Introduction
Lipid oxidation is an important factor limiting the quality and acceptability of meat products. It has currently become one of the greatest economic problems in the meat industry (Králová, 2015). Particularly, ground meat is one of the most susceptible meat products for lipid peroxidation and microbial contamination during processing and storage (Ledward and Macfarlane, 1971). Synthetic antioxidants such as butylated hydroxyanisole (BHA) and butylated hydroxytoluene (BHT) have been widely used for many years owing to their chemical stabilities, strong antioxidant activities, and lower costs (Verhagen et al., 1994). However, due to the potential carcinogenic properties of synthetic antioxidants (Imaida et al., 1983; Kahl, 1984), many researchers are devoted to replacing them with natural substances, derived from animals, plants, or microbes, which can be efficient, non-toxic, and safe for consumer health (Agnieszka et al., 2016).
In the fish production process, more than 60% by-product including head, skin, bones, fins, viscera, and roe are discarded without attempts for use (Chalamaiah et al., 2012). Conversion of these waste materials into value-added functional materials is a promising area of food industry. Among them, the discarded fish skin is a favorable source to extract functional proteins and peptides (Himaya and Kim, 2013). Tuna is one of the top five most-consumed seafood species in the world. Antioxidant, antimicrobial, and antitumor activities could be detected in tuna skin peptide (Gómez-Guillén et al., 2010; Han et al., 2011). However, only few studies have investigated the inhibition of lipid oxidation in raw ground meat by fish skin peptide (FSP), a marine by-product and natural antioxidant. Therefore, this study was focused on the preparation of collagen peptide from tuna (Thunnus obesus) skin and the application of this peptide as a biopreservative in a pork patty model.
Moreover, stability issues including proteolytic degradation and the potential interaction of the functional peptide with food components might result in decreased bioactivity (Malheiros et al., 2010). Bitter taste and fishy smell are also the crucial limiting factor in use of those add-value fish by-products (Benjakul et al., 2014). The encapsulation of the peptides into liposomes represents an alternative to overcome those problems related to the direct application of these functional peptides in foods. Liposome technology is one of the most recent nanoencapsulation techniques. Liposomes are artificial vesicles formed by one or more concentric lipid bilayers separated by water compartments (Munin and Edwards-Lévy, 2011). Due to the possession of both lipid and aqueous phases, liposomes can be utilized in the entrapment, delivery, and release of water-soluble, lipid-soluble, and amphiphilic materials as well as contain targetability (Mozafari et al., 2008). Currently, the encapsulation of fish oil in nanoliposomes has been well-studied; for example, Ghorbanzade et al (2017) reported that yogurt fortified with encapsulated fish oil liposome had higher DHA and EPA contents than yogurt containing free fish oil.
Therefore, in this study, we aimed to evaluate the effects of FSP and FSP-loaded liposomes (FSPL) on lipid oxidation, color deterioration, and other sensory properties of ground pork patties. This research studied the possibility of FSP as a preservative for pork patties and liposome encapsulation as a vehicle for entrapping fishy smell, and provides a theory and practice basis for the application of FSP(L) in meat products.
Materials and Methods
To make the pork patties, Korean native fresh pork ham (mixed with Musculus biceps femoris, M. semitendinosus, M. semimembranosus, M. gracilis, and M. adductor) and back fat (from longissimus dorsi) were purchased from a local processor at 48 h postmortem. All visible fat and connective tissue was trimmed off, and they were frozen at −18°C until further use. Food grade NaCl (Daesang, Korea) was also used for the patty process. Lecithin (Lipoids GmbH., Switzerland) was used as a liposome wall material. Butylated hydroxyanisole (BHA; Sigma-Aldrich, USA) was used as a positive control. Trichloroacetic acid (TCA; Samchum, Korea), 2-thiobarbituric acid (TBA; Sigma-aldrich, USA), and 1 N-hydrochloric acid (HCl; Duksan, Korea) were used for the TBA analysis. Potassium carbonate (K2CO3; Samchum, Korea), boric acid (H3BO3; Showa, Japan), sulfuric acid (H2SO4; Matsunoen, Japan), methyl red (Janssen, Korea), and bromocresol green (Daejung, Korea) were used for the VBN analysis.
