Introduction
Establishment of food safety is a major task for securing the well-being of a population and remains a public health challenge. Microorganisms are the major cause of food poisoning incidents (Nyachuba, 2010), and the long-standing and continuous outbreaks of food poisoning by microorganisms demonstrate the difficulty of controlling pathogenic microorganisms. For example, 4,265 cases of food poisoning were reported in Korea between 2002 and 2017, affecting 111,947 patients (Portal of Food Safety Information, 2018). Among them, 332 cases involving 10,378 patients were attributed to Salmonella infection, which was the third most common cause of food poisoning only after enteropathogenic Escherichia coli and norovirus (Portal of Food Safety Information, 2018). Survey studies have shown that poultry consumption is one of the major causes of Salmonella infection in Korea (Chung et al., 2003; Kim et al., 2012).
Oscar (2004) performed a risk analysis for Salmonella on broilers along the pathway from retail to consumption. However, it is necessary to explore the link between the processing at slaughterhouses and the pathway from retail to consumption because the likelihood of illness after consumption depends on the concentration of Salmonella in broilers at retail (Oscar, 2004). Additionally, evaluation of the impact of the stage of production on risk management emphasized the significance of the processing stage of broilers for food safety (Nauta et al., 2005). It is, thus, necessary to understand how much contamination can be managed in the stages from retail to consumption, and how the level of contamination can be minimized in the stage of processing at slaughterhouses. Chlorination has long been used to decrease the Salmonella contamination level in poultry (Paul et al., 2017). A chlorination level of 20 ppm is widely used to maintain food safety in poultry slaughterhouse in Korea (Woo, 2007). However, Woo (2007) found that treating chickens with chlorination did not have a significant effect on reducing the Salmonella load through a survey on the status of microbial contamination in slaughterhouses. It is, therefore, necessary to determine whether a significant increase in chlorine concentration can provide the required reduction in the concentration of Salmonella in chicken.
Quantitative microbial risk assessment (QMRA) is a relatively useful method that was introduced to quantify risk (WHO, 2002). With the use of QMRA, the probability of acquiring a microbial infection can be estimated by quantifying the prevalence and concentrations of microbes present during each procedure related to food preparation and consumption (Whiting and Buchanan, 1997). Oscar (2004) built QMRA for Salmonella and whole chickens to determine the relationship between the most probable number of Salmonella cells and the illness probability, and how this relationship is affected by each stage in the retail-to-table pathway in the United States. Following this work, in the present study, we built QMRA tracking the procedures from the retail to household consumption stages with respect to Salmonella in chicken. given the specific processing conditions and data of Korea, and explored hypothetical situations in which illness caused by consumption of Salmonella-contaminated chicken is more likely.
QMRA of Salmonella in broilers should be region-specific from country to country, depending on the contamination level of Salmonella in broilers at retail shops and the circumstances of shopping and cooking styles. We built the QMRA based on the Korean circumstance and chose the cooking method of Dakgalbi, which is a traditional and common recipe for chickens in Korea (Muhlisin et al., 2012).
The goal of the study was two-fold: (1) to conduct a baseline microbial risk assessment for Salmonella in chicken employing Monte Carlo simulation, and to perform a sensitivity analysis for the parameters on the probability of illness; (2) to explore how the level of chlorination affects the likelihood of illness. Based on the results, we provide a potential foundation for food safety in terms of risk management for Salmonella in chicken.
