Introduction
5-Hydroxytryptophan (5-HTP) is synthesized from the amino acid tryptophan. The body absorbs tryptophan, converts it to 5-HTP, and then generates serotonin, both centrally nervous and peripherally nervous system (Goldman-Rakic and Brown, 1981). Both tryptophan and 5-HTP can penetrate the blood-brain barrier. 5-HTP is a natural amino acid and biosynthetic intermediate of the neurotransmitter serotonin. The body absorbs tryptophan, converts it to 5-HTP, and then converts 5-HTP to serotonin (Haleem, 2017). 5-HTP functions as a neurotransmitter in dopamine and noradrenergic neurons (Haleem, 2017) and can alleviate a state of awakening (Ursin, 1976); thus, 5-HTP has a sleep-enhancing effect (Ursin, 2002). Insomnia induced by p-chlorophenylalanine was restored by 5-HTP, which can restore sleep by accelerating serotonin synthesis (Zhao et al., 2004). This p-chlorophenylalanine insomnia model demonstrates the importance of 5-HTP and serotonin in sleep regulation (Smith and Kennedy, 2003). However, the clinical use of hypnotics often has many side effects, such as drug dependence, tolerance, rebound insomnia, and other side effects. Over the past several decades, there has been an increased interest in the use of complementary and alternative medicine for insomnia to avoid these side effects (Sarris and Byrne, 2011). Natural sleep alternative supplements are increasingly used worldwide because of their safety and efficacy (Gyllenhaal et al., 2000). Various natural supplements, including Valeriana officinalis, Humulus lupulus, and 5-HTP, are readily available (Yang et al., 2017). In addition, many researchers are interested in the sleep-promoting effects of natural supplements and their ingredients.
When the nutritional value and safety of protein foods or new protein components are established, their functional and biological properties are also examined (Richardson, 1977). The biological activity of enzyme hydrolysates has been widely investigated. The functional, nutritional, and biological properties of proteins can be modified by enzymatic hydrolysis (Yu et al., 2007), which can produce various small peptides and free amino acids (Quist et al., 2009). The biological activity of protein hydrolysates is related to a variety of factors, including protein sources and enzymes (Keohane et al., 1985).
Therefore, enzymatic hydrolysis of egg protein to produce value-added hydrolysates is very attractive for improving insomnia. The goal of this study was to develop a material containing a high content of 5-HTP using liquid egg white for possible applications of this neurotransmitter in sleep disorder therapy. To increase the 5-HTP content in liquid egg white, the liquid egg white hydrolysate (LEH) obtained from enzymatic hydrolysis was subjected to various treatments. We established enzymatic hydrolysis conditions to increase 5-HTP content based on evaluation of the 5-HTP and amino-nitrogen contents and degree of hydrolysis (DH) of the hydrolysate. The sleep-promoting effect of LEH with a high content of 5-HTP was also evaluated in a pentobarbital-induced sleep model.
Materials and Methods
ʟ-Leucine, 2,4,6-trinitrobenzenesulphonic acid (TNBS) and ficin were purchased from Sigma-Aldrich (USA). Alcalase, neutrase, flavourzyme, and protamex were purchased from Novozymes (Denmark). Collupulin was purchased from DSM Corp. (Netherlands). Table 1 shows the characteristics of the enzymes used to prepare the hydrolysate.
Egg white solution (30 mL of liquid egg white in 70 mL of distilled water) was pre-incubated at 45°C for 20 min before enzyme hydrolysis. Hydrolysis was carried out at pH 6.0 for 12 h, and the enzyme reaction conditions are shown in Table 1. After hydrolysis, the enzyme was inactivated by heat treatment at 90°C for 15 min. The hydrolysate was centrifuged at 2,800×g for 20 min in a refrigerated centrifuge (Beckman model J2-21, Beckman Coulter, Inc., USA) and the supernatant was lyophilized (TFD, Ilshin, Korea).