Tuna (Thunnus obesus) fish skin was purchased from a local company (TUNA Factory, Korea). The fish skin was washed three times, and the fish scales and visible fats were removed. The fish skin was cut into 3 cm squares and pulverized in distilled water for 3 min using CNHR-26 four-wing blade blender (Bosch, Hong Kong). Finally, the pulverized fish skin was homogenized at 22,000 rpm for 1 min using a T25 Ultra Turrax® homogenizer (IKA Labotechnik, Germany). Approximately 100 g of the fish skin mixture (67% final solid contents) was vacuum-packaged and frozen at −80°C until use.
Pretreated fish skin was hydrothermally hydrolyzed following a modified method based on Min et al. (2017). The fish skin mixture was suspended in distilled water to a final concentration of 6.7% for hydrothermal hydrolysis. The hydrothermal processing system used in this study was a custom-designed, laboratory-scale, high-temperature, moderate-pressure processing reactor (capacity, 1 L; maximum temperature, 400°C; max pressure, 300 bar; R 101, Rexo Engineering, Korea). In this system, the pressure chamber was heated to 190°C using a movable electric heater, and the pressure reached 1,100 kPa. The target temperature and pressure were maintained for 10 min (holding time) for protein hydrolysis. The movable electric heater was then vertically removed from the pressure chamber, and a cooling jacket with a cryostat (FP-80, Julabo, Germany) was automatically set to 0°C. After the high-temperature, moderate-pressure treatment, the sample was immediately removed from the pressure chamber and cooled to 0°C using ice water. The resultant sample was centrifuged at 6,000 rpm for 15 min. After centrifugation, the supernatant (fish skin collagen hydrolysate) was lyophilized and stored at −80°C until use.
After 12% (w/w) FSP solution was mixed with 1% (w/w) lecithin, the mixture was homogenized by using T25 digital Ultra-turrax high-speed mixer (IKA, Germany) at 8,000 rpm for 3 min. Sonication was, then, used to enhance the reduction of liposome size using an ultrasonicator (Bandelin, Germany) at 60 W for 3 min. The FSPL solution was lyophilized for further study.
Lean materials and pork back fat were minced using a PM-70 mincer (Mainca, Spain). Ground meat was prepared by 80/20 lean-to-fat ratio, mixed with 1.5 wt% of salt (Chun et al., 2014). Four major formulations of patties were prepared on the basis of the ground meat: (A) ground meat without FSP(L) (control); (B) ground meat containing 0.02% (w/w) BHA as positive control; (C) ground meat with different FSP concentrations [0.1%, 0.5%, 1%, 2%, and 3% (w/w)]; and (D) ground meat with different FSPL concentrations (0.1%, 0.5%, 1%, 2%, and 3% (w/w)). Each meat sample was thoroughly mixed in a homomixer (5K5SS, Kitchen Aid, USA). Approximately 85 g of meat batter was molded in a petri dish (90 mm diameter × 15 mm height) to form patties. Then, the patties were wrapped with polyethylene film and kept at 4°C for 14 d. Pork patty processing was run in triplicate for each treatment.
Lipid oxidation, an important factor involved in meat rancidity, was quantified by the thiobarbituric acid reactive substances (TBARS) assay following a modified method based on Witte et al. (1970). The results were expressed as malondialdehyde (MDA) equivalents per kilogram meat (mg MDA/kg meat). A 5 g sample was diluted in 45 mL distilled water and blended for 60 s on high speed. The mixture was filtered through Whatman No.1 filter paper. The filtrate (0.5 mL) was transferred to a test tube and mixed with 4.5 mL of TBA solution (0.25 N HCl, 15% TCA, and 0.375% TBA reagent). The filtrate solution was incubated in BF-30SB water bath (BioFree, Korea) at 95°C for 15 min, and centrifuged at 5,700×g, 4°C for 10 min. Finally, the absorbance at 535 nm was measured using Multiskan™ GO UV/Vis microplate spectrophotometer (Thermo Fisher, USA).