Materials and Methods
We modeled the retail-to-table pathway in Korea by covering the steps beginning at purchase of a chicken from a retail shop to the final consumption of the chicken. Accordingly, the QMRA consisted of the following four stages: stage 1, initial contamination level at retail; stage 2, microbial growth during transport from retail shops to household refrigerators; stage 3, thermal inactivation during cooking; and stage 4, dose-dependent illness after consumption. In addition, the effects of the chlorination procedure were considered at stage 1, although in practice chlorination is actually performed during the chilling process in slaughterhouses (James et al., 2006). Salmonella was not assumed to grow during storage in a refrigerator, because properly working refrigerators keep the temperature below 10℃, i.e., the minimum growth temperature of Salmonella (WHO, 2002). The model excluded the potential of cross-contamination of Salmonella between chicken and other ingredients such as vegetables so as to best focus on the salmonellosis caused specifically by chicken consumption, due to the lack of survey data for cross-contamination in Korea. We tried to incorporate realistic consumption conditions in Korea into the model as much as possible. If relevant data were not available, we referred to foreign data, expert opinion, and logical inferences. The model was simulated with @RISK (Palisade Corporation, Ithaca, NY) using a Monte Carlo simulation with settings of Latin hypercube sampling and was iterated 100,000 times. We employed a high number of iterations because of the extremely low values of the likelihood of illness estimated by this study.
The first stage in the QMRA simulated the contamination level of Salmonella in chicken at a retail shop using the prevalence and concentration of Salmonella in chicken. The prevalence of Salmonella in retail chicken in Korea is widely variable, with values of 22.4% (Kim et al., 2012), 36.0% (Chung et al., 2003), and 42.3% (Hyeon et al., 2011) reported. We used the minimum (22.4%), maximum (42.3%), and average of the four values (31.65%). The concentration of Salmonella per chicken was estimated based on a previous survey conducted on whole raw chicken purchased from a local market in Korea (Hong et al., 2008).
The second stage simulated the growth of Salmonella in chicken during transportation of the chicken from the retail shop to the home. The period of transport was estimated by using the locations of “e-mart”, a major retail franchise in Korea. We spotted two locations of e-mart in Seoul, Korea, and measured the distance between the two, which was 4.1 km. We supposed that consumers visit an e-mart at half of this distance (2.1 km) using a private car or public transportation. A private car and public transportation were expected to take 17 and 35 min, respectively. We used the minimum (17 min), maximum (35 min), and average (26 min) for the Pert distribution. The surveyed average shopping time of Koreans was 1–2 hours (Trendmonitor, 2018). We added half of the average shopping time to the transport time. The ambient temperature during transport was assumed to be the average temperature in Korea (Korea Meteorological Administration, 2018). The transport temperature was used to calculate the growth rate and lag time to estimate the contamination level during transport. The temperature of raw chicken purchased at a retail shop was supposed to increase exponentially per 60/min. Until the temperature of the chicken reached ambient temperature, the average of temperature of the chicken at retail and the temperature of the chicken after transport were assumed to be the temperature of the chicken. If the temperature of the chicken reached the ambient temperature during transport, the temperature of the chicken was assumed to be the ambient temperature. The growth rate of Salmonella in chicken was determined based on the secondary model of Baranyi (Juneja et al., 2007), and the lag time was based on experimental validation of the lag time of Salmonella Typhimurium (Oscar, 2005). The maximum theoretical concentration of 9.5 Log CFU/g was assumed based on the findings of Silva et al. (2009).
The third stage simulated the thermal inactivation of Salmonella during the cooking of chicken. The cooking method was based the recipe of Dakgalbi. The parameters included in the cooking stage were cooking temperature and time. The chicken was cooked at 75℃ to reach an internal temperature of 71℃ (Song et al., 2014), such that 0.8 and 1.2 times 71℃ were used for the Pert distribution. We referred to Lee et al. (2011) for cooking time. The cooking temperature was used to calculate the decimal reduction time (D-value), which is the time required to kill 90% of the microbes of interest (Liu et al., 2015). A thermal inactivation model developed for Salmonella in chicken patties was used to estimate changes in the D-value depending on temperature (Murphy et al., 2002). The ratio of cooking time to the D-value was used to calculate the log cycle reduction, which is the overall parameter indicating the thermal inactivation effect at the cooking stage.
The fourth and final stage simulated the consumption of chicken. The amount of chicken consumed was set to reflect the typical amount of Dakgalbi eaten by Koreans in a given meal. The dose-response model of Salmonella referred to WHO (2002). The outcome of this QMRA was the likelihood of salmonellosis caused by consumption of Salmonella-contaminated chicken.
