pH of fermented extract. Sindelar et al. (2007) also found similar trend of pH values in their treatments, suggesting that lactic acid bacteria was associated with pH trend.
In addition to pH, Table 2 depicts the color characteristics of the raw meat emulsion treatments formulated with fermented red beet extract and ascorbic acid. The lightness of the control, containing no nitrite or fermented red beet extract, was the lowest of all of the treatments (p<0.05). The redness of the control was significantly higher than that of any of the treatments (p<0.05), because oxymyoglobin has brighter color than NO-myoglobin (Honikel, 2008). The redness and yellowness of the treated samples increased with increasing concentrations of fermented red beet extract. Redness also increased with the addition of ascorbic acid due to acceleration effect of ascorbic acid to form NO-myoglobin. The hue angle index (H°) values of the treated raw meat emulsions were significantly higher than the control (p<0.05), indicating they have a browner hue (Hunt et al., 1999). The measured color difference (∆E*) was the lowest for T6 (p<0.05); however, there were no significant differences among the other treatments as compared to the control (p>0.05). The chroma difference (∆C*) between each of the treated raw meat emulsions and the control was not significant (p>0.05). Our results show that color development in the raw meat emulsions tested is similar in both the synthetic nitrite and fermented red beet extract treatments.
All values are mean±standard deviation of three replicates (n=9).
a-fMeans within a column with different letters are significantly different (p<0.05).
1)Control, meat emulsion with nitrite free; T1, meat emulsion with 0.015% nitrite; T2, meat emulsion with 0.015% nitrite and 0.05% ascorbic acid; T3, meat emulsion with 5% fermented red beet extract; T4, meat emulsion with 5% fermented red beet extract and 0.05% ascorbic acid; T5, meat emulsion with 10% fermented red beet extract; T6, meat emulsion with 10% fermented red beet extract and 0.05% ascorbic acid. 2) H°, arctan (b*/a*). 3)∆E*, (∆L*2+∆a*2+∆b*2)1/2. 4)∆C*, (∆a*2+∆b*2)1/2.
The pH values and color characteristics of the cooked meat emulsions containing fermented red beet extract and ascorbic acid are shown in Table 3. Similar to the uncooked samples, the pH of the cooked meat emulsions containing fermented red beet extract decreased as the concentration of fermented red beet extract increased. In addition, the pH was significantly affected by the addition of ascorbic acid (p<0.05). Furthermore, the pH values of the cooked meat emulsions displayed a similar trend to those of the raw meat emulsions.
All values are mean±standard deviation of three replicates (n=9).
a-gMeans within a column with different letters are significantly different (p<0.05).
1)Control, meat emulsion with nitrite free; T1, meat emulsion with 0.015% nitrite; T2, meat emulsion with 0.015% nitrite and 0.05% ascorbic acid; T3, meat emulsion with 5% fermented red beet extract; T4, meat emulsion with 5% fermented red beet extract and 0.05% ascorbic acid; T5, meat emulsion with 10% fermented red beet extract; T6, meat emulsion with 10% fermented red beet extract and 0.05% ascorbic acid. 2)H°, arctan (b*/a*). 3)∆E*, (∆L*2+∆a*2+∆b*2)1/2. 4)∆C*, (∆a*2+∆b*2)1/2.
The measured lightness of the T1 treatment, containing 0.015% nitrite, was the highest (p<0.05), and the lightness of the cooked meat emulsions containing ascorbic acid were lower than those without ascorbic acid and the redness of all of the treated samples was higher than that of the control (p<0.05) due to dissociation of oxymyoglobin that changed to grey color and development of nitrsohemochrome that has bright reddish color (Honikel, 2008). The redness of the samples treated with fermented red beet extract alone were lower than those of the samples treated with both fermented red beet extract and ascorbic acid (p<0.05). Ascorbic acid can accelerate a denaturation of nitrite to nitric oxide that can be able to form NO-myoglobin (Honikel, 2008). The yellowness values of the samples containing nitrite were lower than those without nitrite (p<0.05). The hue angle index (H°) was highest in the control (p<0.05), and the hue angle index values of the samples treated with fermented red beet extract alone were higher than those of the samples treated with both fermented red beet extract and ascorbic acid (p<0.05). The color (∆E*) and chroma (∆C*) differences of the samples treated with fermented red beet extract were lower than that of the samples treated with both fermented red beet extract and ascorbic acid (p<0.05). Therefore, the fermented red beet extract and ascorbic acid can replace synthetic nitrite for color development of the cooked meat emulsions.
