Introduction
Constipation is a common problem, particularly in the elderly, and probiotics have been proposed to improve bowel motility (Gallagher et al., 2008, Müller-lissner et al., 2010). Symptoms of constipation include the following: decrease in the frequency of bowel movements, decrease in the amount of feces, painful bowel movements, dried feces, and dissatisfaction after a bowel movement (Beery and Chokshi, 2014). In terms of improving the colon function of patients suffering from constipation, assessing the nutritional impact of intestinal environment is important. Over the last few decades, studies on the relationship between constipation and nutrition have focused on fibrous and non-digestive oligosaccharides (Dukas et al., 2003, Guimaraes et al., 2001, Morais et al., 1999). Constipation has a significant impact on the quality of life, and prebiotics and probiotics are expected to help in the treatment of constipation (Rothbarth et al., 2001, Whitehead et al., 2001). Therefore, there is a growing interest in better understanding the effects of probiotics on constipation.
Probiotics are defined as living microorganisms that enter the gastrointestinal tract in sufficient numbers of active forms to exert positive effects (Heller, 2001, Sanders, 2008).
The concept of stabilizing intestinal microorganisms via the associated lactic acid bacteria for health improvement dates back to the beginning of the last century. Many studies have been conducted on the influence of probiotic cultures on health (Bezkorovainy, 2001; Fuller, 1992; Isolauri, 2001; Kalliomäki et al., 2001; Kopp-Hoolihan, 2001, Spanhaak et al., 1998). Ingestion of probiotic microorganisms is beneficial for treating different diarrhea-like asthma disorders, promoting lactose digestion, and lowering the levels of metabolites that are harmful to health, including cancerous markers in the colon (De Roos and Katan, 2000, De Vrese and Marteau, 2007). Probiotic microorganisms, members of the genera Lactobacillus and Bifidobacterium, in particular, promote various immunomodulatory effects by modulating the gut microbiota. (Isolauri et al., 2002, Mazmanian et al., 2008). Thus, probiotic bacteria could be used for the treatment of constipation because of their health-promoting benefits. However, there have been few studies regarding the role of various individual lactic acid bacteria or mixtures of different strains in the mitigation of constipation.
The purpose of this study was to investigate the effects of different kinds of lactic acid bacteria (LAB) and a mixture of the strains on constipation relief in rats. In the future, the highly effective single lactic acid bacteria or a mixture of lactic acid strains used in this study could be used commercially.
Materials and Methods
LAB powder was supplied by Chong Kun Dang Bio Corp. (Korea). Enterococcus faecium CKDB003(EF), Lactobacillus acidophilus CKDB 007(LA), Streptococcus thermophilus CKDB021(ST), Bifidobacterium bifidum CKDB001(BB), Bifidobacterium lactis CKDB005(BL), Pediococcus pentosaceus KID7 (PP), the mixture (1:1:1: 1:1 based on CFU of each strain) of 5 strains, except Pediococcus pentosaceus KID7(CK-DB), and the mixture (1:1:1:1:1:1 based on CFU of each strain) of all 6 strains were used in the 8 kinds of LAB preparations.
Three batches of fermented milk were prepared in a 250-mL glass container, each containing 14% skim milk powder. Each batch of fermented milk was analyzed separately. Skim milk solution was sterilized at 121°C for 10 min and cooled to 42-45°C. After cooling, milk was inoculated with a 1% (v/v) starter-culture of CKDB and CKDBP. Fermentation was conducted at 37°C for 12 h.
The pH values of the fermented milk and milk samples were measured at 17-20°C using an Orion 410A pH meter (Orion, USA), which was calibrated using freshly prepared pH 4.0 and pH 7.0 standard buffers. The titratable acidity was determined after mixing a fermented milk sample with 10 mL of hot distilled water and titrating it with 0.1 N NaOH using 0.5% phenolphthalein indicator.
Microbiological analyses of fermented milks included determination of the number of microbes in the starter-culture. One gram of fermented milk sample was diluted with 9 mL of 0.15% peptone water (Oxoid, Australia) and mixed uniformly with a vortex mixer. Subsequent serial dilutions were prepared, and viable numbers were enumerated using the pour plate technique. De Man, Rogosa and Sharpe (MRS) agar (Oxoid, Australia) was used for assaying the total starter culture by incubating the plates at 37°C for 48 h.