In the baseline model, we used the most probable ranges of parameter values that may affect the risk of salmonellosis. The effect of chlorination to decrease the concentration of Salmonella was not considered in the baseline model. The formulas, units, and input settings used in the baseline model are shown in Table 1. In addition, sensitivity analysis was performed to provide a quantitative measure of the most important parameters affecting the probability of illness from Salmonella via the pathway explored in the baseline model. The value of performing this sensitivity analysis is that the general results can be used to prioritize risk mitigation strategies based on the parameters that emerge to show the strongest impact on risk (Zwietering and van Gerwen, 2000). Spearman rank correlation using @RISK was employed to determine the parameters that are most highly correlated with the probability of illness from Salmonella.
Unit operation | Variables | Description | Unit | Formula | Reference |
---|---|---|---|---|---|
Retail | P | Prevalence of Salmonella at retail | Pert(22.4, 31.65, 42.3) | (Chung et al., 2003; Hyeon et al., 2011; Kim et al., 2012) | |
COSC | Contamination of chicken with Salmonella at retail | Discrete([0, 1], [100–P, P]) | |||
TeR | Temperature of chicken at retail | ℃ | 4 | (WHO, 2002) | |
LCSC | Log concentration of Salmonella at retail | Log(CFU/g) | Pert(4.3, 5.3, 6.3) | (Hong et al., 2008) | |
CSC | Concentration of Salmonella at retail | CFU/g | 10^LCSC | ||
CCH | Concentration of chlorination | ppm | 0 | ||
λ | Parameter of Salmonella reduction effect | 1 | |||
ORE | Contamination level at retail | CFU/g | IF(COSC=0, 0, CSC×λ) | ||
Transport | TiT | Transport and shopping time | h | Pert(17/60, 26/60, 35/60)+Pert(0.5, 0.75, 1) | (Trendmonitor, 2018) |
TeT | Ambient temperature | ℃ | Pert(min, median, max) | (Korea Meteorological Administration, 2018) | |
TeTR | Chicken temperature after transport | ℃ | IF(exp(TiT)–1+TeR>TeT, TeT, exp(TiT)–1+TeR) | ||
AvTeTR | Average temperature between retail and after transport | ℃ | Average(TeR, TeTR) | ||
TIAMTR | Time when chicken temperature reaches ambient temperature | h | LOG(TeT, exp(1)) | ||
LT | Lag time of Salmonella | h | 1/(0.02082×AvTeTR–0.01235)2 | (Oscar, 2005) | |
GRRETR | Growth rate of Salmonella between retail and transport before chicken reaches ambient temperature | Log(CFU/g/h) | IF(AvTeTR>TeT, 0.0019×(AvTeTR–3.35)^2×(1–exp(0.29×(AvTeTR–48.01))), 0) | (Juneja et al., 2007) | |
GRAMTR | Growth rate of Salmonella at ambient temperature during transport | Log(CFU/g/h) | 0.0019×(TeT–3.35)^2×(1–exp(0.29× (TeT–48.01))) | (Juneja et al., 2007) | |
MPD | Maximum population density | Log(CFU/g) | 9.5 | (Silva et al., 2009) | |
GS | Growth of Salmonella | Log(CFU/g) | IF(TIT<TIAMTR, GRRETR×TiT, GRRETR×TIAMTR+GRAMTR×(TiT–TIAMTR)) | ||
OTR | Contamination level after transport | CFU/g | IF(ORE+exp(GS)<exp(MPD), ORE+exp(GS), exp(MPD)) | ||