A-N content was measured as described by Benjakul and Morrissey (1997). After adding 2.0 mL of 0.2125 M phosphate buffer (pH 8.2) and 1.0 mL of 0.01% TNBS solution to the diluted hydrolysate (125 µL), the solution was mixed thoroughly and incubated in a dark room for 30 min in a 50°C water bath (Model W350, Memmert, Germany). The reaction was terminated by the addition of 2.0 mL of 0.1 M sodium sulfite. After cooling the mixture at 22±0.5°C for 15 min, the absorbance was measured at 420 nm and the A-N content was expressed as L-leucine. The DH was calculated as follows (Benjakul and Morrissey, 1997):
DH = [(Lt − Lo)/(Lmax − Lo)] × 100
where Lt is the amount of A-N released at time t, Lo is the A-N content in the original liquid egg white, And Lmax is the total A-N content in the original liquid egg white obtained after acid hydrolysis at 100°C for 24 h with 6 M HCl.
Animal experiments were conducted with the approval of Korea University Animal Care Committee (KUACUC-20160824-1). Animals used in the experiment (4-wk-old ICR mice) were obtained from Central Lab Animal, Inc. (Korea). Rodents freely consumed food and water, and the animal breeding room was maintained at 24°C, 50-60% atmospheric humidity, and a 12-h light/dark cycle. Mice were tested for pentobarbital-induced sleep after 5 d of adaptation.
Before the pentobarbital-induced sleep experiment, mice were fasted for 24 h and all samples were dissolved in physiological saline. Sleep latency and duration were assessed following oral administration of 5-HTP (3, 6, and 9 mg/kg) or LEH (The amount of 5-HTP equivalent to 6 mg/kg). The mice were injected with pentobarbital (42 mg/kg) 40 min after sample administration. Mice were individually housed, and sleep latency and duration were measured as described by Yang et al. (2013). Mice that did not sleep for 15 min after pentobarbital-injection were excluded from the experiment.
All data were analyzed by using the Statistical Package for Social Sciences version 12.0 (SPSS Inc., USA). The results are presented as the mean±standard deviation. Differences between groups were evaluated by one-way analysis of variance and Duncan’s multiple range tests. Statistic values of p<0.05 were considered significant.
Results and Discussion
The aim of this study was to optimize the conditions to increase 5-HTP content by hydrolyzing liquid egg white. Enzymatic hydrolysis was monitored by measuring 5-HTP and A-N contents and DH. Alcalase hydrolysis yielded the highest content of 5-HTP (13.50 µg/mL), while neutrase treatment showed the lowest 5-HTP content (5.23 µg/mL) (Fig. 1). While non-enzymatic hydrolysis (control) showed a low level of 5-HTP (6.25 µg/mL), treatment with protamex, flavourzyme, collupulin, and ficin gave 5-HTP contents from 6.89 to 12.5 µg/mL.
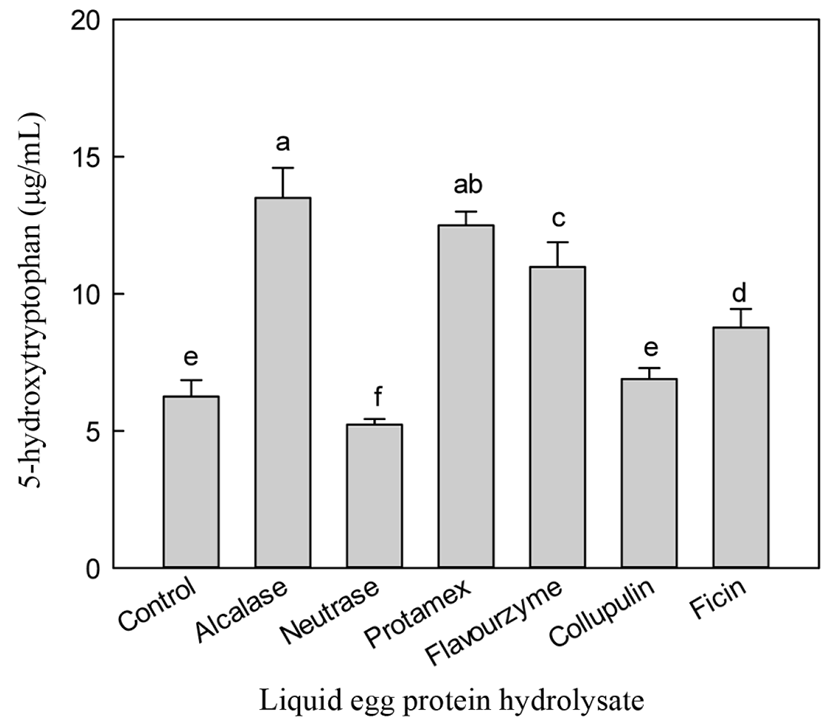
Alcalase hydrolysis yielded the highest A-N (15.27 mg/mL) content, while the control showed the lowest A-N (1.53 mg/mL) content (Fig. 2). Hydrolysis using neutrase, collupulin, papain, and ficin resulted in A-N contents of 1.72-7.86 mg/mL. Unlike the results for liquid egg white hydrolysis, the neutrase hydrolysate of egg white powder showed the highest A-N content (Cho et al., 2014). During the production of egg white powder, egg white proteins undergo thermal, physical, interfacial, and chemical treatments that can cause functional damage (Mine, 1995). Lechevalier et al. (2005) reported that heat treatment affected the tertiary structure of proteins, such as protein folding or aggregation. Therefore, liquid egg white and powder showed differences in the specificity of proteolytic cleavage.
