Introduction
The consumption of duck meat is increasing worldwide because of reduced intake of red meat, which causes many cardiovascular disorders (Adzitey et al., 2012). Duck meat contains essential elements, such as selenium and iron, and is a good source of proteins (Kim et al., 2016). Therefore, after chicken and turkey, the production of duck meat is increasing worldwide (Matitaputty et al., 2015). In Korea, the consumption of duck meat is continuously increasing and has increased 5-folds from 1997 to 2012 (Korea Duck Association, 2014). Although, duck meat is very famous, the risk of food-borne and meat-borne pathogens is quite high due to higher levels of microbial loads, including pathogens, on a commercial scale (Adzitey et al., 2012). Therefore, use of some technologies to assess the microbial decontamination in food products is currently necessary.
Radioisotope is one of the contaminants can be found in food products. Radioactive materials can be found anywhere, in different kind of meat and meat products, water, and animal feed. In addition, anthropogenic radioisotopes, such as 134Cs and 137Cs, might be released into the atmosphere (water or air) in normal practice or found on a higher scale after an incident. (Asano et al., 2001). The average level of radionuclide Cesium in meat was 0.00000005 Bq/kg before atomic bomb testing (Eisler, 2003). Apart from radioactive contamination, the microbial contamination can also be assessed by different kind of techniques. The total number of contaminating microorganisms was assessed regardless of viability by direct epifluorescent filter technique (DEFT), while aerobic plate count (APC) determines the number of viable microorganisms. The assessment of previous decontamination treatments is possible by comparing the two counts (i.e., DEFT/APC ratios) (Ahn et al., 2013).
Food irradiation, without using heat and chemicals, is an effective method and has an ability to prevent food spoilage by controlling pathogenic and/or spoilage microorganisms (Kim et al., 2014). Ionizing radiations enhance the shelf life and quality of meat by killing the pathogens by targeting their DNA (Akram and Kwon, 2010). Different kinds of pathogens in meat, such as Yersinia enterocolitica, Escherichia coli O157:H7, Listeria monocytogenes, and Salmonella spp., can be easily destroyed by irradiation (Cárcel et al., 2015). The overall microbial quality of the food can be easily increased by irradiation and the dose for meat is up to 7 kGy as recommended by the United States Department of Agriculture (Lung et al., 2015). Irradiation is a promising technology used for preservation of meat without affecting the nutritional as well as sensory attributes (Grolichova et al., 2004). There are different sources of radiations. Electron beam (e-beam) uses high-energy electrons generated from an electron accelerator machine, which is different from gamma rays from radio-isotope sources in terms of safety concerns (Ahn et al., 2013). The e-beam also has an ability of 5-fold reduction in E. coli O157:H7 in inoculated carcasses but it can be vary by different kinds of meat and meat products (Maxim et al., 2014).
The main objectives of this study were to determine hygienic status of commercial cold duck meat samples available in different supermarkets from different regions in Daegu city by monitoring the radioactive contamination as well as microbial loads. In addition, e-beam sensitivity (D10 value) was determined from the linear regression model for the log of surviving bacterial cells and irradiation dose.
Materials and Methods
Commercial cold duck meats were purchased from three different supermarkets in different regions in Daegu, Korea. Ten different samples (1 kg/package: 3 replications) were purchased having the same manufacturing date within circulation date. Ten samples (C1-C10) were classified according to their product types (whole, boneless whole/BW, and boneless slice/BS) and smoking process (smoked and non-smoked). The samples were stored in a refrigerator (4°C) for 1 wk prior to use and all experiments were completed within each circulation dates.
