Introduction
Most pork by-products are composed of internal organs, such as liver, lung, heart, stomach, small intestine, large intestine, and blood. The food value of the by-products is low due to their odor and tough texture. Consumption of the by-products is poor and only 20% to 30% of the by-products of slaughtered pigs are sold at low prices, with 70% to 80% of all by-products disposed of at slaughterhouses in Korea. Unlawful disposal and utilization of untreated or improperly treated waste without following the regulations for handling, transport, and disposal of waste materials pose serious dangers to the environment (Russ and Meyer-Pittroff, 2004). Proper use of pork byproducts can improve the conversion of byproducts into value-added food materials or bioactive materials for medical or animal product industries.
Conjugated linoleic acid (CLA) is a mixture of geometric and positional isomers, with double bonds at [9, 11], [10, 12], [8, 10], [7, 9], and [11, 13] (Park and Pariza, 2007). Although a number of CLA isomers are found in food, the primary research focus is on the two main isomers, cis-9, trans-11 and trans-10, cis-12. Naturally occurring CLA present in food, such as beef, milk, and dairy products, primarily consists of the cis-9, trans-11 isomer (>80%) (Chin et al., 1994; Park and Pariza, 2007). Consumption of CLA can reduce body fat, has antioxidant activity, and can be beneficial in cancer, diabetes, and hypertension (Ha et al., 1987; Hur et al., 2004; Wang and Jones, 2004). CLA is a popular weight management ingredient with Generally Recognized as Safe status. The market for CLA was projected to exceed $199 million in 2017 in the United States (Global Industry Analysis, Inc., 2017).
In general, most CLA is mass-produced by alkaline isomerization using safflower oil (Chen et al., 2017). Development of an easy and low-cost method of CLA synthesis from pork by-products would be very helpful in reducing slaughter waste and improving the commercial utilization of pork by-products. The purpose of this study was to develop a commercially viable method to synthesize CLA from pork by-products using an unsaturated fatty acids fractionation method to increase synthesis yield, purity, and economic benefit.
Materials and Methods
Pork by-products and the procedure of sample preparation are presented in Fig. 1. The pork (Landrace×Yorkshire×Duroc : LDY crossbred) by-products (liver, lung, heart, stomach, small intestine, and large intestine) were obtained from a local slaughtering house (Anseong-si, Gyeonggido, Korea). The by-products were washed three times with fresh tap water to remove contaminants. The by-products were homogenized to facilitate extraction and synthesis. After homogenization, the samples were subdivided into 200 g portions and frozen (–30℃) until used.
The procedure of crude fat extraction and fraction of unsaturated fatty acids from pork by-products are presented in Fig. 2. Crude fat was extracted with chloroform and methanol as described by Folch et al. (1957). Two hundred grams of each sample (liver, lung, heart, stomach, small intestine, and large intestine) were homogenized at 10,000 rpm for 1 min by adding 1 L Folch solution (chloroform: methanol in a 3:1 ratio). The homogenized samples were vortexed every 30 min at the refrigerated temperature (4℃) for 3 h. Samples were filtered using Whatman No. 1 filter paper, mixed with 200 mL of 0.88% NaCl, and left at refrigeration temperature (4℃) overnight. The supernatant was aspirated and the remaining bottom layer was dried in a fume hood for 12 h to obtain crude fat.