Color parameters were determined using CR-400 Chroma Meter (Konica Minolta sensing, Japan) calibrated with a white standard plate (CIE L*=+96.79, CIE a*=+0.30, CIE b*=+1.67). The surface color of pork patties was measured 10 times. The results were expressed as lightness (CIE L*), redness (a*), and yellowness (b*) values. Total color difference (ΔE) was calculated as follows:
A 3-cm-diameter and 1-cm-height sample (approximately 8 g) removed from the pork patties was placed in a vacuum-sealed bag, cooked for 30 min at 75°C in BF-30 SB water bath (BioFree), and cooled to room temperature. Cooking loss of the patty was expressed as a percentage of the initial weight as follows:
For the texture analysis, the cylindrical forms of the cooked patties were cut into 20 × 10 × 10 (length × width × height) mm cuboid shapes. Hardness was measured by CT3 texture analyzer (Brookfield Engineering Labs, USA), equipped with a TA3/100 probe and TA/SBA fixture, using the following conditions: 2 mm/s test speed, 5 g trigger load, and 30% compression.
VBN content is an important index of pork’s freshness and was determined by Conway’s micro-diffusion method (Conway, 1950). Briefly, a 5 g sample was homogenized with 20 mL of distilled water in a BagFilter P (Interscience, France) using a stomacher (WS-400, Hansol Tech, Korea). The homogenate was filtered through Whatman No. 1 filter paper. The filtrate (1 mL) was transferred to the outer chamber, and 1 mL of 0.01 N H3BO3 and 100 µL of the Conway solution (0.066% methyl red and 0.066% bromocresol green in 99.99% alcohol), an indicator, were added to the inner chamber of the Conway dish. The Conway unit was sealed immediately after the addition of 1 mL of 50% K2CO3 to the outer chamber. Then, the materials were incubated at 37°C for 2 h. The VBN contents were determined following the addition of 0.02 N H2SO4 to the inner chamber of the Conway unit. A blank test was conducted following the same process but excluding the sample filtrate.
where a = titer for sample (mL), b = titer for blank (mL), f = factor of H2SO4 (0.02 N), S = sample weight (g), and c = dilution ration.
A 5 g sample was diluted with 45 mL distilled water, blended using a homogenizer (PH91, SMT, Japan) for 60 s, and filtered by a BagFilter P. The pH value of the filtrated solution was determined using a pH meter (OrionTM 4-Star Plus pH meter, Thermo Scientific, USA).
All treatments in the present study were performed in at least 3 replicates (n>3). Data were expressed as the means with standard deviations. One-way analysis of variance (ANOVA) was performed and the mean comparisons were done by Duncan’s multiple range test. Differences were considered significant at the level of p<0.05. Statistical analysis was performed using the software SPSS 22.0 (SPCC Inc., USA).
Results and Discussion
Fig. 1A shows the TBARS values of pork patties containing FSP at 4°C during a 14 d storage trial. The TBARS value, an index of oxidative stress, increased as the time of storage increased for all treatments. As expected, the TBARS values of all FSP-treated patties were significantly lower than those of control from day 4, which indicates a clear inhibitory effect of FSP on lipid oxidation with an amount dependent manner. Low concentrations of FSP may be insufficient to protect meat from lipid oxidation, therefore, the TBARS of the less FSP added treatment showed the closer to control group. Especially of note, the 3% FSP-treated patties exhibited similar TBARS values to BHA-treated patties from days 0 to 7 (p>0.05), but such protection is not as well as BHA for a long-term storage. The TBARS value of fresh meat or meat products is approximately 0.2 mg MDA/kg, organoleptic quality degradation begins when the TBARS value reaches 0.46 mg MDA/kg, and a complete corrupt state occurs when the value reaches 1.2 mg MDA/kg (Han et al., 2006). The USDA Food Safety and Inspection Service (FSIS) also recommends that fresh pork roast, steaks, chops or ribs can be stored for only 3 to 5 d at refrigerator temperature (4°C). Therefore, in the case of meat used within the quality guarantee period (approximately 7 d), FSP can be effective to protect meat by reducing the lipid oxidation, resulting in an extension of the shelf life of the product.