Microbial counts of the total aerobic bacteria (TAB), yeasts & molds (Y&M), and coliforms were evaluated according to the guidelines of the Association of Official Analytical Chemists (AOAC, 2000) and expressed as colony forming units (CFU)/g. Nine kinds of foodborne pathogens were analyzed according to Korea Food Code (MFDS, 2011). The condition for enrichment, isolation, and confirmation of 9 kinds of foodborne pathogens is given in Table 1. Meat sample was added to each enrichment broth and incubated at optimal conditions. Colonies isolated from each selective medium were finally identified using VITEK2 Compact (BioMerieux Ind., France).
*Modified Tryptic Soy Broth (mTSB), Peptone Sorbitol Bile Broth (PSBB), Thiosulfate-citrate-bile salts-sucrose (TCBS), MacConkey Agar with Sorbitol, Cefixime and Tellurite (TC-SMAC), 5-bromo-4-chloro-3-indolyl-β-D-glucuronide (BCIG), Cefsulodin, Irgasan, Novobiocin (CIN).
The commercial cold-duck meat samples were analyzed by gamma-ray spectrometry counting system by using standard analysis method suggested by Association of Analytical Communities with method number 996.05 (AOAC, 1998). The minimum detectable activity for radio-nuclides was quantified at 1 Bq/kg per fresh weight after considering the size and the counting time of the sample.
Analysis was performed according to EN 13783 (2001) to screen whether the samples have been pre-pasteurized. Samples of 10 g were added to peptone saline and diluted 10 times. The solution was diluted by logarithmic dilution series (101-107). Each diluted solution was transferred to the filtration manifold tower containing 10 μm polypropylene filter above 0.6 μm polycarbonate filter for DEFT. After that, staining and rinsing steps were performed. For APC (aerobic plate count), 1.0 mL of each dilution was transferred to a Petri dish containing plate count agar (Difco, Laboratories, USA). The plates were incubated at 30°C for 72 h and then expressed as log10 CFU/g. The DEFT count (X) per gram of meat was calculated by using the mean number of DEFT units per microscope field (N/n), the dilution factor (DF) of the sample, and the microscope factor (MF) of the sample Eq. (1).
The DEFT count was then converted to a logarithmic value. The difference between the DEFT count and APC count is then obtained by subtracting the APC count (logarithmic value) from the DEFT count (logarithmic value).
E-beam irradiation was carried out using an electron accelerator (High Energy Linear Accelerator, 10 MeV, EB Tech, Korea) at doses of 0, 1, 3, and 7 kGy, which were applied for determining the reduction effect of the initial microbial populations. The absorbed doses were measured using an alanine-electron paramagnetic resonance dosimetry system, with an EMS 104 EPR analyzer (Bruker Biospin, Germany).
Two pathogens, Salmonella typhimurium (KCTC 1916) and Listeria monocytogenes (KCTC 3569), were grown in a tryptic soy broth (Difco, Laboratories, USA) at 30°C for 48 h. The pathogens were cultured to a cell density of approximately 106-107 CFU/mL levels. One gram of sterile meat samples (30 kGy) were inoculated with cell suspension (200 mL) of the two pathogens, respectively. Then, it was kept in a sterile workstation for 1 min to allow it to be absorbed. The inoculated samples in the stomacher bag were e-beam irradiated from 0 to 3.5 kGy. Ten gram sample was aseptically homogenized in a sterile stomacher bag containing 90 mL sterile saline solution. After serial dilutions, 100 μL aliquot from an appropriate dilution was plated on to the medium. Medium used for the microbial count was tryptic soy agar (Difco, Laboratories, USA). Plates were incubated at the optimal growing temperature of the bacteria for 48 h and the CFU per gram were counted at 30-300 CFU per plate. D10 values (the dose required to inactivate 90% of a population) for each of the organisms tested were determined by the linear fit of the logarithmic survivors versus irradiation dose points (Kim et al., 2007).
