Introduction
The prevalence of various diseases, including gastrointestinal disorders and constipation, has increased due to the westernization of the Korean diet and stress caused because of industrialization. Constipation is experienced by approximately 5 to 20% of the entire population in USA (Locke, 1996). Constipation causes headaches, as well as accelerated skin aging, acne, and other skin disorders that develop when unexcreted fecal toxins are absorbed into the intestine and blood stream. Furthermore, severe constipation causes anal fissures and herniation of hemorrhoids, and can contribute to the development of colon cancer (Corazziari, 1999). For this reason, interest in natural treatments for constipation is increasing, and several studies investigating natural products, including lactic acid bacteria (LAB) or fermented by LAB, have been reported (Choi et al., 2012; Choi et al., 2014a; Choi et al., 2014b; Im et al., 2011; Koebnick et al., 2003).
The mulberry plant, which belongs to the genus of Morus and the family Moraceae, is broadly distributed in tropical and temperate regions (Chung et al., 1996). The mulberry leaf contains 2.7-3.1% and 4.1-7.4% minerals and vitamins, respectively, as well as a relatively high content of γ-aminobutyric acid (Bang et al., 1998), a known hypotensive substance, which makes it a useful functional food ingredient (Omori et al., 1987). It is also reported to contain flavonoids such as rutin, quercetin, quercitrin, and isoquercitrin, as well as alkaloids including 1-deoxynojirimycin (DNJ), which demonstrate α-glucosidase inhibitory activity (Yoshikumi, 1994). In addition, mulberry leaf has been reported to contain relatively high levels of polyphenolic compounds such as chlorogenic acid, isoquercitrin, quercetin 3-O-(6-O-malonyl)glucoside, stragalin and kaempferol 3-O-(6-O-malonyl)glucoside (Choi et al., 2015). Mulberry leaf has been shown to possess anti-hypertension (Omori et al., 1987), hypoglycemic (Asano et al., 1994), antioxidative (Doi et al., 2000; Yoshikumi, 1994), anti-obesity (Lim et al., 2013) and anti-hyperlipidemic properties (Kim et al., 1998; Lee et al., 2011). In addition, according to Lee et al. (2001), the composition of gastrointestinal microflora of rats was improved by treatment with mulberry leaf; this treatment was effective for growth inhibition of harmful intestinal bacteria.
LAB, which is widely distributed in nature, have been used for the fermentation of various foods, is generally recognized as safe (Sandine et al., 1972). LAB, when used as a probiotic strain to promote the growth of beneficial bacteria, have recently been reported to facilitate the enhancement of gastrointestinal functions such as therapeutic effects of diarrhea and constipation, inhibiting action of intestinal decomposition and harmful intestinal microorganisms, and prevention of various diseases (Bang et al., 2012; Gilliland, 1990; Szajewska et al.,2006). Recently, interest in the functionality of fermented foods has increased; numerous studies on the development of new health-enhancing foods are therefore being performed via fermentation with LAB (Hyun and Hur, 2011). The bioavailability of a natural substance often increases after its fermentation using microorganisms, because enzymes and biologically active components in the intracellular organelles are released (Katina et al., 2007). Thus, fermentation is used to improve the nutrition values and increase the production and extraction yields of bioactive compounds in the vegetables and fruits. Many studies have reported that vegetables and fruits fermented by LAB exhibited high levels of polyphenolic and flavonoid compounds, and radical scavenging activity (Lee et al., 2015a; Lee et al., 2015b; Lee and Paik, 2017; Park et al., 2015; Yang et al., 2014). Therefore, the fermented mulberry leaf using LAB are expected to have increased functionality due to increased content of bioactive compounds. Certain polyphenolic and flavonoid compounds are known to exhibit laxative properties (Méité et al., 2010; Sathyanathan et al., 2013; Seo et al., 2013).
This study evaluated the preventative effects of polyphenol-rich LAB-fermented mulberry leaf extract (MLE) on constipation by examining intestinal function and constipation symptoms in loperamide-induced constipated rats.
Materials and Methods
The experimental protocol used in this study was approved by the Institutional Animal Care and Use Committee of Chosun University (CLACUC2015-S0027).
