Introduction
Clean labels require no synthetic additives, minimal processing, a concise list of raw materials, easy-to-understand selection of raw materials, and the use of traditional processing methods (Asioli et al., 2017). Clean labeling of food products was first started in the UK in the 1990s (Yong et al., 2020). Clean label foods have been preferred by consumers because the ingredients in the product are clearly indicated on the packaging of the product (Lee, 2015; Aschemann-Witzel et al., 2019). In particular, clean labeling of meat products, which typically contain many food additives and are prepared using complicated manufacturing methods, is a major concern. Accordingly, food manufacturers have started to evaluate the use of ecofriendly, natural additives rather than synthetic chemicals (Ryu and Lee, 2018).
Despite many recent advancements in the meat processing industry, consumers continue to change preferences owing to health issues. In 2015, the International Agency for Research on Cancer under the World Health Organization announced that processed meat products and red meat are associated with carcinogenicity (Hur et al., 2015). Since then, researchers have focused on the development of healthy meat products. In particular, the use of nitrite, a synthetic additive commonly used in meat products, has been scrutinized, and food manufacturing has shifted focus to the development of processed meat products that are not harmful to health by eliminating food additives or replacing them with natural materials (Kim et al., 2019). Synthetic additive-free approaches have become important in the meat processing industry and are also related to clean labeling (Câmara et al., 2020). Because of the risks associated with additives used in the generation of some meat products, consumers have become skeptical of all types of food additives, and the food industry is therefore aiming to develop alternative technologies.
Accordingly, in this report, we discuss the use of pre-converted nitrite as a natural curing agent from a clean label perspective in processed meat products.
The Role of Nitrite in Meat Products
For years, nitrite has been used in the food and pharmaceutical industries because of its biological and functional roles. In particular, nitrite is widely used as an essential additive in meat products and has been applied for improving microbial safety, developing cured color and flavor, and inhibiting oxidation in meat products (Parthasarathy and Bryan, 2012). In this section, we discuss the roles and chemical reactions of nitrite in meat products.
Nitrite is commonly used in meat products because it inhibits microbial growth, including the growth of food-borne pathogens. When pork meat supplemented with sodium nitrite (150 mg/kg) was stored for 9 d at 4°C, the numbers of total aerobic bacteria, Enterobacteriaceae, Salmonella enterica, and Listeria monocytogenes were more than 1 Log CFU/g lower than those of the control group (Lamas et al., 2016). Nitrite can also inhibit anaerobic bacteria, particularly the neurotoxigenic species Clostridium botulinum. C. botulinum is a spore-forming bacterium, and some of its spores can survive at mild heating temperatures or pressures above 1,500 MPa (Majou and Christieans, 2018). According to Lebrun et al. (2020), sodium nitrite (≥ 30 mg/kg) can prevent the outgrowth and toxinogenesis of C. botulinum in cooked ham. In this regard, various studies have been conducted to elucidate the mechanisms mediating the bactericidal effects of nitrite, which could be explained by the reactions of nitric oxide (NO) and peroxinitrite.
When nitrite (NO2−, pKa=3.3) is added to a meat product having a pH of 5.5–6.5, it is progressively reduced to nitrous acid (HNO2) and NO, as describe as Eqs. (1–3). In this process, ascorbic acid, which is generally added to meat products with nitrite, accelerates the formation of NO via the reaction shown in Eq. (4) (Honikel, 2008). Another pathway involved in the production of NO in meat involves the activity of deoxy-myoglobin (MbFe2+), which exhibits nitrite reductase activity, as shown in Eq. (5) (Majou and Christieans, 2018).
The NO produced by the above processes easily reacts with iron (Fe) or the SH-group in amino acids (Honikel, 2008). Thus, NO can inhibit microbial growth by reacting with iron-sulfur proteins and forming iron-NO complexes (Majou and Christieans, 2018). Tompkin (1978) explained that when nitrite is added to canned ham, it reacts with ferredoxin (Fig. 1a), which is an iron-sulfur enzyme necessary for energy production in clostridial vegetative cells. Subsequently, modification of ferredoxin by NO results in inhibition of Clostridium outgrowth. Ren et al. (2008) suggested that NO induces bacteriostasis in Escherichia coli because it reacts with iron dihydroxyacid dehydratase, which is an iron-sulfur protein.