DH is the most widely used indicator of proteolytic degradation in hydrolysis processes. Therefore, DH was measured to estimate the degradation extent of liquid egg white. DH values of hydrolysates were 0-43.2% after 24 h hydrolysis (Fig. 2). Alcalase showed the highest DH value (43.2%), which was more efficient in hydrolyzing liquid egg white hydrolysis compared to the other enzymes tested.
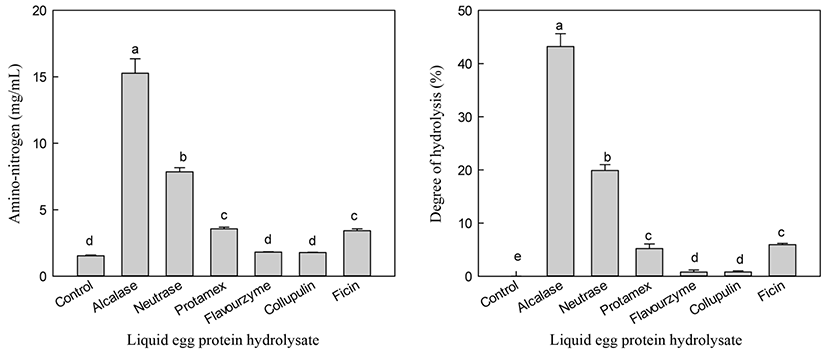
Alcalase showed a high degree of DH, which may be because alcalase contains an endo-protease that enhances the hydrolysis of liquid egg white. For enzymes showing a high DH, to the likelihood of obtaining egg white proteins composed of amino acids or short peptides is also high. Therefore, alcalase is ideal for commercial application because of its low cost and high recovery of A-N. Based on our results, alcalase is suitable for the production of LEH containing high 5-HTP content.
Enzyme specificity, enzymatic hydrolysis conditions, and substrate concentration used in proteolysis greatly affect the molecular weight and amino acid sequence of the hydrolysates and their biological activities (van der Ven et al., 2002). Taking into account the economic considerations in protein recovery from liquid egg white, various substrate concentrations were compared to determine the most suitable concentration for substrate hydrolysis.
Fig. 3 shows the changes in 5-HTP according at various substrate concentrations. A substrate to water ratio of 1:2 showed higher production of 5-HTP compared to other ratios such as 2:1. However, there were no significant differences in 5-HTP content between the 1:2 and 1:1 ratios of egg-white and water.