Male SD-Rat (160-180 g, 6 wk old) were purchased from Orient Bio (Korea) and grown in individual cages. The experimental animals were kept at a temperature of 21±1°C and relative humidity of 50-55%. After 5 d of adaptation, loperamide (3 mg/kg) was orally administered (per oral, PO) to the groups, except for the normal control, once a day for the same time. Various lactic acid bacteria (108 CFU/kg) were orally administered once a day for 14 d after induction of constipation in the experimental animals was confirmed, and then the animals were sacrificed.
Body weight, food intake, and water intake of the animals were measured every 3 d. Food and water intake were measured once a day during the experimental period of inducing constipation using loperamide. Dietary efficiency was calculated by dividing the food intake by the weight gain, from the first day of the constipation to the day of sacrifice.
Food efficiency ratio (FER) (%) = B / A × 100
A: Weight gain (based on the first day of constipation induction and the day of sacrifice)
B: Food intake (based on the first day of constipation induction and the day of sacrifice)
The number of stools, weights of fecal pellets, and water content in stools were measured once a day during the loperamide-induced constipation period. After inducing constipation, the number of stools, weights of fecal pellets, and water content in stools were measured every 3 d. For measuring the water content, the fecal sample was dried at 70°C for 12 h, and the dry weight was measured. The difference in the weight before and after drying was calculated as the percentage of wet weight.
Serum samples were collected by centrifugation using an automatic serum analyzer (Dri-chem3500i, Fujifilm, Japan). Total cholesterol (TCHO), HDL-cholesterol (HDL), triglyceride (TG), and glucose (GLU) contents were analyzed using an automatic serum analyzer (Dri-chem3500i, Fujifilm, Japan). Weights of liver, heart, kidney, spleen, bowel (excluding appendix), and cecum were measured. The measured organ weight was calculated as the value corresponding to 100 g of the experimental animal.
Intestinal transit ratio of bowel contents was measured using a modified method from Baik et al. (Baik et al., 2004). To investigate the effect of administration of LAB on intestinal transit, the activated carbon diet (8% charcoal meal) was orally administered to experimental animals, and they were sacrificed 30 min later to excise their gastrointestinal tract. The length of the intestine was measured by summing the length of the small intestine and the large intestine (excluding the cecum), and the intestinal transit ratio was calculated by dividing the intestinal distance traveled by the charcoal meal by total length of the intestinal tract.
T (%) = B / A × 100
where T: Intestinal tract ratio
A: Total length of small intestinal tract
B: Distance traveled by the charcoal meal
To observe the intestinal tissues, paraffin embedding was performed, the tissues were deparaffinized with xylene, and then subjected to 100%, 90%, 80%, and 70% ethanol for 5 min, followed by treatment with hematoxylin solution (Sigma-Aldrich, USA) for 3 min. The stained tissue was washed and re-stained with eosin solution for 3 min. After the dyeing was completed, the washed tissue was dehydrated using 70%, 80%, 90%, and 100% ethanol for 5 min, washed with xylene, and sealed. The stained intestinal mucosa cells were observed under an optical microscope (Zeiss, Axiovert S100, Germany).
Tissue sections (5 µm thickness) were deparaffinized in xylene and rehydrated in a graded series of alcohol solutions (100, 90, 80, and 70%) with distilled water. Subsequently, antigens were retrieved by heating with citrate buffer solution in a microwave oven at 98°C for 20 min and then immersing in phosphate buffered saline (PBS). Endogenous peroxidase was blocked by incubating the sample with 3% H2O2 for 10 min, and nonspecific binding was blocked by incubating the sample with normal goat serum for 10-15 min. The sections were then incubated with primary rabbit anti-c-kit antibodies (1:200) at 37°C for 2 h. After washing in PBS, sections were further incubated with biotin-conjugated secondary antibodies for 10 min at 37°C. The sections were subsequently incubated with streptavidin-biotinylated horseradish peroxidase for 15 min at 37°C. Slides were developed using a DAB horseradish peroxidase color development kit (ZhongShan JinQiao, China), then the sections were counterstained with hematoxylin and mounted in neutral balsam. Tissue sections were examined under a light microscope, and the number of pixels with RGB values in the dyed interstitial cells of Cajal (ICC) was determined using the MATLAB software.
Area of ICC in intestine (%) = (Number of pixels with specific RGB values/Total number of pixels) × 100
To measure the concentration of short chain fatty acids (SCFs), 1 g of feces was extracted with 5 mL of methanol and centrifuged at 28,000 × g for 15 min. The extract was stored at −60°C until use. The stored sample was thawed, filtered through a 0.45-µm Millipore filter (Millipore, USA), and analyzed using a DB-FFAP 123-3253 (50 m × 0.32 mm × 0.50 µm), a flame ionization detector equipped with an Agilent 6890N gas chromatography system (Agilent Technologies, USA). Nitrogen gas was used as the carrier at a flow rate of 1.4 mL/min and a sample injection volume of 1 µL. Inlet and detector temperatures were 200°C and 240°C, respectively. Acetic acid, propionic acid, and butyric acid contents were evaluated by constructing a calibration curve using the respective standard reagents.