The TBARS value of pork patties containing FSPL was also assessed and is shown in Fig. 1B. Unexpectedly, the TBARS values of FSPL-treated patties were observed significantly higher compared to the control and increased rapidly with the increase in storage time or concentration (p<0.05). Phospholipids act as the major contributors to oxidative rancidity in lean meat (Cheng, 2016). Therefore, it is possible that the lecithin in the coating layer consists of phospholipids, which can initiate lipid oxidation. Even the TBARS values of FSPL-treated patties at day 0 were observed to be increased as a function of the liposome concentration. Moreover, in other studies, lecithin-liposome model system was used to evaluate the antioxidant activity of some antioxidants (Kong et al., 2010).
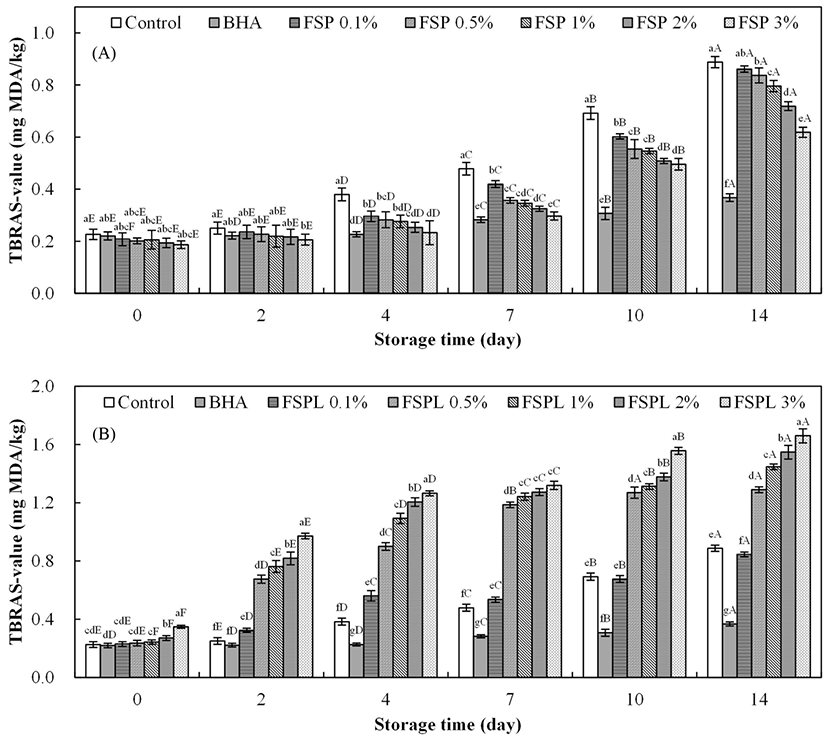
The changes of the meat surface CIE L*, CIE a*, CIE b*, and total color difference by FSP and FSPL treatments are given in Table 1A and 1B, respectively. In Table 1A, the CIE L* of all patties showed similar values at day 0 (p>0.05), but the values of FSP-treated patties were significantly lower than that of control and BHA at day 14. However, no significant differences were observed among the different concentration of FSP treatments at the same storage period (except day 7).
Lightness (CIE L*) was not affected by the concentration of FSP. In comparison, the CIE L* values of FSPL-treated (Table 1B) patties were higher than that of FSP-treated patties after 14 d storage.