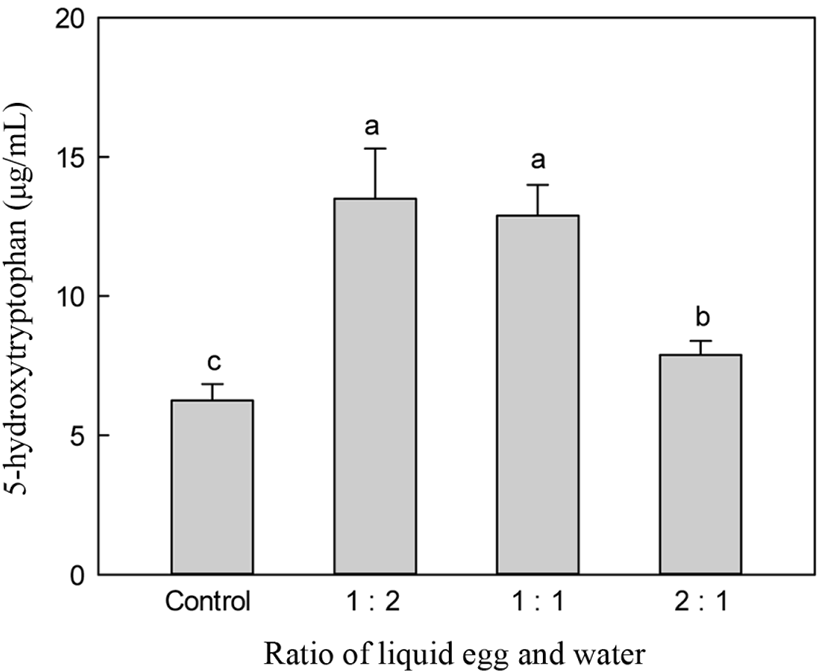
Fig. 4 shows the changes in A-N content and DH at various substrate concentrations. A substrate to water ratio of 1:1 showed the highest A-N content among other ratios, such as an egg white liquid to water ratio of 1:2 and 2:1. The 1:2 ratio was not significantly different from the A-N content obtained using a 1:1 ratio. Increasing the water addition to liquid egg white increased DH. However, the costs of manufacturing the desired bioactive peptide are an important consideration. To minimize the energy cost of water removal to dry hydrolysate and reduce the reaction time, the liquid egg white ratio for alcalase was a substrate to water ratio of 1:1. A high concentration of substrate is very important for decreasing production costs.
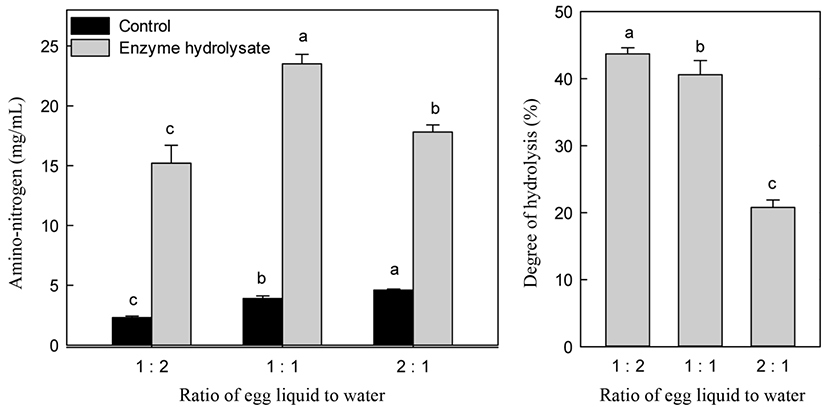
5-HTP and A-N contents and DH of alcalase hydrolysate were increased with increasing enzyme addition (Figs. 5 and 6). 5-HTP, A-N, and DH increased until 24 h of hydrolysis and then slightly increased thereafter during hydrolysis with 2% and 5% enzyme addition. Significant changes in 5-HTP, A-N, and DH were observed following enzyme treatment at 2% and 5% addition between 19.19 and 24.84 µg/mL, 27.88 and 34.63 mg/mL, and 42.30 and 51.80% at 24 h hydrolysis, respectively. Particularly, 5% alcalase addition did not significantly increase the 5-HTP, DH, and A-N contents after 24 h of hydrolysis (Figs. 5 and 6).