Metagenomics analyses were conducted to identify changes in the cecal microflora. Metagenomic DNA was extracted using a DNA extraction kit and a bead-beater within one hour after sample collection to obtain DNA reflecting the microbial community structure of the sample. The extracted DNA was electrophoresed on an agarose gel, and a total DNA sample for next generation sequencing (NGS) was prepared for each treatment. NGS was analyzed by Chunlab (Chunlab, Inc., Korea), a specialized analysis company.
Results were expressed as mean ± standard error of the mean (SEM), and were analyzed using the Statistical Package for Social Sciences version 12.0 (SPSS Inc., USA). An analysis of variance (ANOVA) test was used to determine the differences among samples at a significance level of p<0.05.
Results and Discussion
The weight gain was significantly (p<0.05) lower in the constipation induced-control group (122.20 g), as compared to that in the normal group (176.41 g), but was similar to that in the normal group in the group treated with the LAB (Fig. 1A, 1B). There was no significant difference in the food efficiency values between the experimental groups (Fig. 1C, 1D). These results are similar to those reported by Kim et al. (2015).
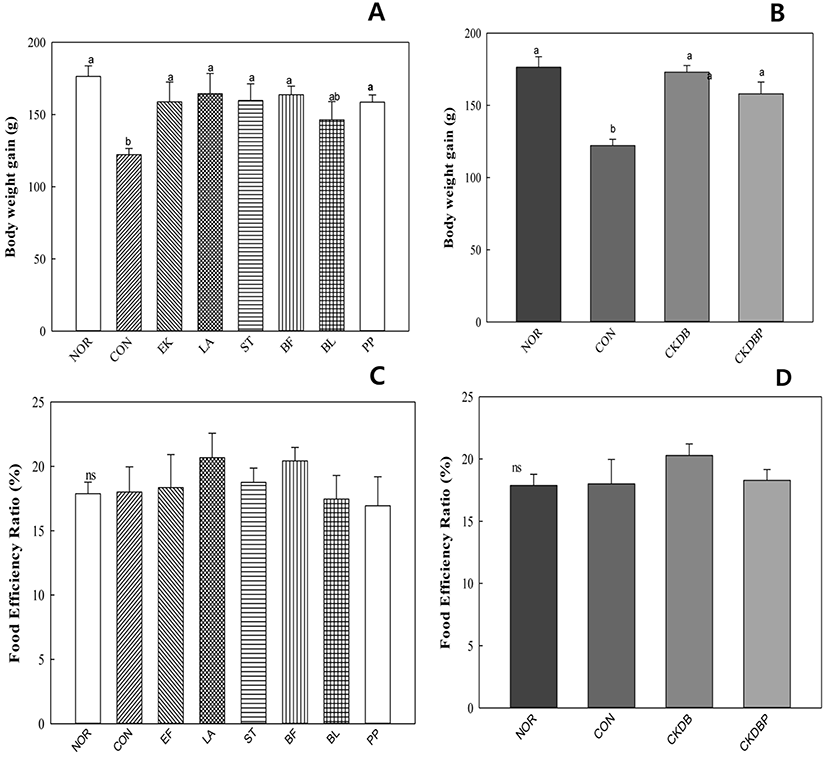
Low water content in the stool reduces bowel activity and causes symptoms of constipation. Therefore, water content could be an indicator of constipation degree and constipation relief. The measured water contents of the different stool samples are shown in Fig. 2A and 2B. The water content of the control group (CON: 27.51%) was lower than that of the normal group (NOR: 29.01%), indicating that constipation was induced. The stool water contents of EF (33.18%), LA (32.31%), ST (30.87%) and CKDBP, the mixture of 6 strains (30.56%) were significantly higher than that of the control group (p<0.05). However, pH values of the stool samples did not show any significant differences in the control and experimental groups (Fig. 2C, 2D).