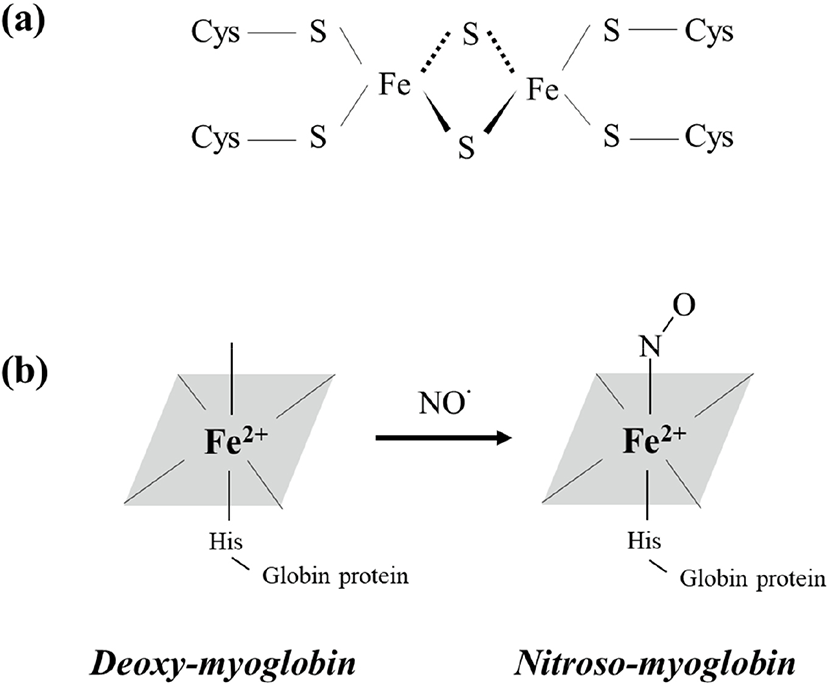
When meat products are progressively oxidized, superoxide radical anion (O2−) and hydrogen peroxide (H2O2) can be produced; these compounds can react with NO and nitrite, respectively, and then produce peroxynitrite (ONOO−), as shown in Eqs. (6–7) (Jo et al., 2020). Peroxynitrite is a strong oxidant and bactericidal compound that causes oxidative stress, denaturation of the cell wall, and DNA damage (An et al., 2019). For these reasons, nitrite addition improves the microbial safety of meat products.
The color of cured meat products is also an important factor that affects consumers’ purchase decisions (Flores and Toldrá, 2020). Generally, cured meat products exhibit a heat-stable pink color owing to changes in the form of myoglobin. Myoglobin, the main factor associated with meat color, is composed of globin protein and a heme-group containing a centrally located iron atom (Jo et al., 2020). Because NO is produced via nitrite addition to meat (Eqs. 1–5) and can react with iron, MbFe2+ (purple-red color) can bind to NO and be converted to nitroso-myoglobin (NO-MbFe2+, dark red), as described in Eq. (8) and Fig. 1b (Yong et al., 2019). Additionally, NO can also bind met-myoglobin (MbFe3+, brown color) and form nitroso-myoglobin through the action of reductases, such as ascorbic acid, as shown in Eq. (9) (Sebrane and Bacus, 2007). Subsequently, when the cured meat undergoes cooking, nitroso-myoglobin is denatured and converted to bright pink nitrosohemochrome. The pink color of cured meat products is caused by the presence of nitrosohemochrome (Parthasarathy and Bryan, 2012).
Another remarkable property of nitrite is its ability to inhibit lipid oxidation of meat products during storage or heating (Flores and Toldrá, 2020). Pork jerky made with sodium nitrite (70 mg/kg) shows low lipid oxidation compared with that without sodium nitrite (Yong et al., 2019). Additionally, NO converted from nitrite can act as a metal ion chelator. Indeed, NO binds to and stabilizes heme-iron and reduces the amount of free iron released from myoglobin. Because these irons are the major pro-oxidants in meat products, NO can block lipid oxidation (Parthasarathy and Bryan, 2012). Furthermore, NO can react with free radicals to terminate the lipid oxidation reaction (Jo et al., 2020). Because of its antioxidant effects, nitrite can suppress warmed-over flavor, an unpleasant rancid flavor detected in meats. Thomas et al. (2013) reported that the addition of nitrite inhibits the production of aldehyde by fatty acid oxidation production. However, the antioxidant effects of nitrite alone are insufficient for explaining the characteristic flavor of cured meats, and more complex reactions are involved in determining the flavor of meat products (Jo et al., 2020).