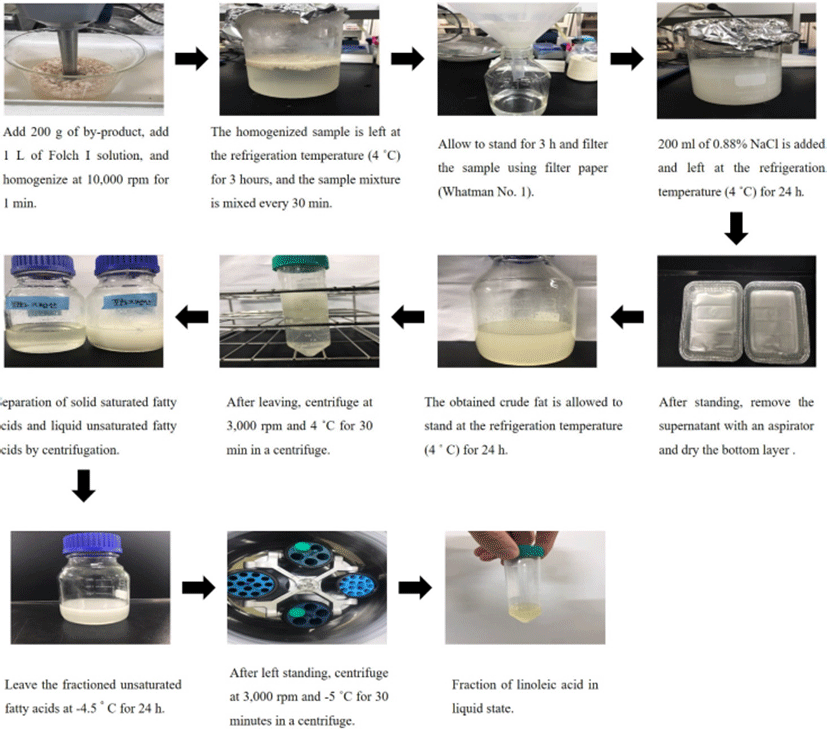
To increase the purity and yield of CLA, unsaturated fatty acid including linoleic acid (the precursor of CLA) was fractionated from crude fat in pork by-products. A simple fractionation method using the physical properties of the fatty acid, including boiling and melting points, was used. In brief, 100 g of crude fat was allowed to stand at refrigeration temperature (4℃) for 24 h and then centrifuged at 101×g for 30 min to separate the solid saturated fatty acid and the liquid unsaturated fatty acid. Unsaturated fatty acids in the liquid state were left at –4.5℃ for 24 h and then centrifuged again at 101×g for 30 min to increase the yield of unsaturated fatty acids. The fractionated unsaturated fatty acids were stored at refrigeration temperature (4℃) until use.
The CLA synthesis protocol from fractioned unsaturated fatty acid obtained from the crude fat of pork by-products is presented in Fig. 3. One hundred grams of propylene glycol was placed in a round flask, and a nitrogen inlet tube, cooling tube, and thermometer were connected to the round flask. The round flask was placed in the heating block. The temperature was increased to 180℃ with the flow of nitrogen so that a small droplet was formed. After 10 min, the temperature was cooled to 160℃ and 26 g of KOH was added. At the time of addition, the amount of nitrogen injected was increased to block the injection of air as much as possible. After the addition of KOH, the temperature was again raised to 180℃ and maintained for 10 min. Five grams of fractionated unsaturated fatty acid from each by-product was added and reacted at 180℃ for 2 h with stirring. After the reaction, the mixture was cooled to room temperature, and 200 mL of methanol (high-performance liquid chromatography grade) was added. After 10 min, 250 mL of 6 N HCl was added and mixed. After transferring the mixture to a separatory funnel, 200 mL hexane and 200 mL distilled deionized water were added and the mixture was shaken several times. The lower layer liquid was poured off, the top layer liquid was collected, and Na2SO4 was added to remove water. Finally, hexane was removed using a vacuum concentrator to collect the synthesized CLA.
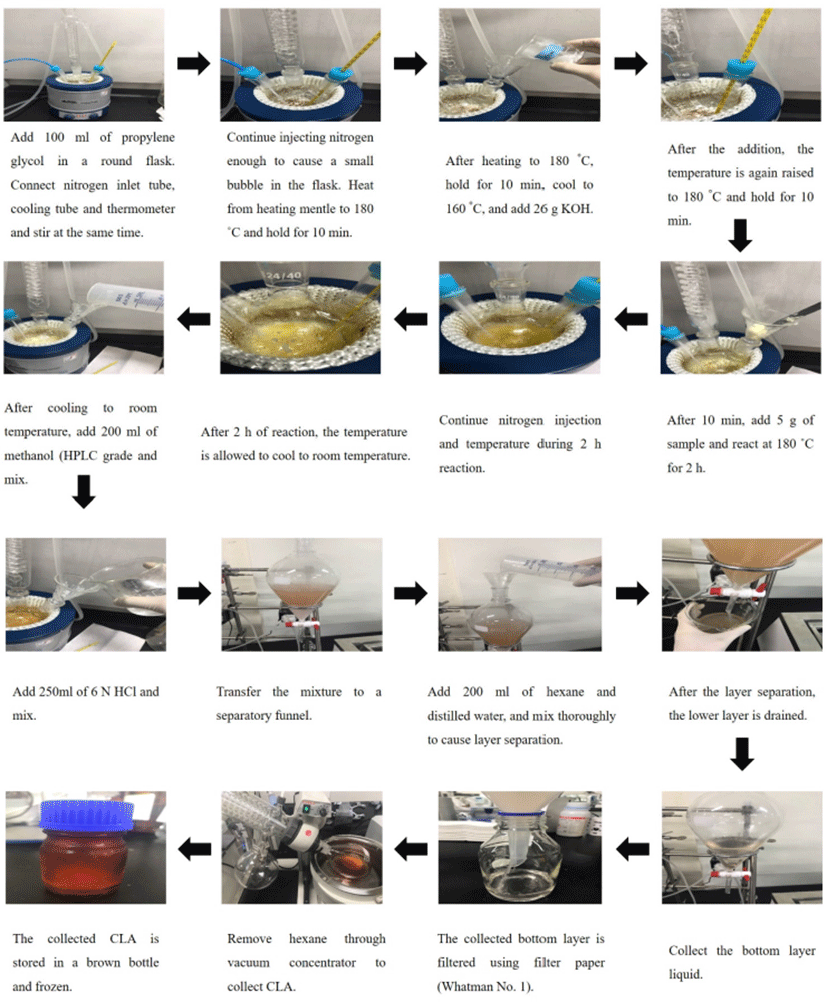
Cis-9/trans-11, trans-10/cis-12, and the combination (cis-9/trans-11, trans-10/cis-12) CLA of by-products (liver, lung, heart, stomach, small intestine, and large intestine) were determined as previously described (Hur et al., 2004) with slight modification. Lipids from pork by-products were extracted with chloroform and methanol as previously described (Folch et al., 1957). For lipid hydrolysis, an aliquot of the lipid extract (30 mg) and 3 mL of 0.25 mM H2SO4 in methanol were combined in a screwcap test tube. The test tube was placed in boiling water (100℃) for 20 min and subsequently cooled at 25℃. The resulting CLA was methylated with boron trifluoride (140 mg in 1 mL methanol) at room temperature for 30 min. Water (1 mL) and hexane (5 mL) were added. Samples were vortexed and centrifuged at 500×g for 10 min. The upper organic solvent layer was used to determine CLA concentration. Fatty acid methyl