1)Control, pork patty without additive; BHA, pork patty containing 0.02% (w/w) butylated hydroxyanisole as positive control; and FSP(L), pork patties containing different concentrations of FSP(L) (0.1%, 0.5%, 1%, 2%, and 3% (w/w)); FSPL, pork patties containing different concentrations of FSPL.
2)a-dMeans with different letters within the samples at the same storage time and A-EMeans with different letters within the same sample group for the entire experiment days are significantly different by Duncan’s range test (p<0.05).
The redness discoloration was observed in all FSP(L) patties over the storage period. Radicals generated by lipid oxidation can promote the accumulation of metmyoglobin (Faustman et al., 2010), which makes the meat color turn brown. A decreasing trend in CIE a* value have been found as the concentration of FSP increased at day 0. However, from day 2, redness value was proportional to FSP concentration. This could be due to the interference from the intrinsic FSP color at beginning, and then, FSP take into effect. The larger amount of FSP added, the effects of anti-lipid oxidation and red color protection were more evident. Reversely, the redness of all FSPL-treated patties showed a decreasing trend as the concentration of FSPL increased from day 2 with an exception of the 3% FSPL-treated patties at day 14. With the concentration increase of liposome, the larger amount of lecithin compound in pork patties might interfere with the antioxidant activity of FSP, as explained above.
The yellowness of all patties, including control, gradually increased as the storage time increased. The CIE b* values of the pork patties with different concentrations of FSP(L) showed no obvious regularity. Marked rancidity in meat is usually associated with a change in the color of fat, from white to yellow, by the oxidation reaction (David and Rorbert, 2014).
The initial values for total color difference (ΔE) of FSP-treated patties ranged from 2.27±0.54 to 3.04±1.20 and showed no significant difference among ttem. The ΔE values of all FSP-treated patties were higher than that of the control from day 2, but lower than the control on day 14. Correspondingly, the ΔE values of FSPL-treated patties showed a similar pattern to those of FSP-treated ones. ΔE values of FSP(L)-treated patties dynamically increased during 14 d storage at 4°C.