Cooking | TeC | Cooking temperature | ℃ | Pert(71×0.8, 71, 71×1.2) | (Song et al., 2014) |
TiC | Cooking time | min | Pert(10, 15, 20) | (Lee et al., 2011) | |
D | D-value | min | 10^(8.7344–(0.1316×TeC)) | (Murphy et al., 2002) | |
LCR | Log cycle reduction | - | TiC/D | ||
OC | Contamination level after cooking | CFU/g | (10^(–LCR))×OTR | ||
Consumption | CW | Consumption weight | g | Pert(100, 200, 300) | (Fatsecret, 2018) |
Dose | Amount of Salmonella consumed | CFU | OC×CW | ||
PILL | Probability of illness | IF(P=0, 0, (1– (1+10^Dose/2885)^ (–0.3126))) | (WHO, 2002) |
In the chlorination model, we applied the different levels of chlorination effects in stage 1 to simulate the reduction of Salmonella concentration in chickens at retail, and we explored whether the decrease in Salmonella concentration in chickens at retail by chlorination can affect the likelihood of illness after consumption of Salmonella-contaminated chicken. A predictive model for the effect of chlorination to reduce the concentrations of Salmonella in chicken was based on previous findings showing that 50, 100, and 200 ppm chlorine reduced the Salmonella load by 1 Log CFU/g (Northcutt et al., 2005), 1.4 Log CFU/g (Hong et al., 2008), and 2 Log CFU/g (Lu and Wu, 2012), respectively. The square root of the published chlorination effect was plotted against the chlorination concentration and linear regression was used to model the effect of chlorine concentration on Salmonella reduction (Fig. 1). In the chlorination model, we first used fixed values of chlorination concentration (0, 50, 100, 200, and 400 ppm). Then, for sensitivity analysis, we used the Pert distribution of chlorination concentration of 0, 200, and 400 ppm for minimum, most likely, and maximum values, respectively.
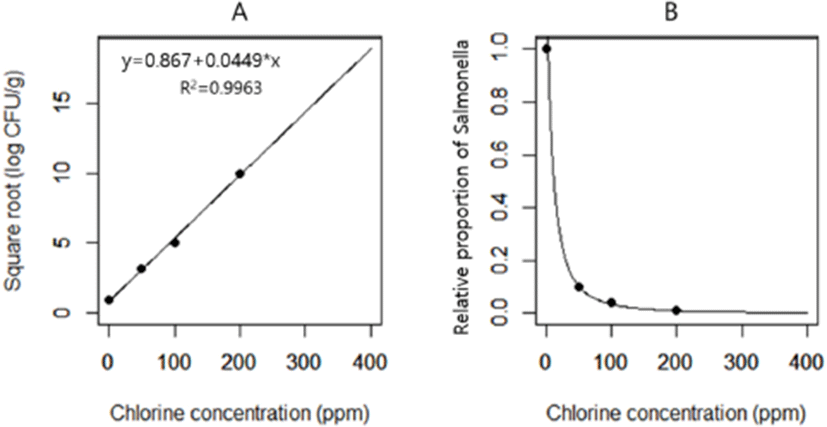
Results
The simulation results of the baseline models showed that the likelihood of illness was not affected by the transport temperature (Table 2). The short distances between retail shops in Seoul contributed to ensure food safety during transport, because microbial growth could hardly be achieved during such a short time of exposure to ambient temperature.
Month | Average likelihood of illness |
---|---|
March | 0.0127 |
April | 0.0112 |
May | 0.0122 |
June | 0.0124 |
July | 0.0111 |
August | 0.0120 |
September | 0.0122 |
October | 0.0120 |
November | 0.0125 |
The sensitivity analysis indicated that the prevalence of Salmonella in broilers had a predominant impact on deciding the likelihood of illness after consumption of Dakgalbi (Fig. 2A). We also performed the sensitivity analysis with the deterministic value of Salmonella prevalence, which meant that the chicken at retail was always contaminated with Salmonella (Fig. 2B). The results of this sensitivity analysis showed that cooking temperature had a predominant effect on deciding the probability of illness.