Results and Discussion
Ten different cold commercial duck meat samples (C1-C10) were monitored for total aerobic bacteria (TAB), yeasts & molds (Y&M), and coliform counts, which ranged from 5.18 to 7.23 Log CFU/g, 4.85 to 6.56 Log CFU/g, and 3.72 to 6.04 Log CFU/g, respectively, as shown in Table 2. TAB and Y&M posed a serious microbial threat, and maximum microbial contamination was found in the BS (C4-C6) duck meat samples. Seven different samples (C1-C7) were highly contaminated with coliforms, whereas the smoked BW (C8-C10) samples were not contaminated. The contamination level in duck meat has been studied by many researchers. Kim et al. (2016) reported that the refrigerated whole raw duck meat showed lower TAB than that showed by sliced duck meat. The results for TAB and coliforms of whole duck were also in consistent with the findings of Chae et al. (2006) and Sung et al. (2013). Szosland-Fałtyn et al. (2014) suggested that yeasts and molds in whole duck meat were up to 3.77 and 4.45 Log CFU/g, respectively.
The nine foodborne pathogens were also monitored from different cold duck meat samples (Table 2). The pathogen, L. monocytogenes, was detected in all BS duck meat samples (C4-C6), while Salmonella spp. was only found in BS (C6). The other pathogens were not detected in all the 10 different cold duck meat samples. Haslia et al. (2015) depicted that L. monocytogenes and Salmonella spp. pathogens were detected in the raw duck meat products, which is consistent with the results of the present study. Some other studies are also consistent with the present study and demonstrated that some samples out of 32 cooked and raw meat samples showed contamination with Salmonella spp. (Jalali et al., 2008). Szosland-Fałtyn et al. (2014) reported that about 25% and 6% of L. monocytogenes and Salmonella spp. pathogens, respectively, were present in the duck meat sample. Higher contamination of microorganisms, including L. monocytogenes and Salmonella spp., in commercial cold duck meat may be very lethal for the safety status of meat and in return may seriously threaten the human health.
1)W: whole; BS: boneless sliced; BW: boneless whole. 2)Log CFU/g, Values are means of triplicate experiments; +: Positive; −: Negative.
The contents of radionuclides, such as 131I, 137Cs, and 134Cs, from different commercial cold duck meat samples are given in Table 3. It is evident from the results that there were no radionuclides detected from any of the 10 different samples. The acceptable limit for each radionuclide in foods is different. The maximum permitted concentration of 134Cs and 137Cs is less than 100 Bq/kg in general foods according to the Korean Food Code (MFDS, 2011). According to the Ministry of Agriculture, Forestry, and Fisheries, Japan (MAFF), concentration less than 10 Bq/kg in drinking water, less than 50 Bq/Kg in milk and infant foods, and less than 100 Bq/Kg in meat and fish are acceptable for human consumption (Manabe et al., 2016). The results are somehow consistent with the findings of Brandhoff et al. (2016), who reported that different food samples, including dairy and meat, were subjected to the analysis of selected radionuclides in meat but are less than the acceptable limit. The results were further supported by the findings of Miyazaki et al. (2013) who depicted that about 300 samples were determined for the presence of radioactive iodine (131I) and cesium (137Cs and 134Cs) and it was found that only few samples showed some contamination, but below the regulatory limits.
Sample No. | Radionuclides (Bq/kg fresh weight) | |||||||||
---|---|---|---|---|---|---|---|---|---|---|
|
||||||||||
131I | 134+137Cs | |||||||||
C1 | -1) | - | ||||||||
C2 | - | - | ||||||||
C3 | - | - | ||||||||
C4 | - | - | ||||||||
C5 | - | - | ||||||||
C6 | - | - | ||||||||
C7 | - | - | ||||||||
C8 | - | - | ||||||||
C9 | - | - | ||||||||
C10 | - | - |
1)−: Negative (below the minimum detectable level).