The Need for Natural Alternatives to Synthetic Nitrite
In the food industry, synthetic nitrites, such as sodium nitrite (NaNO2) and potassium nitrite (KNO2), are often used because they are inexpensive, stable, uniform, and easy to prepare. However, consumers’ perceptions that organic foods are healthier and more nutritious than inorganic foods, resulting in major concerns regarding the exposure of consumers to synthetic nitrite (Jo et al., 2020). Despite the fact that there really are no differences between organic food and inorganic food, consumers are willing to pay 10%–40% more for organic and natural foods (Sebranek and Bacus, 2007). Thus, the clean label food market stresses the use of natural additives instead of synthetic additives (Asioli et al. 2017).
In accordance with consumer demand, several studies have been conducted to identify substitutes for synthetic nitrite. Some studies have focused on the possibility of substituting synthetic nitrite with bacteriocins, organic acids, essential oils, and plant extracts showing strong antimicrobial activity (Flores and Toldrá, 2020). However, nitrite itself is difficult to replace using simple antioxidants or antimicrobial substances because it can serve multiple functions simultaneously. Accordingly, food manufacturers have explored the use of nitrite (NO2−) converted from nitrate (NO3−) from natural plants as a substitute for synthetic nitrite (Gassara et al., 2016). Using nitrate as a natural substance for meat products is not a new concept; centuries ago, meat curing processes used natural nitrate in the form of saltpeter (KNO3) (Honikel, 2008). However, modern technology has significantly improved, and various starter cultures are now used to reduce nitrate to nitrite with shorter incubation times. In addition, natural nitrite additives are being developed that do not affect the quality of the product, such as flavor (Sebranek et al., 2012).
Natural Nitrate Sources Used in Meat Product
Nitrate naturally occurs in soils, the atmosphere, plants, and waste waters. Among these natural nitrate sources, some plants contain considerable amounts of nitrates and are easy to use in meat products (Gassara et al., 2016). The general nitrate concentrations in plants are shown in Table 1. Several plants, including celery, spinach, radishes, and lettuce contain more than 2,500 mg nitrate/kg (Gassara et al., 2016; Schullehner et al., 2018). Among these plants, celery is the most extensively studied plant and has been used commercially because it does not significantly affect the sensory properties of meat products (Sebranek et al., 2012). Table 2 shows studies in which meat products were produced using various nitrate sources, including celery powder. Most of the studies used both nitrate-containing plants and a starter culture (nitrate-reducing bacteria) to reduce nitrate to nitrite. Sindelar et al. (2007) added celery juice powder and starter culture in emulsified sausages and incubated the sample. As a result, sausages made with celery juice powder showed similar quality to those made with sodium nitrite. Moreover, Kim et al. (2017) reported that when Swiss chard was fermented with starter culture and used in cooked loin ham, there were positive effects on color (particulary redness) and lipid oxidation. In cooked sausages, the addition of celery powder (0.8%) effectively inhibits quality deterioration during storage (Jin et al., 2018). Choi et al. (2017) showed that a meat-emulsion containing pre-converted nitrite from red beets (10%) with ascorbic acid had similar quality compared with that prepared using sodium nitrite. Importantly, natural nitrite sources are effective for controlling microbial growth and quality in meat products. According to Golden et al. (2014), pre-converted celery powder (nitrite content: 80 mg/kg) inhibits the growth of L. monocytogenes in deli-style turkeys.
Meat product | Pre-converted nitrite sources (added concentration) | Strain in starter culture | Reference |
---|---|---|---|
Ham | |||
Ground, cooked and sliced ham | Celery powder (1%) | Staphylococcus carnosus | Krause et al. (2011) |
Ready to eat uncured ham | Celery powder (1%, 2%) | S. carnosus | Sindelar et al. (2007) |
Ham | Celery juice powder (0.2%) and vinegar, lemon powder, and cherry powder blend (0.45%) | S. carnosus | Jackson et al. (2011) |
Sausage | |||
Cooked sausage | Cabbage (250 g/kg), chinese cabbage (250 g/kg), young radish (250 g/kg) | S. carnosus and S. xylosus | Ko et al. (2017) |
Cold smoked sausage | Celery (2.58%) | S. xylosus and Pediococcus pentosaceus | Eisinaitė et al. (2020) |