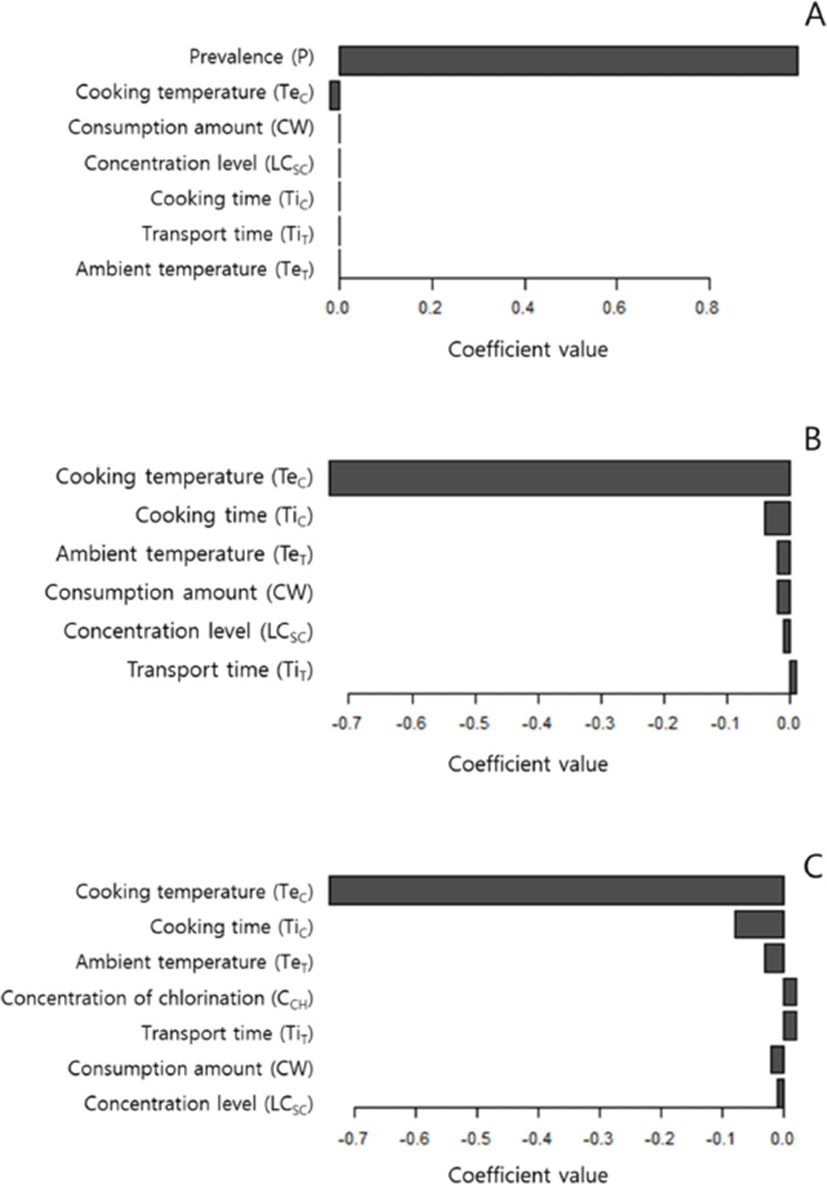
In the chlorination model, we explored whether the effect of chlorination on reduction of Salmonella concentration in broiler chickens can reduce the probability of illness. Increased concentration of chlorination had little effect on the probability of illness (Fig. 3). This finding indicates that the usage of methods including chlorination during chilling at poultry slaughterhouses that could decrease the concentration of Salmonella should not be recommended for reducing the probability of illness. The usage of chlorination during the chilling process was simulated to reduce the concentration of Salmonella in chickens at the retail stage. However, the sensitivity analysis of the chlorination model showed that the effect of the reduced concentration through chlorination was negated by the following stages, particularly the cooking stage (Fig. 2C).