DEFT shows the number of both viable and non-viable cells from the samples, while APC shows the number of viable cells only from the sample (Akram et al., 2012). DEFT/APC technique confirms the microbial decontamination by some previous processing operations. When there is no decontamination treatment, the DEFT count can be similar to that of APC. However, mostly the APC count will be lesser than the DEFT count after decontamination treatments because heat or non-heat processes have an ability to inhibit the growth of viable microorganisms (Ahn et al., 2013). If DEFT/APC ratio is higher than 4, it can be an indication of some processing treatment (EN 13783, 2001). The DEFT/APC results of commercial cold duck meat products are shown in Table 4.
The results showed that DEFT count (9.41-11.35) of commercial duck meat samples was higher than the APC (5.15-7.08). The ratios of DEFT/APC for commercial duck meat samples ranged from 3.02 to 6.20. The cold duck meats (C1-C6) showed DEFT/APC ratios below 3.76; however, the smoked duck meat samples (C7-C10) presented DEFT/APC ratio higher than 4. Higher DEFT/APC ratios were demonstrated by smoked duck meat samples constituting significantly higher difference in microbial contamination, which was attributed to pre-pasteurization process, such as smoking (EN 13783, 2001). Osman et al. (2013) reported that DEFT/APC is a microbiological screening method for irradiation treatments based on comparison of counts. They discriminated the fresh and deboned chicken meat samples based on microbial population. Previously, Sommers and Boyd (2006) reported that this method clearly distinguishes the samples having different level of APC among different samples, which is consistent with the findings of our study where different duck meat samples have different value of APC and DEFT.
The microbial qualities of 10 (C1-C10) commercial cold duck meat samples were evaluated and the sample BS (C6) showed presence of Salmonella typhimurium and Listeria monocytogenes among all the samples as shown in Table 2. We calculated D10 values from graphical analysis and regression equation and determination of D10 values was carried out in accordance with the reported method of Kortei et al. (2014). The sample BS (C6) was designated as model sample and treated with different doses (0.5, 1, 1.5, 2, 2.5, 3, 3.5 kGy) of e-beam and D10 values (kGy) of S. typhimurium and L. monocytogenes were determined. The results showed that the D10 values for S. typhimurium and L. monocytogenes were 0.65 kGy and 0.42 kGy, respectively. The regression (R2) for L. monocytogenes and S. typhimurium was 0.9859 and 0.9615, respectively. The level of contamination decreased with increasing the dose level of e-beam irradiation. L. monocytogenes could not be detected after increasing