esters were analyzed on a model 6,890 gas chromatograph (Agilent, Wilmington, DE, USA) equipped with an on-column injector port and flame ionization detector. A Silar capillary column (30 m×0.32 mm×0.25 µm) was used for the separation of the fatty acid methyl esters. The initial gas chromatography oven temperature was 140℃ and was increased at a rate of 2℃ /min to a final temperature of 230℃. The injector port and detector temperatures were set at 240℃ and 250℃, respectively. Fatty acid methyl ester (1 mL) was injected onto the split injection port (100:1 split ratio). The flow rate for the helium carrier gas was 50 mL/min. CLA concentration was measured by comparison to the retention time of standard CLA samples.
Statistical analyses were conducted for three batches of by-products. Data were analyzed by the generalized linear model procedure using SAS software (SAS Institute Inc., Cary, NC). The Student-Newman-Keuls' multiple range test was used to compare differences among means. Significant differences (p<0.05) between mean values of quintuplicate samples were determined for the amount of CLA.
Results and Discussion
The chemical compositions of each pork by-product are presented in Table 1. The moisture contents of pork by-products ranged from 75.15% to 83.41%. The contents of crude fat varied among the pork by-products. In particular, small intestine and large intestine contained higher amounts of crude fat than the other pork by-products. The fatty acid composition of the pork by-products is presented in Table 2. The content of linoleic acid, the key precursor of CLA, ranged from 7.27 to 18.09 g per 100 g of by-products. The fractionation rate of linoleic acid ranged from 5.92 to 12.20 g per 100 g crude fat by-products. The amount of linoleic acid in the total crude fat of the by-products was higher in the small intestine and large intestine than other by-products. Therefore, the yield of CLA could be higher in the small intestine and large intestine than other by-products in the total crude fat base.
The main natural CLA isomers are cis-9, trans-11 and trans-10, cis-12 CLA (Park and Pariza, 2007). Consistent with this, we also found that the main isomers of CLA synthesized from pork by-products were cis-9, trans-11 and trans-10, cis-12 (Fig. 4). Both cis-9, trans-11 and trans-10, cis-12 CLA have anti-cancer activity (Masso-Welch et al., 2004; Park and Pariza, 2007) and, in particular, the trans-10, cis-12 CLA isomer is responsible for the reduction in body fat (Hur et al., 2009; Park and Pariza, 2007). Therefore, cis-9, trans-11 and trans-10, cis-12 CLA synthesized from pork by-products can be used as CLA sources as bioactive components. Ruminant species and their products are a rich source of CLA, and cis-9, trans-11 CLA is commonly produced during the partial biohydrogenation of linoleic and linolenic acids in the rumen (Coakley et al., 2003; Hur et al., 2017). However, monogastric animals, such as swine, cannot easily increase CLA concentration through the activity of enterobacteria using linoleic acid as a key precursor (Hur et al., 2017). Thus, synthesis of CLA after fractionation would be a useful way to obtain CLA from pork.