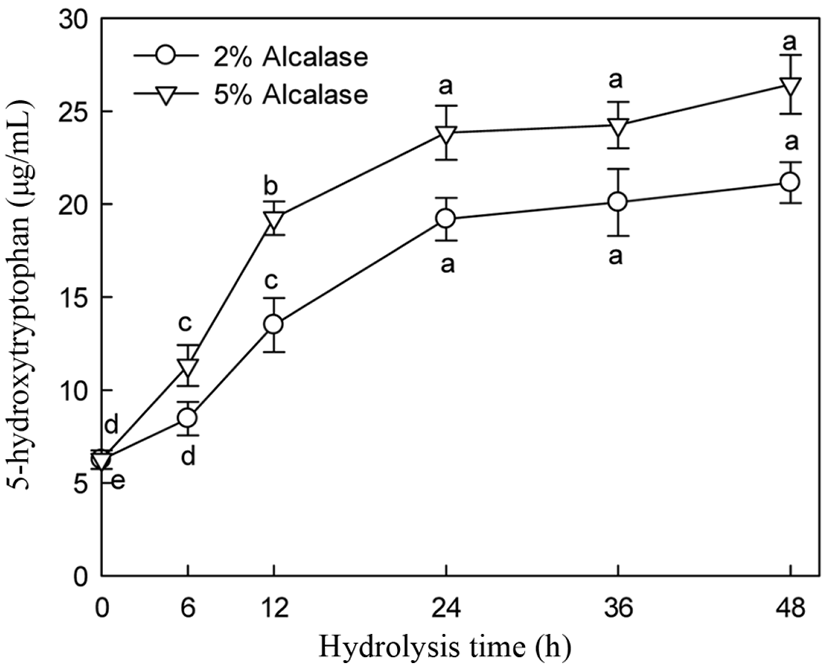
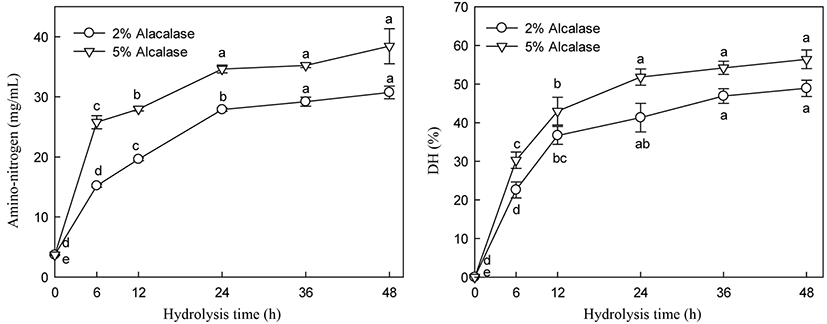
Many researchers have observed similar trends in hydrolysis using various proteases and substrates (Lopez et al., 1998, Qi et al., 1997). The hydrolysis pattern, particularly the decrease in hydrolysis rate, is not fully understood. Alder-Nissen (1986) found that changes in hydrolysis rate are not attributed to substrate exhaustion. Constantinides and Adu-Amankwa (1980) suggested several explanations, such as the depletion of peptide bonds that enzyme intends to hydrolysis, product inhibition, and/or enzyme inactivation.
The ratio of enzyme to substrates suitable for hydrolysis was 2-10: 100 (w/w) according to the recommendations of most suppliers (Gibbs et al., 2004). Other studies showed that a range of 1.00-3.00% is an economically feasible enzyme range, with DH increasing as the E/S ratio is increased up to approximately 2.40%, with slight decreases at higher E/S ratios (Wu et al., 2008).
Pentobarbital sodium, a barbiturate compound, is used to induce sleep in both rodents and humans (Koch-Weser and Greenblatt, 1974). Pentobarbital-induced sleep in mice is the most commonly used method of screening of sedative-hypnotic drugs. Therefore, we used this method to evaluate the sleep-potentiating effects of 5-HTP and LEH.
5-HTP and LEH were administered 40 min before the pentobarbital-induced sleeping test. The 5-HTP and control groups showed differences in the sleep induction effect (Fig. 7). Oral administration of 5-HTP (3, 6, 9 mg/kg) generally increased the sleep duration and decreased the sleep latency time in pentobarbital-induced mice. Particularly, 5-HTP administration of 6 and 9 mg/kg significantly increased sleep duration and decreased sleep latency time compared to that in control mice (p<0.05). However, 5-HTP administration at doses of 6 and 9 mg/kg did not result in significant differences in sleep latency and sleep duration time.
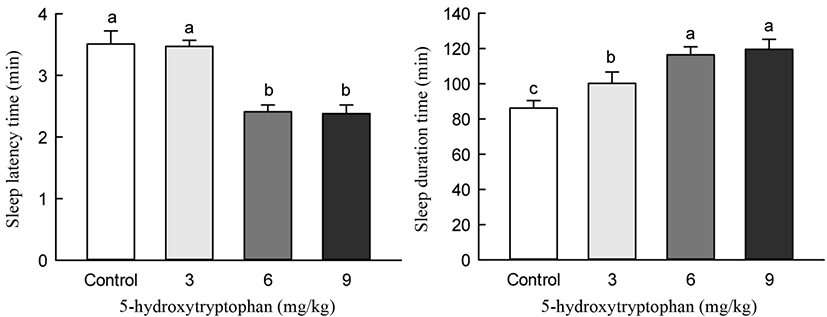
We also compared the sleep-promoting effect of 5-HTP (6 mg/kg) and LEH (150 mg/mouse), which was equivalent to 5-HTP at 6 mg/kg for sleep latency and sleep duration in mice treated with pentobarbital (42 mg/kg, i.p.). 5-HTP and LEH significantly decreased the sleep latency time compared to that in control mice (p<0.05). There were no significant differences in sleep latency time between 5-HTP and LEH. 5-HTP and LEH also significantly increased the sleep duration time compared to that in control mice (p<0.05). There was a significant difference in sleep duration time between mice treated with 5-HTP and LEH (p<0.05). These results demonstrate that oral administration of LEH had sleep-promoting effects. The sleep-promoting effect of LEH was caused by 5-HTP.