LAB-fermented ML powder was prepared and supplied by Bioresource, Inc (Korea). ML powder (MLP, 100 g) was suspended in 1 L distilled water and sterilized using an autoclave at 121°C for 15 min. The microorganisms used consisted of a mixture of three kinds of LAB (Lactobacillus bulgaricus, Streptococcus thermophilus, and Lactobacillus acidophilus) provided by the Korean Culture Center of Microorganisms. The bacteria mixture was cultured in MRS broth (peptone 10%, beef extract 10%, yeast extract 9%, dextrose 36%, polysorbate 1.8%, ammonium citrate 3.6%, sodium acetate 9%, magnesium sulfate 0.2%, manganese sulfate 0.1%, and dipotassium phosphate 3.6%). The visual cell counts (CFU/mL) of the inoculum were determined by the standard plate method with MRS medium after 24 h of incubation at 37°C. For fermentation, the starter culture inoculated into 10% ML medium, followed by 3 d of incubation at 37°C with shaking at 100 rpm. After fermentation, the culture ML medium was then freeze-dried (ED 8512, IlShin, Korea).
To obtain ethanol extracts, 100 g of lyophilized ML fermentate was added to 1,500 mL of 80% ethanol. The lyophilized ML fermentate was stirred on a plate stirrer for 24 h at 25°C, and the extracted ethanol solution was filtered through Whatman filter paper (No. 2, GE Healthcare, UK). The solvent was removed from the remaining extract using a rotary vacuum evaporator (EYELA VACUUM NVC-1100, Japan). The extracts were then concentrated, lyophilized, and stored at −70°C until use.
The total polyphenol contents of MLE and LAB-fermented MLE were determined using the modified Folin-Cicocalteu method (Folin and Denis, 1912) and expressed as a tannic acid equivalent (mg/g). Total flavonoid contents were measured according to the Davis method with slight modifications (Chae et al., 2002) and calculated as a rutin equivalent (mg/g). Chlorogenic acid and DNJ contents were analyzed by high-performance liquid chromatography (Waters HPLC system, Waters Crop., USA). Chlorogenic acid contents were measured according to the Coseteng and Lee method (1987) with slight modifications. DNJ contents were assessed using the modified method of Stead and Richards (1996). The results represent the mean data of three parallel determinations.
Male Sprague-Dawley rats (n=32) with a mean weight of 305-310 g were obtained from Central Laboratory Animal Inc. (Korea). They were acclimatized to the housing conditions for 7 d, during which they were allowed free access to commercial pelleted rat chow (Samtako Co. Ltd., Korea) and water. They were then divided into four groups of eight rats each, using the randomized blocks design, and one rat from each group was separately raised for 33 d. Food pellets (Samtako Co. Ltd., Korea) prepared based on AIN-93 rodent diet. The four experimental groups consisted of the normal group (N), loperamide treated group (C), loperamide and LAB-fermented MLE 300 mg/kg b.w treated group (MLEL), and loperamide and LAB-fermented MLE 600 mg/kg b.w treated group (MLEH). LAB-fermented MLE groups were orally administered once daily for 33 d, while N and C groups were administered with saline. To induce constipation, loperamide (2.0 mg/kg b.w) was dissolved in 0.5% (v/v) Tween 20 and subcutaneously injected into all groups, except for the N group, twice a day for 5 d from day 29 to 33 (Shimotoyodome et al., 2000). Normal rats were injected 0.5% (v/v) Tween 20 in the same manner as the experimental groups. The temperature and relative humidity of the animal room were maintained at 22±1°C and 65±5%, respectively, with a controlled 12-h light/dark cycle (light, 09:00-21:00). Throughout the entire experimental period, water and feed were provided ad libitum, and the feed intake and body weight were measured weekly.
The animals were fasted for 12 h after loperamide injection, lightly anesthetized with carbon dioxide, and blood was collected. The blood samples were centrifuged at 1,150 g for 20 min. The serum was separated and analyzed for its lipid content.
The number, weight, and water content of the fecal pellets were determined before and after the induction of constipation. The feces of each experimental animal were collected four times on day 1 of the experiment, followed by every 7 d, and then every day during the constipationinduction period. The dry weight was measured after the feces were dried at 70°C for 24 h; the fecal water content was calculated as the difference between the fresh and dry fecal weights, divided by the fresh fecal weight.