The results of our VBN and TBARS analyses of the meat emulsions containing fermented red beet extract and ascorbic acid are shown in Table 4. The highest VBN levels were found in the control, and the VBN values of the samples treated with fermented red beet extract alone were higher than that of the samples treated with combined fermented red beet and ascorbic acid (p<0.05). VBN levels are affected by amino acid decarboxylase activity, as well as the activity of other enzymes and microorganisms (Kohsaka, 1975). Nitrite decreases the VBN levels of meat products as a result of its ability to inhibit enzymatic activity and the growth of microbes (Jay, 1992; Weiss et al., 2010) and the lower VBN values measured may be the result of the inhibition of microbial growth by nitrite. Inevitably, lower total viable count had lower VBN values and treated samples with ascorbic acid had lower VBN values than samples without ascorbic acid because ascorbic acid and nitrite created a synergetic antioxidant activity (Hwang et al., 2013).
Lipid oxidation in meat leads to rancidity and alters its nutritive value, color, and flavor (Choi et al., 2011). Our TBARS analysis results are also shown in Table 4. The highest TBARS levels were found in the control (p<0.05), while the TBARS values of the samples treated with both fermented red beet extract and ascorbic acid were lower than those of the samples without ascorbic acid. Sindelar et al. (2007) reported similar results that lipid oxidation were significantly different between treatment with fermented vegetable powder and control with nitrite. TBARS values of treatment were higher than those of control due to the antioxidant effects of nitrite. Krause et al. (2011) reported that treating meat with fermented vegetable powder containing sodium nitrite reduces lipid oxidation. A 0.5-1.0 mg MD/kg TBARS value is considered the lower threshold for observable oxidized odor (Tarladgis et al., 1960). All of the TBARS values measured in this study were less than 0.5 mg MD/kg. Therefore, the natural nitrite derived from fermented red beet extract effectively inhibits the production of malondialdehyde (MD), similar to synthetic nitrite, in the meat emulsions examined.
All values are mean±standard deviation of three replicates (n=9).
a-dMeans within a column with different letters are significantly different (p<0.05).
1)Control, meat emulsion with nitrite free; T1, meat emulsion with 0.015% nitrite; T2, meat emulsion with 0.015% nitrite and 0.05% ascorbic acid; T3, meat emulsion with 5% fermented red beet extract; T4, meat emulsion with 5% fermented red beet extract and 0.05% ascorbic acid; T5, meat emulsion with 10% fermented red beet extract; T6, meat emulsion with 10% fermented red beet extract and 0.05% ascorbic acid.
The TVC and number of E. coli and coliform bacteria in the meat emulsion treatments are shown in Table 5. TVC was highest in the control, and lowest in treatment T2, which contained synthetic nitrite and ascorbic acid (p<0.05). In all samples containing ascorbic acid, the TVC was lower than in the samples without ascorbic acid. E. coli and coliform bacteria were not detected in any of the treatments. Djeri and Williams (2014) reported similar results, suggesting that anaerobic bacterial counts were lower in meat products treated with celery juice powder and cherry juice powder, likely as a result of their sodium nitrite content. Furthermore, Jackson et al. (2011) found that nitrite significantly decreased microbial growth in inoculated frankfurters cured with natural pre-converted nitrite. Sebranek et al. (2012) found that meat products manufactured with incubated celery powder, which contains nitrite, had antimicrobial properties. Our results show that treating meat with fermented red beet extract and ascorbic acid lowers TVC, and might have the potential to extend shelf-life.
All values are mean±standard deviation of three replicates (n=9).
a-eMeans within a column with different letters are significantly different (p<0.05).
1)Control, meat emulsion with nitrite free; T1, meat emulsion with 0.015% nitrite; T2, meat emulsion with 0.015% nitrite and 0.05% ascorbic acid; T3, meat emulsion with 5% fermented red beet extract; T4, meat emulsion with 5% fermented red beet extract and 0.05% ascorbic acid; T5, meat emulsion with 10% fermented red beet extract; T6, meat emulsion with 10% fermented red beet extract and 0.05% ascorbic acid.