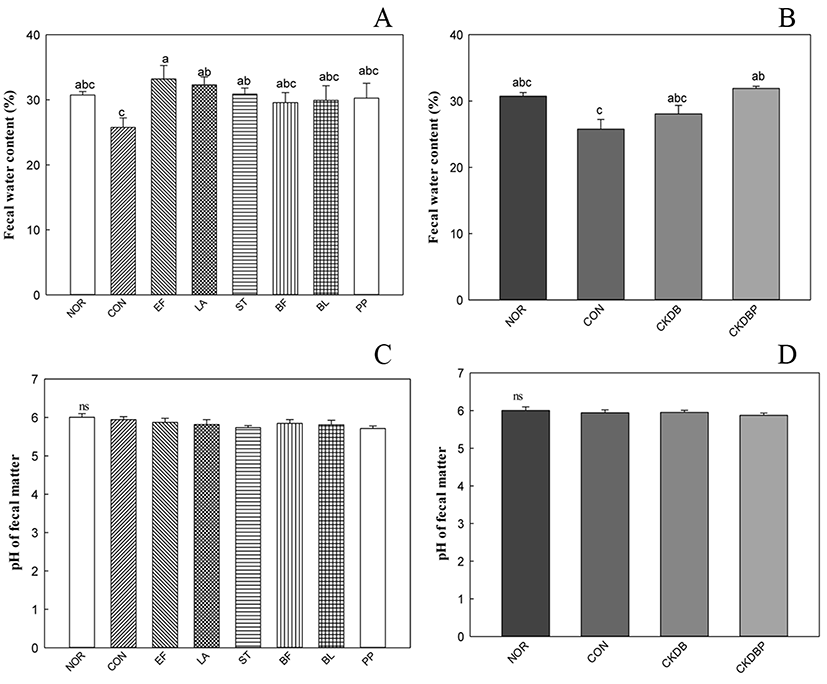
Administration of probiotic bacteria altered the fecal quality in rodents. It is noteworthy that the water content of the feces increased, suggesting that probiotic bacteria may show constipation-alleviating effects (Jeong et al., 2017).
The weights of organs were checked after harvesting them to confirm toxicity and abnormality of probiotics. Each organ was collected on the day of sacrifice, and the weight of liver, heart, kidney, spleen, and cecum were calculated as the organ weight per 100 g of the body weight. The weight of each organ showed no significant difference (Table 1), similar to the results reported by Park et al. (2011).
NOR: normal group. CON: loperamide-treated group, EF-PP: E. faecium, L. acidophilus, S. thermophilus, B. bifidum, B. lactis, P. pentosaceus and loperamide-treated group; CKDB: mixture of five probiotics and loperamide-treated group; CKDBP: P. pentosaceus and CKDB and loperamide-treated group. Each probiotic was administered at the same final concentration of 108 CFU/mL per kg of body weight.
The total cholesterol, HDL-cholesterol, TG, LDL-cholesterol, and glucose concentrations were within the normal range (Table 2). The normal concentration of HDL-cholesterol in the blood ranges from 25.00 to 63.8 mg/dL, and the total cholesterol is 72.0 to 206.0 mg/dL, based on SD-rats of 4 to 13 weeks. In addition, the normal concentration of triglyceride is 23.0-110.6 mg/dL, and that of glucose is 153.14-254.89 mg/dL (Han et al., 2010, Lee et al., 2012). The total cholesterol level was significantly higher in the control group (68.80 mg/dL) than in the experimental group, and the lowest value was found in the CKDB group (57.00 mg/dl) (p<0.05).
NOR: normal group. CON: loperamide-treated group, EF-PP: E. faecium, L. acidophilus, S. thermophilus, B. bifidum, B. lactis, P. pentosaceus and loperamide-treated group; CKDB: mixture of five probiotics and loperamide-treated group; CKDBP: P. pentosaceus and CKDB and loperamidetreated group. Each probiotic was administered at the same final concentration of 108 CFU/mL per kg of body weight.
To facilitate the bowel movement, intestinal motility should be actively performed, which can be confirmed by the rate of movement of the digestive tract. The rate of digestive tract migration was calculated by dividing the distance traveled by the activated carbon by total length of the intestine extracted on the sacrificial day. The migration rate of the constipation-induced control group was conspicuously lower than that of the normal group, indicating severe constipation in the loperamide-treated control group. However, the groups treated with LAB showed significantly higher intestinal motility, as compared to the control group, suggesting that peristalsis of intestinal tract was relatively facilitated in the LAB-treated groups. CKDBP administration group, in particular, showed the highest migration rate of 50.45% (Fig. 3, p<0.05). It seemed that LAB administration in loperamide-treated mice play an important role in maintaining the intestinal sensory and motor function for gut health. Probiotics, such as Lactobacillus acidophilus and Bifidobacterium bifidum, have been reported to release neuromessengers to promote intestinal motility (Ge et al., 2017). The motility and transit time of the intestine is important for distinguishing chronic constipation from normal-transverse constipation, or between slowly-variable constipation (STC) and defecation or rectal evacuation disorders. Therefore, the results of increasing the motility of intestines by single strain or mixture of LAB are important for developing effective probiotics for constipation relief.