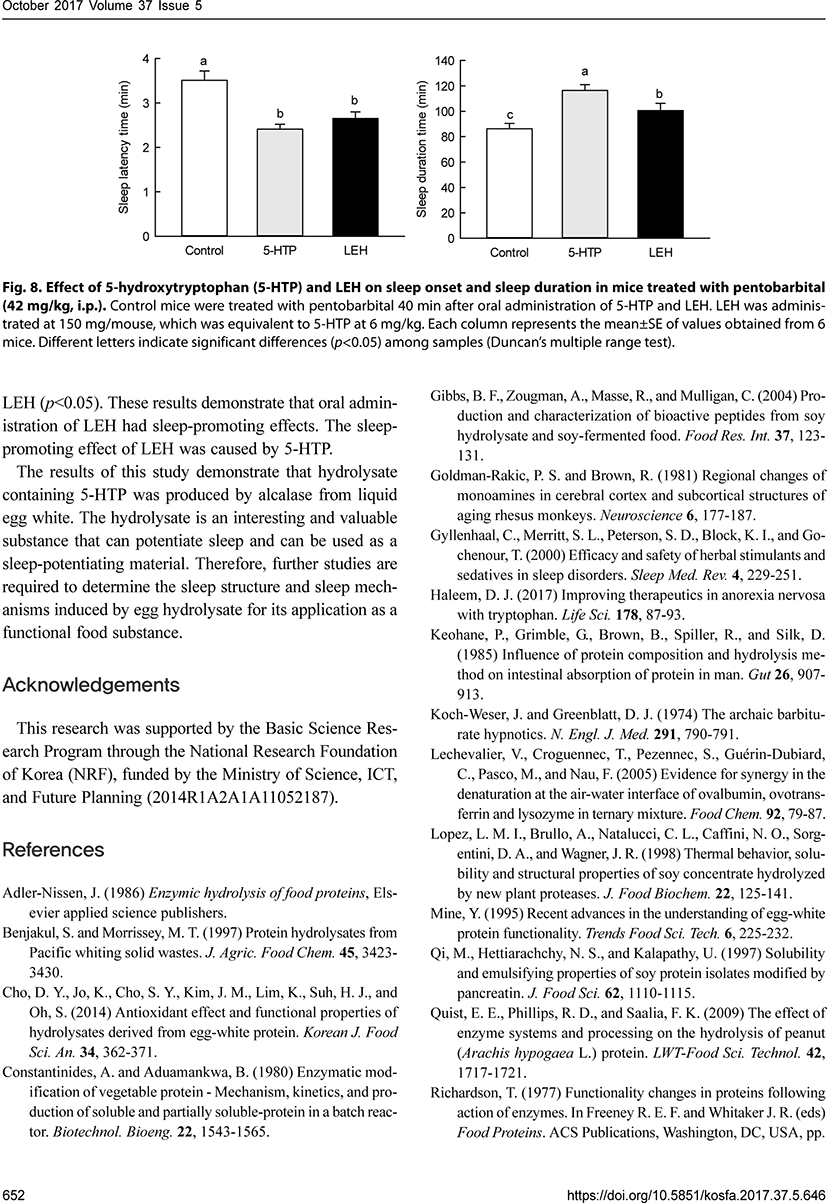
The results of this study demonstrate that hydrolysate containing 5-HTP was produced by alcalase from liquid egg white. The hydrolysate is an interesting and valuable substance that can potentiate sleep and can be used as a sleep-potentiating material. Therefore, further studies are required to determine the sleep structure and sleep mechanisms induced by egg hydrolysate for its application as a functional food substance.