The intestinal transit time of the consumed diet was determined based on the method of Hinton et al. (1969). This parameter was calculated as the total time required for blue feces to be excreted after the animals were moderately fed for 12 h on day 6 following loperamide administration, with 10% Coomassie brilliant blue dye (1 g) added to the next feed. The intestinal length, including both the small and large intestines and excluding the appendix, was measured once and then resected.
The number of fecal pellets in the large intestine was determined following the euthanasia of rats on day 6 after loperamide administration. The entire intestine from the appendix to the rectum was resected and fixed with 10% formaldehyde (Shimotoyodome et al., 2000).
After rat sacrifice, viable fecal Lactobacillus were enumerated using the candle jar method (plate-in-bottle method, Mitsuoka et al., 1969) with MRS agar. After collection, fecal samples were stored in liquid nitrogen prior to the enumeration of Lactobacillus. These samples were then thawed at room temperature, and Lactobacillus-selective agar plates containing a 10-fold dilution of the test agents were incubated at 37°C for 96 h under anaerobic conditions using candle jars. After 96 h of incubation, colonies were counted (× 108 CFU/mL). Lactobacillus numbers were then determined.
The serum triglyceride (TG), total cholesterol (TC), and high-density lipoprotein (HDL)-cholesterol contents were measured using a blood biochemistry automatic analyzer (Fuji Dri-Chem 3500s, Fujifilm, Japan). The low-density lipoprotein (LDL)-cholesterol content was calculated using the Friedwald formula (Friedwald and Fredrickson, 1972).
The experimental data were analyzed using general linear model (GLM) analysis via the Statistical Analysis System (SAS) software package (SAS Institute Inc., USA) and expressed as the mean±standard error (SE). The significance of the means of each experimental group was verified using Tukey’s test at α<0.05.
Results and Discussion
Table 1 shows functional components in MLE and LAB-fermented MLE. Total polyphenol, total flavonoid and chlorogenic acid contents of the LAB-fermented MLE were slightly higher than those of the MLE. However, there were no significant differences in DNJ content between MLE and LAB-fermented MLE. Park et al. (2015) also reported that magnolia flower petal extract fermented by Pediococcus acidilactici KCCM 11614 had greater total polyphenolic compounds content, as well as antioxidative and anticancer effects than those of the non-fermented extract. Phenolic compounds have been reported to affect the growth of LAB (Etxeberria et al., 2013). In addition, bioactive compounds including polyphenols, polydatin, and resveratrol extracted from peanut sprout (Seo et al., 2013) and flavonol naringenin extracted from citrus fruits (Yang et al., 2008), have been reported to exert laxative properties.
1)Values are means±S.E. of triplicate experiments.
Body weight gain, feed intake, and feed efficiency ratio (FER) of the rats orally treated with LAB-fermented MLE (300 and 600 mg/kg) before and after administration of loperamide are shown in Table 2. Prior to loperamide administration, the body weight gain was significantly lower in the LAB-fermented MLE groups than in the C and N groups. There was no difference between groups in the feed intake prior to loperamide administration. After loperamide administration, the body weight gain was significantly lower in the MLE groups and C group treated with loperamide than in the N group. The feed intake increased in the MLE groups after loperamide administration, when compared with the C group. These results showed a similar trend to a study by Jeon et al. (2007), which reported that loperamide administration was decreased feed intake and consequently affected body weight. Generally, feed intake is known to be a significant factor in the evaluation of constipation symptoms (Im et al., 2011; Jeon et al., 2007). Im et al. (2011) also induced constipation in rats via the administration of loperamide. They reported that the feed intake of these rats increased when they were orally fed dietary fiber-rich functional yogurt, when compared to rats that received only loperamide. In this study, after loperamide administration, FER decreased in the LAB-fermented MLE groups when compared with the N and C groups. There were no differences in the body weight gain, feed intake, and FER between the MLEL and MLEH groups before or after loperamide administration.
1)N, normal group; C, loperamide treated group; MLEL, loperamide and LAB-fermeneted MLE 300 mg/kg b.w. treated group; and MLEH, loperamide and LAB-fermented MLE 600 mg/kg b.w. treated group.