Cooking losses of the pork patty samples containing FSP and FSPL are presented in Fig. 2. The BHA treated samples had the lowest weight loss, but no significant difference between control and each of FSP(L)-treated patties was observed. While the patties with the higher concentration of FSPL showed lower cooking losses from day 2, the cooking loss values of different concentrations of FSPtreated patties were little changed after 14 d of storage. Patties with high concentrations of FSPL showed significantly lower cooking losses than BHA-treated patties. The greater cooking losses are a result of a lowered water holding capacity and are associated with less juiciness and tenderness of the products (Tan et al., 2006).
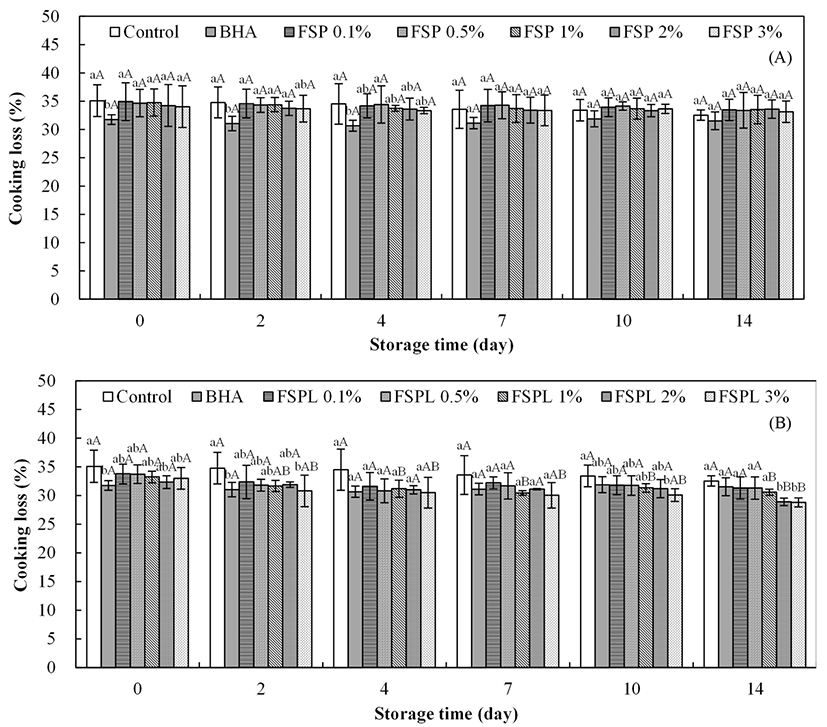
The results of the textural property of cooked pork patties are shown in Fig. 3. As storage time increases, the hardness value of the control patties were significantly increased, but there were no significant differences in the other treatments (BHA and different concentrations of FSP(L)-treated patties). This suggests that FSP(L), FSPL, BHA possess an inhibitory effect on hardness. These results are in general agreement with the report of Peng et al. (2016), which stated that 15% whey protein hydrolysate was effective on the maintenance of the gel texture and water-holding capacity of treated pork patties. Protein oxidation induces the increased hardness in pork patties through the loss of protein functionality (Xiong, 2000), and the formation of cross-links between proteins eventually leads to undesirable textural changes (Estevez et al., 2005; Karel et al., 1975). In Fig. 3A, BHA-treated patties had the lowest hardness values compared to all FSP-treated patties and control, and showed a significant difference from day 4 (p<0.05). FSP-treated patties showed a decreasing trend in hardness with an increase in FSP concentration, but no significant differences were found between them. The hardness values of FSPL-treated patties also revealed the same pattern as those of FSP-treated patties, but the trend was more clearly. FSPL-treated patties showed lower values compared to FSP-treated patties. This effect was presumably due to the controlled-release mechanism of the liposome system (Mwangi et al., 2016).
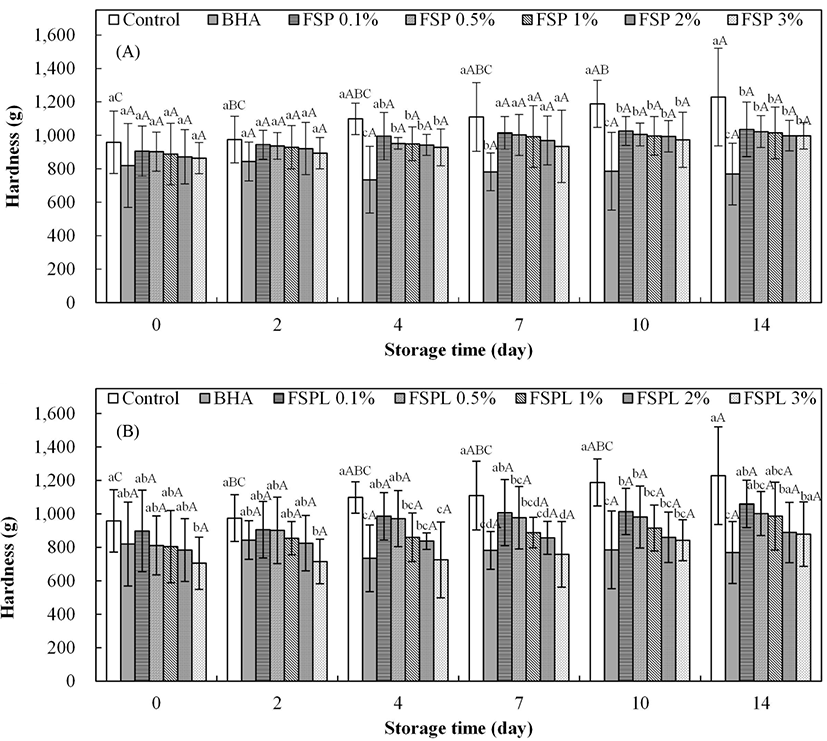