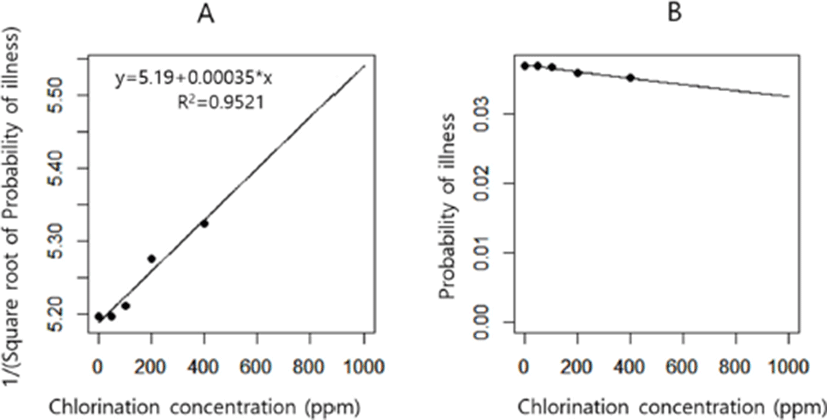
Discussion
The QMRA developed in this study covers all of the processes involved in the likelihood of becoming infected with Salmonella from a contaminated chicken, from the retail to consumption stage. By combining published data for the Salmonella contamination level (Hong et al., 2008) and prevalence (Chung et al., 2003; Hyeon et al., 2011; Kim et al., 2012) at retail in Korea, reduction of Salmonella concentration by chlorination (Hong et al., 2008; Lu and Wu, 2012; Northcutt et al., 2005), microbial growth (Juneja et al., 2007; Oscar, 2005; Silva et al., 2009), thermal inactivation (Murphy et al., 2002), and dose dependence of food illness (WHO, 2002), we estimated the probability of illness caused by the consumption of Salmonella-contaminated chicken and explored the relative significance of each parameter for the probability of illness. In this way, we were able to determine the parameters that should be focused on for preventing illness.
The methods to reduce the concentration of Salmonella in broilers during processing steps at slaughterhouses could reduce the concentration of Salmonella on raw chickens at retail shops, but this effect was found to be negligible compared to the effect of cooking for thermal inactivation of Salmonella. Although increasing the concentration of chlorination can decrease the probability of illness to some extent at the retail stage, the probability of illness was found to be minimally affected by the chlorination concentration. This finding emphasizes that disinfection of microbes at slaughterhouses should be performed very meticulously after sufficient validation is found. In addition, a high concentration of chlorination is not recommended because a chlorine-based washing solution might be detrimental to human health or deteriorate the quality of chicken (Lu and Wu, 2012).
The finding that the prevalence of Salmonella in chicken has a predominant impact on the likelihood of illness suggests the importance of prevention of Salmonella contamination at stages of rearing and slaughtering. The efforts to decrease the contamination level of Salmonella in chicken at the slaughtering stage should be preceded by efforts to prevent contamination. Therefore, it is necessary to understand the routes of Salmonella infection in poultry rearing farms and to prevent the contamination of broiler with Salmonella (Rose et al., 1999). In slaughterhouses, efforts to decrease the contamination of chicken with microbial pathogens should be focused on the steps that may cause cross-contamination during slaughtering, such as evisceration rather than the chilling step that uses disinfectant agents (Hue et al., 2010). Specifically, to reduce the prevalence of Salmonella in chicken meat at retail, it is essential to determine the points of the process that are most prone to Salmonella contamination. Kim et al. (2012) showed that the highest prevalence of Salmonella occurred in broiler transporting trucks (71.43%), followed by the slaughterhouse (63.89%) and broiler farms (16.05%). According to this finding, further efforts to reduce the prevalence at retail should be concentrated on the processes involved in the transportation and slaughter of broilers. In addition, the prevalence of Salmonella in chicken meat will vary substantially depending on the distribution channel. One survey in Korea demonstrated that the prevalence of Salmonella in chicken meat was 25% at wholesale markets, 44.4% at retail stores, and 55.56% at traditional markets (Hyeon et al., 2011). This finding emphasizes the need to apply strict food safety protocols, particularly at traditional markets. Comparison to studies on the prevalence of Salmonella in chicken meat at retail worldwide shows that the prevalence in Korea is similar to that reported in other countries: 41.6% (Zhu et al., 2014) and 33.8% (Huang et al., 2016) in China, 30% in the USA (Oscar, 2004), and 30% in Canada (Smadi and Sargeant, 2012).