2)FER, Feed efficiency ratio = body weight gain (g)/feed intake (g).
3)Mean±S.E. (n=8).
4)Values with different superscripts in the same row are significantly different (p<0.05) between groups as determined via Tukey's test.
5)NS, not significantly different among groups.
The number, weight, and water content of the fecal pellets of rats orally treated with LAB-fermented MLE before and after loperamide administration are shown in Table 3. There was no significant difference in the fecal pellet number, fecal wet weight, and fecal water content between the groups prior to loperamide administration. The fecal number, wet weight and water content after loperamide administration were higher in the MLEH and N groups than in the MLEL and C groups. The number, weight, and water content of the fecal pellets, before and after loperamide administration, were higher in the MLEH group than in the MLEL group. Loperamide is an antidiarrheal medicine that prolongs the defecation time by inhibiting bowel movements (Schiller et al., 1984). In this study, loperamide administration reduced the number, weight, and water content of fecal pellets; these symptoms were alleviated by supplementation with LAB-fermented MLE. These results suggested that LAB-fermented MLE consumption increased fecal volume and defecation frequency. Previous studies investigating the effect of different interventions on the number and weight of fecal pellets revealed that there were increased in groups treated a functional yogurt containing LAB (Koebnick et al., 2003), dietary fiber (Im et al., 2011), fermented rice extract (Choi et al., 2014a), or polyphenol-rich compounds (Méité et al., 2010; Sathyanathan et al., 2013; Seo et al., 2013). According to Choi et al. (2014), increases in the number, weight and water content of the fecal pellets in rats were found with fermented rice treatment, and these results were considered to be direct evidence that fermented rice extract shows laxative effects.
1)N, normal group; C, loperamide treated group; MLEL, loperamide and LAB-fermeneted MLE 300 mg/kg b.w. treated group; and MLEH, loperamide and LAB-fermeneted MLE 600 mg/kg b.w. treated group.
2)Mean±S.E. (n=8).
3)NS, not significantly different among groups.
4)Values with different superscripts in the same row are significantly different (p<0.05) between groups as determined via Tukey's test.
Intestinal transit time and length in rats treated with LAB-fermented MLE are shown in Table 4. The intestinal transit time was significantly decreased in the MLEH group compared with the C group, and similar to that of the N group. It has been reported that, although dietary fiber prolongs the transit time in the stomach and small intestine, the transit time in the large intestine is shortened; therefore, the final defecation time is shortened (Chung et al., 1996). However, intestinal length was not statistically different among the experimental groups although it tended to be longer in the MLEH group than the other groups.
Groups1) | ||||
---|---|---|---|---|
|
||||
N | C | MLEL | MLEH | |
Transit time (min) | 509.43±30.432)a3) | 691.43±43.21b | 670.12±35.21b | 611.54±29.43a |
Intestine length (cm) | 137.29±9.86NS4) | 128.35±10.97 | 134.21±16.21 | 140.31±11.08 |
1)N, normal group; C, loperamide treated group; MLEL, loperamide and LAB-fermeneted MLE 300 mg/kg b.w. treated group; and MLEH, loperamide and LAB-fermeneted MLE 600 mg/kg b.w. treated group.
2)Mean±S.E. (n=8).
3)Values with different superscripts in the same row are significantly different (p<0.05) between groups as determined via Tukey's test.
4)NS, not significantly different among groups.