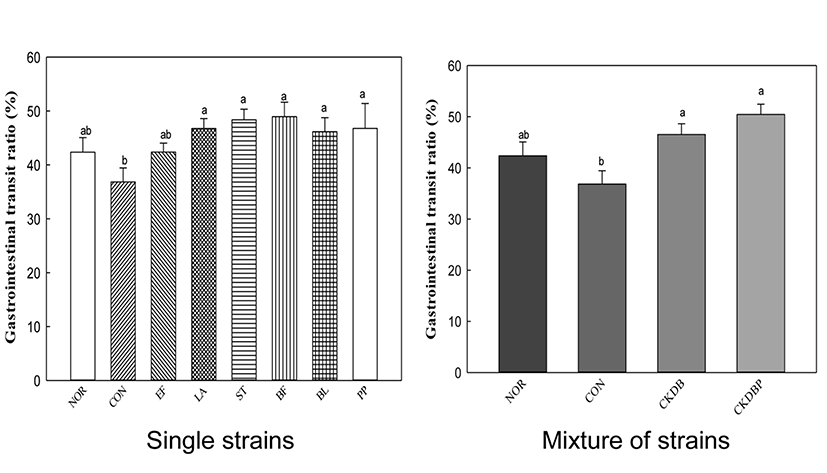
The longer the length of mucosa in the intestines, the higher the motility of the intestines (Jeon and Choi, 2010, Kim et al., 2015). Therefore, eosin H & E staining was performed to measure the length of the intestinal mucosa. The length of the control group (CON: 71.13 µm) was significantly lower than that of the normal group (NOR: 83.25 µm), and all groups treated with LAB showed significantly increased lengths, as compared to the control group (Fig. 4, p<0.05). These results are consistent with those reported by Kim et al. (2015), showing that LAB increase the length of intestinal mucosa. Especially, PP (88.93 µm), CKDB (91.10 µm) and CKDBP (89.11 µm) groups showed significantly longer lengths than the normal group.
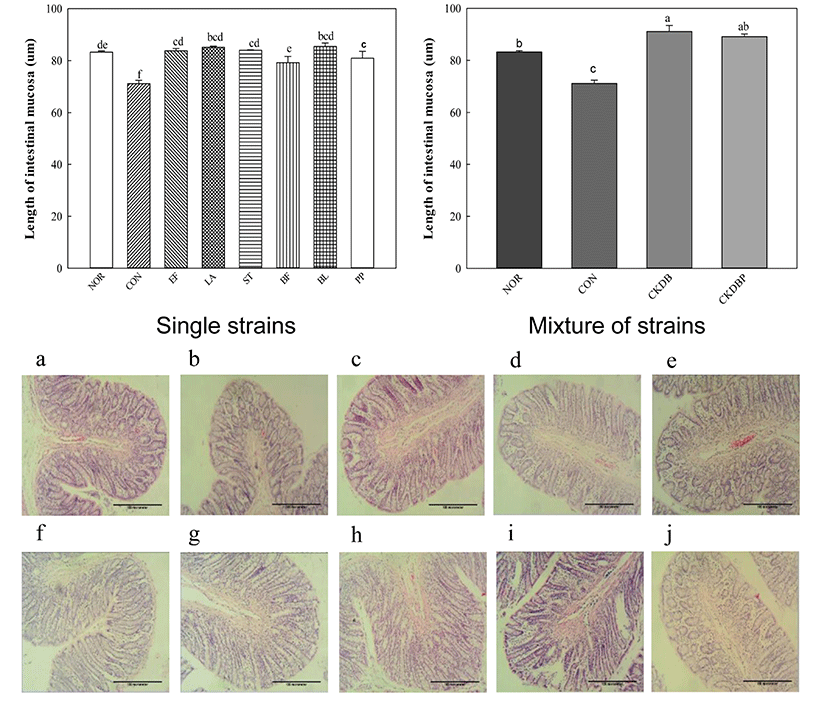
Alcian blue staining was performed to measure crypto cells that play a role in intestinal motility by regulating the secretion of intestinal mucus. The mucus layer overlying the intestinal epithelium is an important barrier against antigens and pathogenic bacteria (Ouwehand et al., 2002). The Alcian blue staining showed that mucus layer in the control group (CON: 3314.58 pixels) was significantly lower, as compared to that in the normal group (NOR: 53170.14 pixels), and the values of the group treated with the LAB were significantly higher than that of the control group (Fig. 5, p<0.05). In particular, the groups treated with individual LAB LA (53421.20 pixels), ST (52829.20 pixels), BL (53574.53 pixels), PP (50043.20 pixels) groups, and the mixture of strains, CKDB (49441.73 pixels) and CKDBP (5174433 pixels) showed much higher values than the control group.