Microbes are sensitive to high temperature (van Asselt and Zwietering, 2006); thus, encouraging the public to cook meat at a high temperature can reduce the possibility of an outbreak of foodborne illness. The sensitivity analysis without Salmonella prevalence (Fig. 2B) supported the well-known fact that even a slight change in cooking temperature can generate a massive impact on the probability of illness, even without changing any other parameter (van Boekel, 2002). Nevertheless, it is not always easy to apply a sufficiently high temperature to chicken meat, because some widely eaten chicken recipes in Korea make it difficult to effectively cook the deeper parts of the chicken meat. Compared to boiling and frying, stir-frying in a pan is a poor condition for achieving high internal temperature of meat pieces and for accomplishing effective thermal inactivation of Salmonella. For example, Koreans often use built-in grills or portable burners on tables to stir-fry chicken meat while eating it. This cooking style increases the likelihood of eating less-cooked chicken meat and the likelihood of causing cross-contamination from uncooked chicken meat to vegetables because the meat is cooked not by a professional chef but by consumers. Therefore, the risk caused by the consumption of less-cooked chicken meat is particularly significant due to this characteristic Korean cooking style. Additionally, one recipe in Korea and Japan involves eating chicken sashimi, and this eating habit has been involved in food safety concerns (Asoshima et al., 2015; Moore and Matsuda, 2007). Considering the critical importance of cooking and the limited effect of chlorination on the probability of illness, eating raw chicken meat should be strongly discouraged in terms of food safety, unless the prevalence and concentration of Salmonella in raw chicken at retail are substantially improved.
In this study, we excluded some parameters that may also affect the probability of illness but cannot be logically assumed or reliably referred. For example, cross-contamination between raw chicken and vegetables during the preparation of foods may occur in the kitchen and can be an important source of salmonellosis (Luber, 2009). The contamination of Salmonella from a contaminated raw chicken to a cooked chicken is also possible and is called self-contamination (Oscar, 2004). However, this was too difficult to simulate as there are too many factors related to such cross-contamination, which would be difficult to model with only a few parameters (for example, the transfer rate from raw chicken to vegetables or utensils, consumption amount of vegetables, microbial growth rate on vegetables and utensils, and storage of contaminated vegetables). Although Oscar (2004) applied data from consumer surveys of handling practices of cooked food and laboratory studies that quantified the transfer rates of Salmonella, we did not refer to these data in the present model, because such variables would be highly dependent on the specific recipe and conventional handling practices of chicken in different countries. Therefore, there is a need to properly quantify the level of cross-contamination from chicken in Korea, especially given the increasing trend of eating chicken with uncooked vegetables such as chicken salad.
Conclusion
In summary, given the current data on the concentration of Salmonella in chicken at the retail level, one risk management option might be to prioritize the prevention of Salmonella contamination. Other than reducing the prevalence, use of a high cooking temperature is the most reliable method to ensure safe consumption of chicken without illness caused by Salmonella. Although methods to reduce the concentration of Salmonella at slaughterhouses can be an effective measure to decrease the Salmonella concentration in chicken at retail, as a sole practice, these methods are far from guaranteeing safety, even at high concentrations of chlorination.
The establishment of standardized chicken risk management guidelines would be useful for consumers concerned about individual or family food safety. In this regard, the present risk assessment may also be useful for food safety-related authorities when setting a quantitative standard for Salmonella in chicken. Finally, this risk assessment points to the need for additional research on the effects of other factors (e.g., concentration of Salmonella on chicken, cross-contamination, and effects of cooking depending on the recipe), which may be useful in securing the safety of chicken from salmonellosis.