Dietary therapy, including the sufficient intake of dietary fiber; probiotics; fluids, milk and milk products; and fruits such as green kiwifruit, prune, persimmon and banana; is a simple and beneficial treatment for constipation (Ait-Belgnaoui et al., 2006; Bae 2014; Chung et al., 1996; Im et al., 2011). Constipation cannot be managed using medication alone. The effects of probiotics, such as LAB, are known to be related to their effects on intestinal peristalsis, including intestinal pH reduction by low-quality fatty acid production; the resulting low-pH environment increases intestinal peristalsis and decreases intestinal transit time (Szajewska et al., 2006). Moreover, it has been reported that probiotics improve the shape and concentration of the feces by decomposing bile acids and stimulating the intestinal nerves, which activate bowel movements (Ait-Belgnaoui et al., 2006). Dietary fiber is known to absorb water in the large intestine and thereby softens the feces and increases its volume (Meance et al., 2001). It also promotes the growth and byproducts of microorganisms (Chen et al., 1998) and stimulates intestinal peristalsis (Anti et al., 1998). Furthermore, dietary fiber has been reported to affect the intestinal length. It is not fully digested in the small intestine, but is decomposed by microorganisms and absorbed in the appendix, colon, and rectum, which is enlarged and, consequently, the total intestinal length is increased (Dowling, 1967). The dietary fiber obtained from food does not contain toxins and is safe; therefore, its long-term consumption is possible (Lee et al., 2011; Poller, 1970). In this study, the total dietary fiber content of the LAB-fermented MLE was as high as 38% (data not shown) and therefore influenced the gastrointestinal functions of rats that consulted the LAB-fermented MLE treatment. The intestinal transit time of the groups treated LAB-fermented MLE was the fastest and intestinal length was the longest. Among bioactive compounds of MLE, the DNJ, which is known to inhibit the α-glucosidase activity, is also thought to play a role in constipation effect. DNJ has been shown to inhibit glucose uptake in the small intestine, thereby, increase osmotic pressure and water retention of stool, accelerate the intestinal transit time of bowl movement, and soften consistency, all of which may alleviate constipation (Asano et al., 1994; Lee et al., 2000).
The effect of LAB-fermented MLE on the number of fecal pellets in the constipation-induced rat colon is shown in Fig. 1. The number of fecal pellets in colon was lowest in the N group that was not administrated with loperamide; it was remarkably decreased in the MLE groups when compared with the C group. While constipation was induced in the MLE groups treated with LAB-fermented MLE, the number of fecal pellets decreased to a level similar to that seen in the N group fed a normal diet without constipation induction. These results suggest that loperamide may have induced constipation by reducing intestinal peristalsis (Schiller et al., 1984). Furthermore, the LAB-fermented MLE contributed to reducing the intestinal transit time and, thereby, decreased the number of fecal pellets (Lumpton and Morin, 1993).
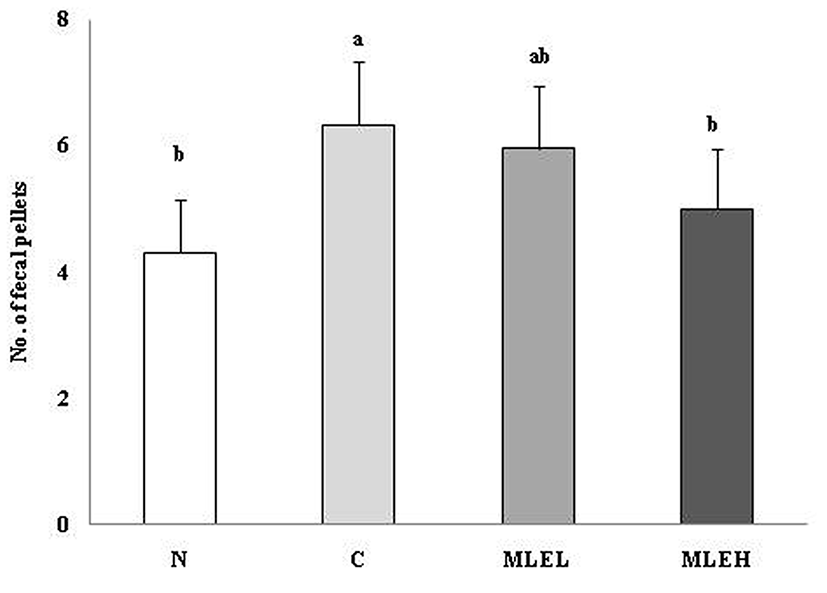
The effect of LAB-fermented MLE on viable Lactobacillus numbers in feces is shown in Fig. 2. A significant increase in viable Lactobacillus was detected in the MLE groups when compared with the other groups. The viable fecal Lactobacillus numbers was significantly greater in the MLEH group than in the MLEL group. The intestinal flora plays an important role in the physiological functions of the alimentary tract. Probiotics, such as Lactobacillus, have been suggested as alternative therapies for various digestive disorders, and they have shown favorable effects on constipation, with fewer toxic effects than pharmaceutical treatments (Choi et al., 2014b). In addition, probiotics alleviate colic, which are associated with severe constipation (Savino et al., 2010). Lee et al. (2001) reported that the composition of gastrointestinal microflora was improved by treatment of ML in rats and very effective for growth inhibition of the harmful bacteria in intestine.