Our sensory evaluation of the meat emulsions containing fermented red beet extract and ascorbic acid are shown in Table 6. Treatment T2, with both nitrite and ascorbic acid, had the highest color score (p<0.05), and the color scores of each of the samples containing ascorbic acid were higher than in those without ascorbic acid (p<0.05) and the higher the concentration of fermented red beet was, the higher the score for color was (p<0.05). Sindelar et al. (2007) found that their control, containing nitrite, scored significantly higher for color than the treatments with vegetable juice powder, and that visual cured color was influenced by incubation time and vegetable juice powder concentration. The flavor scores ranged 6.43 to 7.57. The lowest flavor and off-odor scores were found in the control (p<0.05). Juiciness was not significantly different for samples treated with fermented red beet extract and ascorbic acid (p>0.05). Although control had similar score for color with samples treated fermented red beet extract without ascorbic acid (p>0.05), T5 had higher score for overall acceptability than control (p<0.05) and T2 had the highest overall acceptability score, but was not significantly different from treatment T6 (p>0.05). Djeri and Williams (2014) reported similar results, suggesting that the overall acceptability score for bologna made with celery juice powder was not significantly different from that of bologna made with nitrite. Terns et al. (2011) found that the overall acceptability for cured emulsified cooked sausage manufactured with cherry powder using a starter culture was not significantly different from that of their control. In this study, samples with 10% fermented red beet extract had similar score for overall acceptability with samples with nitrite.
All values are mean±standard deviation of three replicates (n=9).
a-dMeans within a column with different letters are significantly different (p<0.05).
1)Control, meat emulsion with nitrite free; T1, meat emulsion with 0.015% nitrite; T2, meat emulsion with 0.015% nitrite and 0.05% ascorbic acid; T3, meat emulsion with 5% fermented red beet extract; T4, meat emulsion with 5% fermented red beet extract and 0.05% ascorbic acid; T5, meat emulsion with 10% fermented red beet extract; T6, meat emulsion with 10% fermented red beet extract and 0.05% ascorbic acid.
Fig. 1 shows the residual nitrite content of the meat emulsions containing fermented red beet extract and ascorbic acid. The lowest residual nitrite content was found in the control (p<0.05). The residual nitrite content of the samples treated with ascorbic acid was lower than that of the samples without ascorbic acid (p<0.05). Hayes et al. (2013) showed an increase in the rate of residual nitrite depletion following the addition of ascorbic acid. Meat emulsion with 5% fermented red beet extract had lower residual nitrite content than meat emulsion with 10% fermented red beet extract (p<0.05). This results agree with the findings of Kim et al. (2017), who reported that the residual nitrite content of cured pork loin increased as the concentration of fermented spinach extract increased, indicating that residual nitrite content was likely influenced by the presence of pre-converted nitrite in the fermented spinach extract. Sindelar et al. (2007) reported that the residual nitrite content of meat products manufactured with vegetable juice powder increased as the amount of pre-converted nitrite added to the meat product increased. Terns et al. (2011) observed an increase in residual nitrite in meat products made with cherry powder and a starter culture. These results indicate that the combination of fermented red beet extract and ascorbic acid has a large impact on residual nitrite content.
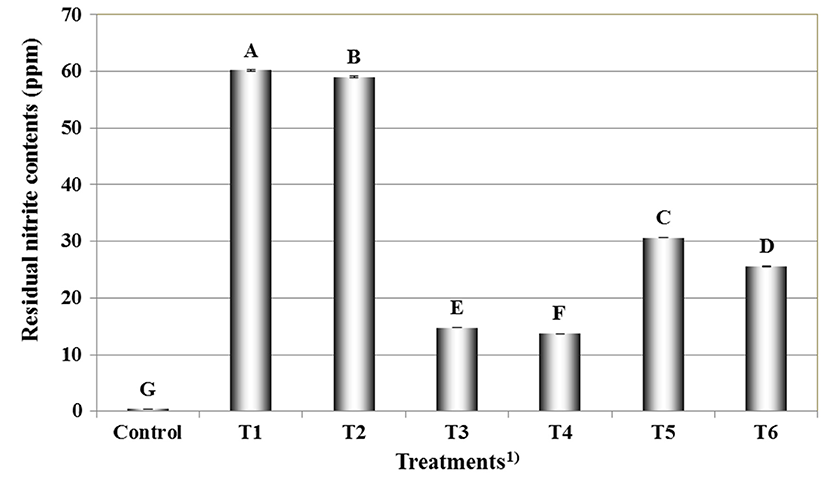
Conclusion
In this study, we have shown that the combination of fermented red beet extract and ascorbic acid has significant potential for use in the manufacture of meat products from natural pre-converted nitrite and ascorbic acid sources. Our results show that these natural sources can replace synthetic nitrite while maintaining color development in meat emulsions.