Kim and Shin (2011) reported that a peculiar smell developed when the VBN value was over 15 mg%. In this study, the VBN values of the control patties increased over time and ranged from 10.09 mg% to 13.45 mg% (Fig. 4A), but not beyond the standard range. Each concentration of FSP-treated patties showed a slightly increasing trend in the VBN values over time. At day 14, the VBN values were increased with the concentration of FSP increase, but no clear trends in the other storage time among different concentration of FSP. Most VBN values of the FSP-treated patties were higher compared to control patties during storage. These results agree with a previous report by Abu-Salem et al. (2014). In that study, they added the soybean peptide as a bioactive component in beef burgers and found that soybean additives increased the total VBN values at a significant level (p<0.05) compared to the control. The hydrolysis of protein can result in the accumulation of non-protein nitrogen (Yunus et al., 2001). Therefore, it is speculated that a peptide, one of non-protein nitrogen, might lead to an increase in nitrogen contents and VBN values when it was added to pork patties. On the other side, the VBN value of FSPL-treated patties increased as the concentration of FSPL increased (Fig. 4B). The difference in VBN values among various concentrations of FSPL-treated patties gradually decreased over time. No significant difference between control, BHA and FSPL treatments was observed on day 14.
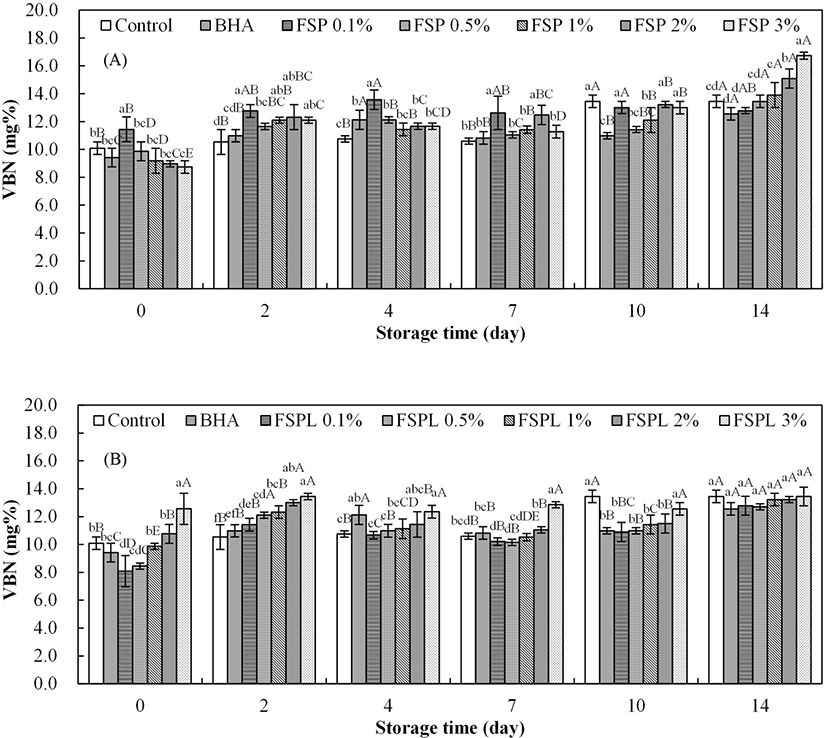
The effect of the different concentrations of FSP(L) on the pH of the patties is shown in Fig. 5. In Fig. 5A, the initial values for the pH of control and FSP-treated patties ranged from 5.60±0.01 to 5.64±0.01, with no significant difference among them (p>0.05). Wang et al. (2010) reported a similar result that the addition of housefly peptide had no effect on the pH value of chilled pork at the beginning of storage. The pH value of FSP-treated patties was slightly increased as the concentration of FSP increased, with an exception of rapid rise of the pH value at day 2. This may be due to the pH of the peptide (approximately 5.95), which might cause an increased pH in the FSP-treated patties. The finial pH values of FSP-treated patties were similar to those of the BHA treated patties and lower than that of control, with an exception of the 3% FSP-treated patties.