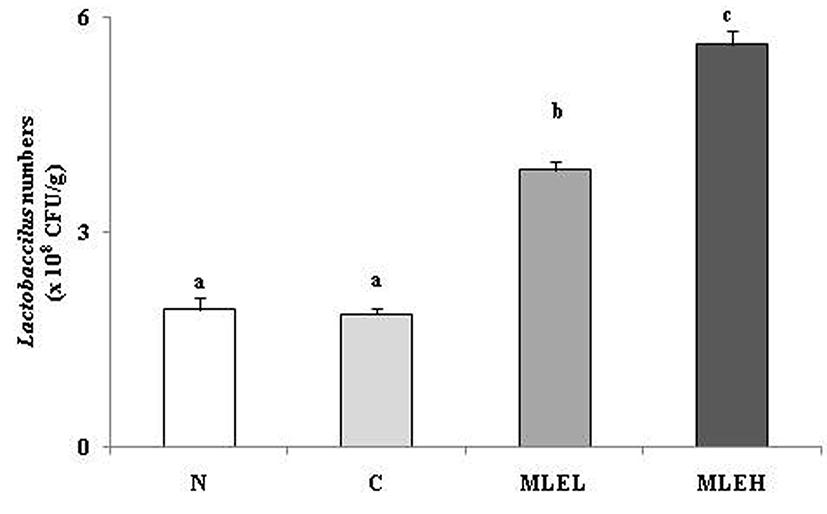
The effects of LAB-fermented MLE on serum lipid profiles are shown in Table 5. Serum TG, TC, and LDL-C levels tended to be lower in the MLE groups than in the C and N groups. Serum HDL-C level significantly increased in the MLE groups compared to the C and N groups. Similar results were reported by Kim et al. (1998), who showed that, following high cholesterol induction, MLE decreased total cholesterol, LDL-C, and TG levels but increased HDL-C level and, therefore, improved lipid metabolism. Moreover, MLE is known to effectively inhibit cholesterol synthesis (Asano et al., 1994; Kim et al., 1998). It was postulated that the high dietary fiber level and functional components of green tea powder improve the serum lipid profile by inhibiting the absorption of cholesterol and stimulate the excretion of bile acids (Russell and Setchell, 1992). Many studies reported that vegetables and fruits by fermented LAB improved the serum lipid profiles (Lee et al., 2015a; Park et al., 2015). Moreover, the bioactive compounds of ML were increased by fermentation of LAB, and these results also indicated that the improvement of serum lipid profile was shown.
1)N, normal group; C, loperamide treated group; MLEL, loperamide and LAB-fermeneted MLE 300 mg/kg b.w. treated group; and MLEH, loperamide and LAB-fermeneted MLE 600 mg/kg b.w. treated group.
2)Mean±S.E. (n=8).
3)Values with different superscripts in the same row are significantly different (p<0.05) between groups as determined via Tukey's test.
The leaf extracts of medical or herbal plants have long been very important sources of drugs against constipation (Méité et al., 2010). Laxative properties of plants found to be due to tannins, alkaloids, saponins, flavonoids, sterols, terpenoids, and reducing sugars (Méité et al., 2010; Sathyanathan et al., 2013; Seo et al., 2013). Therefore, in this study, these bioactive compounds, such as total polyphenol, total flavonoid, chlorogenic acid and DNJ may mediate the laxative properties of LAB-fermented MLE.
In conclusion, the LAB-fermented MLE increased the number, weight, and water content of the fecal pellets in loperamide-treated animals. In addition, LAB-fermented MLE showed constipation-preventing effects by reducing the intestinal transit time and the numbers of fecal pellets in the colon, and increasing the fecal Lactobacillus numbers. However, the exact mechanism of laxative effect of the LAB-fermented MLE is not known, and needs further studies.