Dried sausage/Chorizo | Citric acid (200 ppm), acerola (100 ppm), rosemary (200 ppm), lettuce (3,000 ppm), arugula (1,500 ppm), watercress (1,500 ppm), spinach (3,000 ppm), celery (3,000 ppm), chard (3,000 ppm), and beet (3,000 ppm) | Pediococcus, S. xylosus, and S. carnosus | Martínez et al. (2019) |
Dried fermented sausage | Freeze-dried leek powder (75 ppm, 150 ppm) | S. carnosus | Tsoukalas et al. (2011) |
Fermented sausage | Radish (0.5%, 1%) and beetroot (0.5%, 1%) | S. carnosus | Ozaki et al. (2020) |
Mortadella-type sausages | Parsley extract powder (1.07 g/kg, 2.14 g/kg, 4.29 g/kg) | S. carnosus | Riel et al. (2017) |
Pork sausage | Fermented spinach extract (3.0 g/100 g), fermented lettuce extract (3.0 g/100 g), fermented celery extract (3.0 g/100 g), and fermented red beet extract (3.0 g/100 g) | S. carnosus | Hwang et al. (2018) |
Sausage | Celery power (0.8%), fruits extract powder (0.6%), purple sweet potato powder (0.45%), and fruit and vegetable extract powder (0.5%) | (Not mentioned) | Jin et el. (2018) |
Turkish fermented beef sausage (sucuk) | Beetroot (0.12%, 0.24%, 0.35%) | S. carnosus, P. acdilactici, and Lactobacillus sakei | Sucu and Turp (2018) |
Ground meat product | |||
Ground pork meat product | Chinese cabbage powder (0.4%), radish powder (0.4%), spinach powder (0.4%) | S. carnosus | Jeong et al. (2020) |
Cooked ground pork product | White kimchi powder (0.2%), acerola juice powder (0.1%), celery powder (0.4%) | S. carnosus | Choi et al. (2020) |
Others | |||
Cured pork loin | Fermented spinach (10%, 20%, 30%) | L. farciminis | Kim et al. (2017a) |
Cured pork loin | Swiss chard (10%, 20%, 30%, 40%) | Bactoferm S-B-6 (Chr. Hansen, Gainesville, FL, USA) and S. carnosus | Kim et al. (2019a) |
Cured meat model system | Freeze-dried leek powder (0.84%, 1.68%) | (Not mentioned) | Tsoukalas et al. (2011) |
Deli-style turkey | Celery powder (1%, 3.8%) | Lactic acid starter culture | Golden et al. (2014) |
Deli-style turkey breast | Celery powder (0.21%, 0.41%) | S. carnosus | King et al. (2015) |
Meat emulsion | Fermented red beet extract (5%, 10%) | S. carnosus | Choi et al. (2017) |
Pork patties | Swiss chard and celery (2%) | S. carnosus | Shin et al. (2017) |
Nitrate obtained from plant sources can be used in two ways. The first is the direct addition of a plant and starter culture to the brine or product during the manufacturing process. This process requires an incubation time to allow adequate formation of nitrite by the culture. However, this method is difficult to use because the amount of generated nitrite is unknown and depends on the incubation conditions (Sebranek et al., 2012). Accordingly, industries prefer to use different methods. The second method uses the ‘cultured’, ‘prefermented’, or ‘pre-converted’ nitrate-containing plant source, which has already been incubated with starter culture to produce nitrite. Pre-converted plant powder is simple to use because certain nitrites can be applied (Flores and Toldrá, 2020). Sebranek et al. (2012) reported that pre-converted vegetable products typically contain about 15,000–20,000 mg/kg nitrite.
Starter Culture
For manufacturing meat products, several microorganisms, called starter cultures, are used for different purposes. Many types of commercial starter cultures have been developed, and these starter cultures can be divided into four types: lactic acid bacteria, protective bacteria, curing agent cultures, and molds or yeasts (Frece et al., 2014). Among these starter cultures, those that can reduce nitrate to nitrite are essential in meat production (Gassara et al., 2016). Meat products are generally incubated with nitrate and starter cultures at the same time. However, generation of nitrite by this process is often slow, and incubation at appropriate temperature for culture can reduce the quality of meat products (Sindelar et al., 2007). To overcome these limitations, pre-converted nitrite can be added directly to meat products (Kim et al., 2017a; Krause et al., 2011).
Because pre-converted nitrite is formed before adding meat products, the selection of proper starter culture and incubation conditions is essential for the production of stable and abundant nitrite (Sebranek et al., 2012). Starter cultures, which contain lactic acid bacteria, micrococci, yeast, and molds, are generally added to meat during fermentation and have various important roles in fermented meat products. The major roles of lactic acid bacteria, yeasts, and molds are to decrease the pH and improve the flavor of meat products (Miralles et al., 1996; Sunesen and Stahnke, 2003). However, these starter cultures may not be helpful for reducing nitrate to nitrite, except in some microorganisms that express nitrate reductases (Ammor and Mayo, 2007).