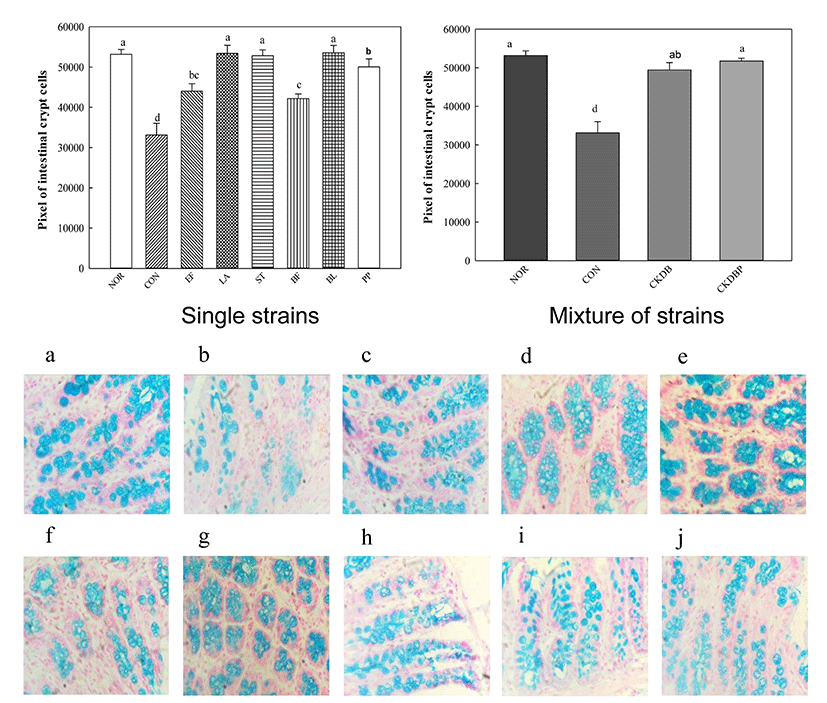
The interstitial cells of Cajal (ICC) are interstitial cells found in the gastrointestinal tract, which serve as electrical pacemakers and generate spontaneous electrical slow waves in the gastrointestinal (GI) tract. The greater the ICC number, the more the contraction and relaxation of the intestines (Hagger et al., 1997).
Immunohistochemically (IHC) staining was performed to analyze the interstitial cells of Cajal. The value of the control group (CON: 193006.73 pixels) was significantly lower than that of the normal group (NOR: 196334.58 pixels). In contrast, values of all the experimental groups fed with LAB were significantly higher, as compared to that of the control group (Fig. 6, p<0.05). CKDBP group (199396.44 pixels) showed the highest value among all the experimental groups.
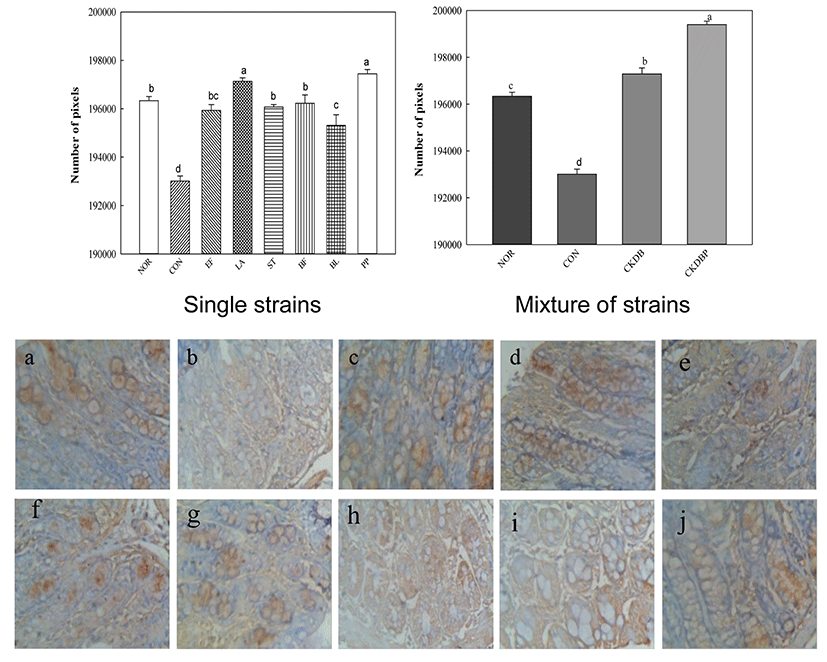
Constipation is reported to decrease mucus secretion in the intestinal mucosa. When loperamide is administered, mucin secretion is reduced due to decrease in the colon and intestinal peristalsis, consequently inhibiting movement of the stool contents (Hsieh, 2005). Therefore, the products tested in this study, especially those containing LA, CKDB, and CKDBP appear to substantially relieve constipation.