The pH value of FSPL-treated patties is shown in Fig. 5B. Counter to the pH results of FSP treatments, a decrease in pH values was observed with the amount of FSPL increase, with an exception of 3% FSPL-treated patties from day 4. This phenomenon was more clearly at day 2. Each concentration of FSPL-treated patties has remained fairly constant in pH value over time (p>0.05), except of day 2.
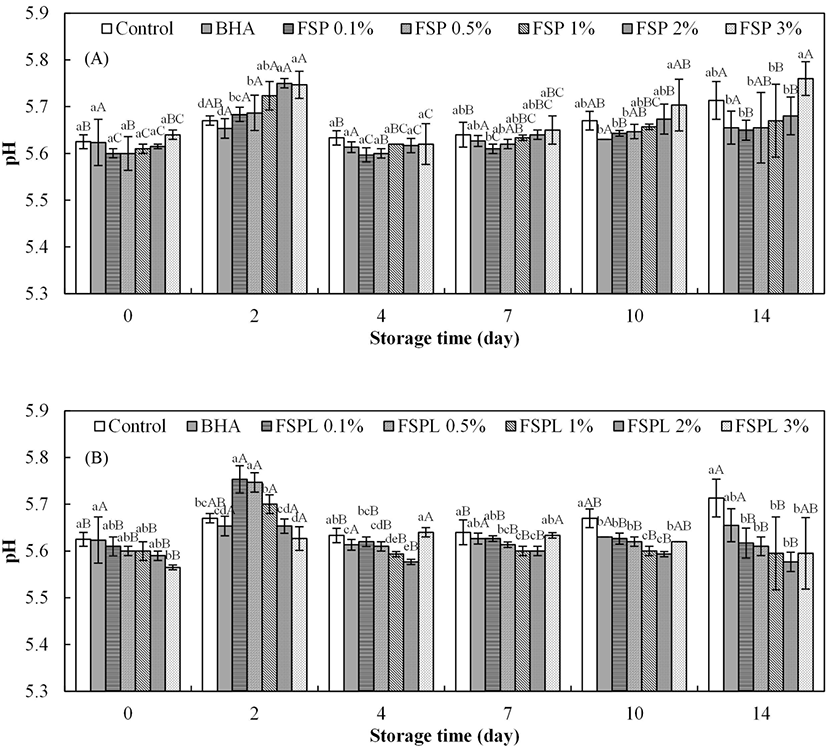
Conclusions
The present work evaluated the effect of FSP and its liposome form on the antioxidative and physicochemical properties of pork patties during 14 d of refrigerated storage. The results of the lipid oxidation analysis indicated that FSP was effective in the inhibition of pork patty fat oxidation for 7 d, and the most effective concentration of FSP was 3%, the highest concentration. It was also suggested that liposome made by lecithin was not a good candidate for FSP encapsulation, since the TBARS values increased dramatically as the concentration of FSPL treatment increased. FSP(L) had no positive effect on maintaining bright red color of the mince or the VBN value, but the addition of FSP could affect the yellowness and total color difference during refrigerated storage. Cooking loss was not influenced by the addition of FSP; however, the value of cooking loss was significantly decreased by more than 2% FSPL treatment at day 14. The hardness decreased as a function of increased FSP(L) concentration, and significance was shown from day 10 compared with control. BHA-treated patties showed significantly lower hardness values compared with all other samples. The pH values were proportional to the concentration of FSP added into pork patties. Further research will be needed to see whether the higher concentration (more than 3%) of FSP have the better antioxidant activity in pork patties. Also, other coating material or technology is necessary to improve the deterioration of physicochemical properties of pork patties supplemented with FSP.