Kim et al. (2017b) reported that Lactobacillus strains exhibit nitrate reduction capacity and that L. farciminis, L. coryniformis, L. fructosus, L. reuteri, L. amylophilus, L. hilgardii, L. delbrueckii, L. fermentum, L. plantarum, and L. brevis reduce nitrate. Among these microorganisms, almost all Lactobacillus sp. reduce nitrate to a lesser extent than Staphylococcus carnosus; however, L. farciminis has the most abundant reduction ratio, although the reduction ratio is higher than that of S. carnosus. L. farciminis also reduces nitrate, showing the highest reduction ratio at 30°C for 36–72 h. Coagulase-negative micrococci or staphylococci possess high reduction activity at various temperature ranges (15°C–20°C and over 30°C) (Casaburi et al., 2005). For example, S. carnosus cannot produce nitrite well at low temperatures (6°C), and medium temperatures (24°C) are more suitable than high temperatures (38°C) with regard to the amount of nitrite produced, but the reduction rate is increased at high temperatures compared with that at medium or low temperatures (Krause et al., 2011). Although the incubation temperature is an important factor when reducing nitrate to nitrite, the incubation time is a more critical factor because excessive incubation time induces a decrease in the nitrite content of pre-converted nitrite sources (Kim et al., 2019b; Sindelar et al., 2007). This decline in nitrite content may be due to a decrease in the pH value of the pre-converted nitrite solution. The acidic condition decreases the activity of nitrate reductase and the release of nitrite to NO easily (Paik and Lee, 2014).
Strengths and Weaknesses of Natural Nitrite
Nitrite is an important curing agent with roles in antioxidant activity, antibiotic effects, flavor enhancement, and color development (Honikel, 2008). Because excessive use of nitrite is harmful to human health, various countries regulate and limit the amount of added or residual nitrate and nitrite in meat products (Gassara et al., 2016). Therefore, despite difficulties in developing a complete nitrite substitute, researchers have investigated approaches for replacement of sodium nitrite.
Among the various weaknesses of natural nitrite, residual nitrite content may be the most challenging problem. Pre-converted nitrite, called natural nitrite, may not be harmful to humans; however, the risk of nitrite inducing carcinogenesis cannot be ignored. Amines and residual nitrite in meat can still be a threat to human health because of the formation of n-nitrosamine (Honikel, 2008). Furthermore, although spices can be used to mask vegetable flavor, the flavor of vegetable powder in meat products may not be acceptable to consumers (Kim et al., 2017a; Sebranek and Bacus, 2007). Moreover, some patients may experience allergic reactions to certain vegetables, which could limit the use of natural nitrite sources as a replacement for synthetic nitrite (Sebranek et al., 2012). Despite these weaknesses, natural nitrite may still have applications in the replacement of synthetic nitrite.
In terms of consumer behavior, pre-converted nitrite from vegetable powder could have positive effects because it can be considered ‘natural’. Consumers generally have limited information regarding the form of natural nitrite added to meat products, and this positive feature of such meat products may represent an effective marketing approach (Hung et al., 2016). Furthermore, the residual nitrite contents of meat products supplemented with natural nitrite were found to be lower than those of meat products supplemented with synthetic nitrite in various studies (Choi et al., 2020; Kim et al., 2017a; Kim et al., 2017b; Sindelar et al., 2007). Natural nitrite-containing vegetable powder could accelerate the formation of colorants (NO-MbFe2+) because the acidic conditions of these sources and natural antioxidants in vegetables could help reduce nitrite to NO (Kim et al., 2017a). Therefore, decreased residual nitrite contents reduce the formation of nitrosamine in frying process or the stomach when the same concentration of nitrite is used compared with that of synthetic nitrite (Honikel, 2008). Appropriate addition of natural nitrite does not result in a bad odor or cause the product to have a flavor typical of fermented vegetables, and a significant difference was not observed (Shin et al., 2017). In addition because almost all vegetables contain nitrate, it may be possible to substitute for vegetables that are common causes of allergic reactions (Choi et al., 2017; Hwang et al., 2018; Kim et al., 2019a; Kim et al., 2017a).
Conclusion
Various additives are used to improve the quality characteristics of meat products. The addition of nitrite in the manufacturing of meat products is restricted, and nitrites are thought to have negative effects on health. In this review, we discussed pre-converted nitrite technology as a natural curing agent in processed meat products from a clean label perspective. This approach can eliminate the use of synthetic nitrites, which are disliked by consumers, and instead promote natural curing using natural materials. The development of clean label meat products may be improved by exploiting the strengths and limiting the weaknesses of natural nitrite, and the use of natural ingredients may improve consumer perception of meat products. Overall, pre-converted nitrite technology is expected to have important applications in natural curing of meat products.