Higher the number of LAB in the intestines, greater the fermentation of food ingredients (such as carbohydrates) in the intestines, resulting in increased amounts of short chain fatty acids (SCFAs) in the feces. Therefore, GC analysis of feces in the cecum was performed to analyze the contents of SCFAs (Campbell et al., 1997). The acetic acid content in the feces of the cecum was lower in the constipation induced-control group (33.86 mM), as compared with the normal group (42.98 mM), but the contents in the LAB treated groups were significantly higher (p<0.05). The highest acetic acid content (52.26 mM) was found in the CKDBP group (Fig. 7, p<0.05). Propionic acid content was also lower in the constipation-induced control group (4.65 mM) than in the normal group (6.40 mM). However, the propionic acid concentrations were significantly higher, not only in the groups fed with individual lactic acid bacteria (EF: 7.27 mM, ST: 7.70 mM, BF: 7.03 mM, BL: 8.48 mM, and PP: 7.6 mM) but also in the group fed with mixture of the 6 bacterial strains (CKDBP: 7.87 mM) (p<0.05). Among these groups, BL and CKDBP groups showed the highest propionic acid content. The concentration of butyric acid in the control group (17.42 mM) was lower than those in the normal group (29.33 mM), the single strain EF group (29.22 mM), and the mixed strains CKDBP (27.87 mM) group. There was no significant difference in the valeric acid content among all the treated groups. Total SCFAs were calculated by sum of the concentrations of acetic acid, propionic acid, butyric acid, and valeric acid in each treated group. Total SCFA content in the constipation-induced control group (56.76 mM) was significantly lower than that in the normal group (80.01 mM). The groups treated with the LAB showed significantly high total SCFAs content (EF: 85.03 mM, BL: 79.08 mM, and CKDBP groups: 88.87 mM), as compared with the constipation-induced control group (56.76 mM) (p<0.05). In particular, the content was the highest in the CKDBP group. The concept of colonic health has become a target for the development of prebiotics, probiotics, and synbiotics. Increasing the production of SCFAs in the colon has been associated with reduced risk of some diseases, including constipation and cancer (Hooper et al., 2002). The individual LAB and mixture of strains used in this study seemed to provide constipation relief by increasing the short chain fatty acid secretion in the intestine of loperamide-induced constipated rats.
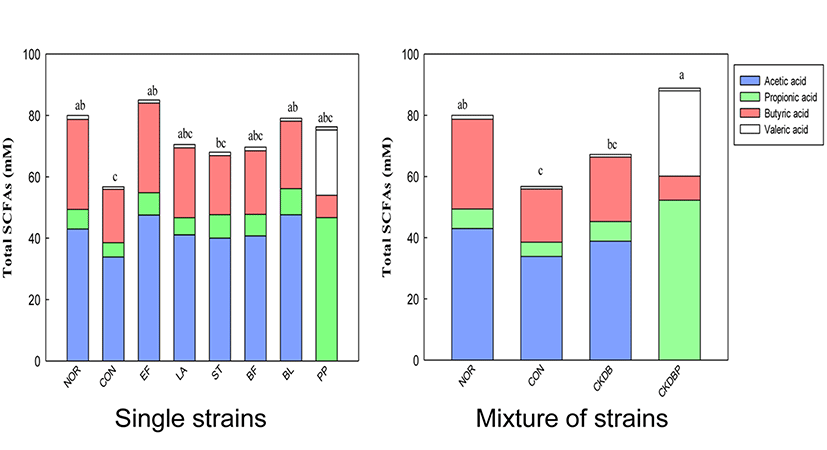
Microbiota present in the fecal mass in the cecum were analyzed using metagenomics. The results showed that distribution of Lactobacillaceae family containing the Lactobacillus species, which are useful bacteria, was higher in the lactic acid bacteria-treated groups than in the control group. Therefore, we analyzed three strains, constituting more than 5% of the microbiota (Lactobacillus intestinalis, Lactobacillus HQ768009_s, Lactobacillus reuteri). Analysis of the ratio of the three species in each group revealed that the ratio in the control group was considerably lower (11.11%) than that in the normal group (56.86%). All the LAB-treated groups showed a higher ratio than the control group. The ratios in PP (61.94%), CKDB (60.31%), and CKDBP (51.94%) groups, in particular, increased to the level of the normal group (56.86%) (Fig. 8).