the dose to 2 kGy, but Salmonella was detected even up to 3.5 kGy as shown in Fig. 1. The results are consistent with the findings of Medina et al. (2009) who reported that the loads of L. monocytogenes in cold smoked salmon were reduced with increasing e-beam irradiation. In another study, application of 3 kGy e-beam irradiation resulted in the reduction of the Salmonella load in beef by 2-4 Log CFU/g, which supports findings of the present study (Li et al., 2015).
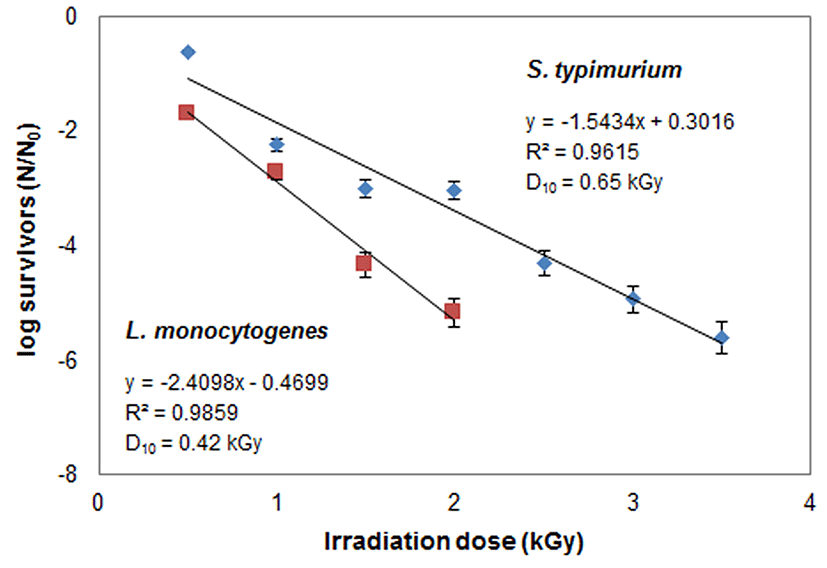
The microbial quality upon e-beam irradiation of the BS (C6) cold duck meat is shown in Fig. 2. Four doses (0, 1, 3, 7 kGy) of e-beam were applied to the BS (C6) cold duck meat sample and the findings indicated that TAB, Y&M, and coliforms were significantly reduced from 9.36 to 3.13 Log CFU/g, 9.19 to 3.00 Log CFU/g, and 3.38 Log CFU/g to the non-detected level, respectively. It is obvious that the initial microbial loads of TAB in the meat sample were effectively reduced in a dose-dependent manner and similar patterns were observed for Y&M and coliforms. Lewis et al. (2002) reported that the use of e-beam at 1.8 kGy significantly reduced the total plate count from 4.60 to 1.62 Log CFU/200 mL in poultry meat. This showed that e-beam irradiation significantly reduced the TAB count. The results are in agreement with the findings of Kim et al. (2013) who reported that application of e-beam dose to pork jerky led to the reduction in TAB count from 4.54 to 2.81 Log CFU/g. Recently, the results were further supported by the reports of An et al. (2017) who depicted that there was reduction in TAB and coliforms were obtained in duck meat.
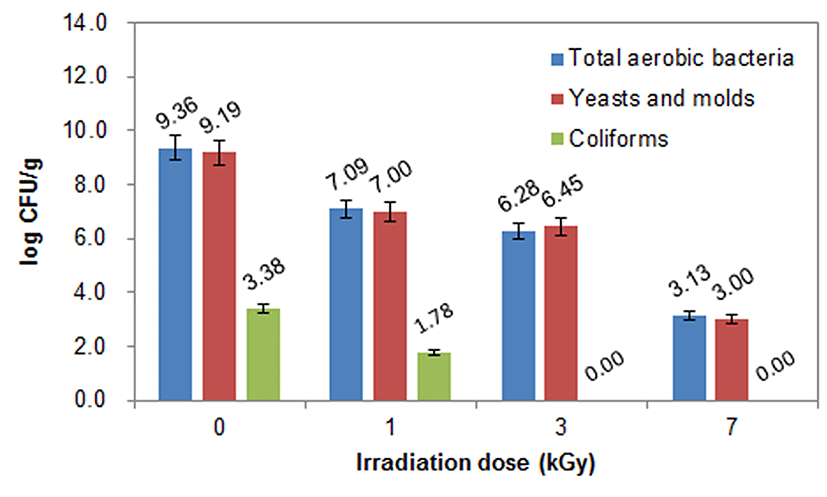
Conclusions
This study aimed to assess the hygienic quality of commercial cold duck meat samples by determining their microbial and radioactive contaminations. The status of prepasteurization treatment was also confirmed by counting the DEFT/APC ratios of each sample. The 10 commercial cold duck meat samples have serious threat of contamination with TAB and YAM (4-7 Log CFU/g). The pathogens, S. typhimurium and L. monocytogenes, were also detected in 3 kinds of duck meat samples. However, there was no radioactive contamination in all commercial duck meat samples. The smoked samples presented DEFT/APC ratios higher than 4, which was due to pre-pasteurization process. E-beam of 7 kGy reduced 6 log cycles of TAB and Y&M populations in a model sample. Conclusively, the commercial cold duck meat samples have a serious threat of microbial contamination, and e-beam irradiation showed a potential to be used for the improvement of microbiological quality of commercial duck meat products. It is recommended that relevant perspective studies should be conducted in future for commercial duck meat products.