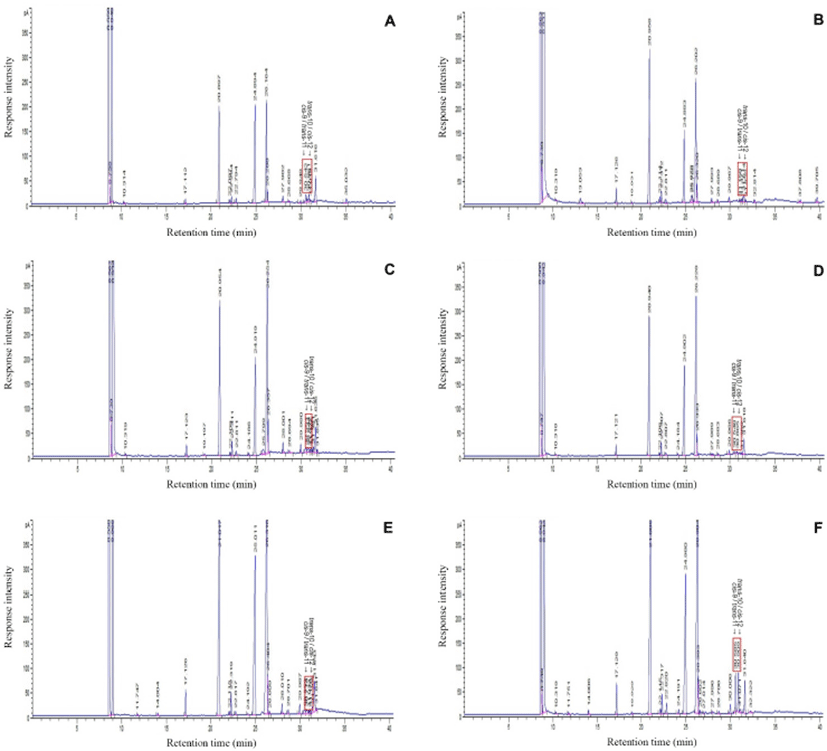
The yields of synthesized CLA from by-products before and after fractionation are presented in Fig. 5. The yield of CLA synthesized from raw pork by-products ranged from 0.19 to 0.55 g per 100 g raw materials. The yield of CLA synthesized in crude fat (non-fractioned) extracted from pork by-products ranged 0.59 to 5.29 g per 100 g crude fat. However, the yield of CLA synthesis was doubled after commercial fractionation compared to non-fractionated crude fat. The yield of CLA synthesized from fractioned fat ranged from 1.55 to 11.18 g per 100 g fractioned fatty acid. This result indicated that the yield of CLA could be doubled by commercial fractionation methods in pork by-products. This increase is possible because linoleic acid is a precursor of CLA and the concentration of linoleic acid was also doubled by commercial fractionation in pork by-products.
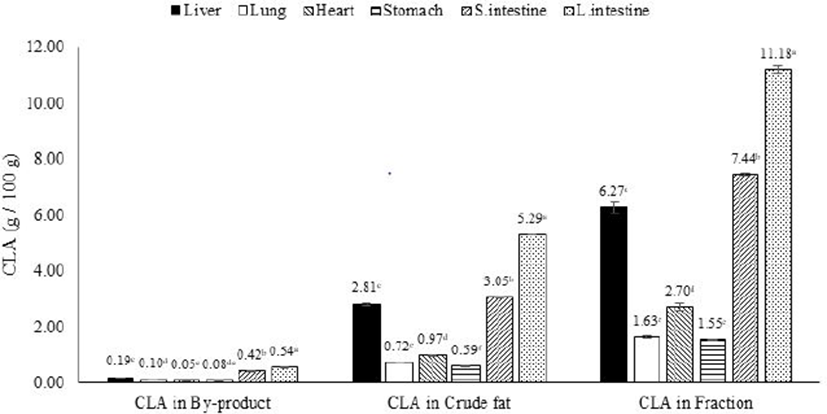
To increase the synthesis of CLA to commercially-viable quantities, a simple fractionation method that relies on the melting point of fatty acids was applied in this study. The doubling of the synthetic yield of CLA by the fractionation protocol indicates the value of this method to increase the yield of CLA in pork by-products.
Conclusion
A commercially viable and low-cost CLA synthesis method was developed from pork by-products using a novel fractionation method, and cis-9, trans-11, and trans-10, cis-12 CLA was synthesized from pork by-products. The amount of synthesized CLA was the highest in the small intestine and large intestine by-products. Fractionation of unsaturated fatty acid from the pork by-products approximately doubled the yield of CLA synthesis. The data indicate the potential for increased CLA synthesis from commercial fractionation at low cost.