The initial pH of milk (6.70 at 0 h) decreased to 4.43 for culture CKDB and to 4.41 for CKDBP at 21 h. The decrease in pH of 0.17 units from the set pH of 4.5 was obviously due to continued fermentation during overnight cooling till the temperature of the product reached 4°C. The pH dropped sharply until 9 h fermentation, and gradually decreased after 9 h. The trend was identical for all the starter cultures, similar to that observed for TA (Fig. 9A).
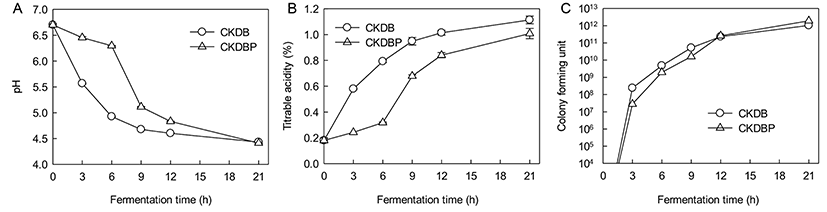
Fig. 9B shows an increase in titratable acidities of fermented milks with starter cultures of CKDB and CKDBP after 21 h. The initial TA of milk (0.18%) increased to 1.13 and 1.00% in fermented milks made from cultures CKDB and CKDBP after 21 h, respectively. Starter bacterial numbers in CKDB fermented milk were higher than those in CKDBP fermented milk following 12 h of fermentation After 12 h of fermentation, the bacterial count of CKDBP fermented milk was higher than that of CKDB (Fig. 9C). Thus, the amount of concentrated graph juice (CGJ) used in the fermented milk may be critical for the culture growth. Also, high osmotic pressure and high amounts of glucose in the medium (substrate inhibition) may cause loss of viability (Ramaswamy and Basak, 1992). No yeast or molds were detected in any of the fermented milk samples in these trials. On manufacturing fermented milk using CKDB and CKDBP starter, CKDB showed better fermentation characteristics than CKDBP during early stages of fermentation. However, CKDBP showed better fermentation characteristics than CKDB in the later stages of fermentation. For production of functional fermented milk, a recent trend is to use a mixture of 5 to 6 different strains of probiotic organisms for their potential health benefits, in addition to two starter bacterial cultures of S. thermophilus and L. delbrueckii subsp. bulgaricus. B. bifidum, B. breve, B. longum and B. animalis are commonly used to produce these fermented milks with other LAB. B. animalis lactis added in fermented milk fermentation has been found to survive significantly during passage through the gastrointestinal tract, and could be attached to enterocytes (Haschke et al., 1998). Therefore, fermented milk is used as an efficient carrier of probiotic bacteria. In this respect, addition of CKDB or CKDBP to starter bacterial cultures of fermented milk may be an effective means to supplementing these probiotics for potential health benefits.
Conclusion
In conclusion, probiotic bacteria are functional microorganisms that affect the gastrointestinal function. This study showed the potential of various individual LAB and a mixture of these strains for commercial enhanced gastrointestinal transit and alleviated constipation in loperamide-treated rats, by increasing stool excretion frequency, increasing stool water content, and reducing the remnant stools in the colon. Moreover, we observed from histopathological evaluation that mucus in the epithelial cells of loperamide-treated rats increased on supplementing with probiotic bacteria, particularly, CKDBP supplemented with six strains. Treatment with CKDBP, associated with stabilizing the mucosal barrier of the intestine, may be beneficial both in protection of the intact epithelium and in the treatment of the obstructed mucosal barrier. Consistent with this idea, our results show that the probiotic bacterial mixture, CKDBP, alleviates the symptoms of loperamide-induced constipation. Therefore, PPB is easy to use and can be recommended as a cost-effective option for constipation treatment.