Introduction
Cooking is one of the main methods for safe preparation of food ingredients such as vegetables and meat. Cooking process on meat and meat products can prevent the spread of food-borne diseases. Although it is an effective way to eat safely, the cooking process can affect the meat protein quality through protein denaturation and degradation (Gao et al., 2009; Murphy and Marks, 2000). Cooking causes that the denaturation of protein dimensional structure and alters the amino acid residues present in meat (Yu et al., 2017). Depends on the meat states (frozen, thawed and fresh), the cooking proved significantly differences for color, cooking loss, shear force and sensory characteristics in meats (Obuz and Dikeman, 2003). Previously, the relationship between moisture content and cooking traits of beefs using a water bath was reported by Oillic et al. (2011). They are reported that the dry-aged beef might be less affected by cooking procedure since the initial moisture content was low in dry-aged beef.
Dry-aged beef has been proven to lower shear force and contain plentiful free amino acids, which are involved in the Maillard reaction, which accounts for improved flavor after cooking (Lee et al., 2017; Obuz et al., 2014). Previously, the limited research on dry-aged beef found that the dry-aging (DA) process, with some modifications, such as different muscles, aging conditions and packaging methods, changes meat quality, and lipid oxidation. However, it may be possible that protein of dry-aged beef was highly affected by the cooking process compared to fresh meat protein, since the protein was denatured/degraded by proteolysis during DA.
Although the DA does have positive effects on sensory characteristics as the above mentioned results, there has been limited studies determining how the interaction between DA and cooking affects the protein characteristics and digestion of beef. We speculated that the DA and heating provide significant effects on protein characteristics. Therefore, the objective of this study was to compare the protein characteristics of fresh and dry-aged beef after heating. Our study could provide basic knowledge of the DA and heating effects on protein characteristics of beef longissiumus dorsi.
Material and Methods
Sirloins at 2 d postmortem, from a total of 6 Holstein steers (quality grade 2, approximately 26 months age), were purchased from a commercial slaughterhouse. The samples were bisected, and randomly assigned into two groups, a control and dry-aged beef (n=6). Dimensional changes, cooking rate (CR) and shear force of fresh samples were immediately measured. For dry-aged beef, the sirloin was hung in a refrigerated room with ventilation. The condition of DA was 1°C, relative humidity about 85%, and 0.5–1.5 m/s of air circulation for 28 d (Dashdorj et al., 2016). The myofibril protein was immediately extracted from the fresh and dry-aged samples, and frozen at –80°C for further analyses.
Samples at 4°C were cut into cube shapes (3×3×2.54 cm, diameter×diameter×thickness) and then cooked at 145°C using preheated electronic grills. The core temperature of samples reached 71.1°C, as measured using a digital thermocouple machine (Tes-1305, Tes Electrical Co., Taiwan) equipped with a data logger (RS-232, Tes Electrical Co., Taiwan). Dimensional changes were calculated as percentages of changes of thickness and surface shrinkage. The CR was expressed as °C/min. After cooking, the core samples were collected parallel to the muscle fiber shape by using a 1.27 cm diameter handed coring device, and the shear force was measured using a TA-XT2i (Stable Micro System Ltd., Surrey, UK) equipped with a triangle angular slot cutting edge under a crosshead speed of 1.5 mm/s (Kim et al., 2017).
In vitro gastrointestinal digestion model was conducted in specific steps, with four phases (mouth, gastric, duodenal, and bile juices), as described by Kim and Hur (2018). The protein digestibility in the cooked beef during simulated human gastrointestinal digestion was measured by the infiltration rates of the dialysis tubing and expressed as the percentage of protein concentration inside and outside the dialysis tubing. The protein concentration was measured by the Biuret method.
MFI measurement was conducted using the method of Culler et al. (1978).
The myofibril protein extracts were used to assess protein functionalities (aggregation and hydrophobicity) and oxidation (carbonyl and sulfhydryl content). Before the aggregation and hydrophobicity measurements, the myofibril protein concentration was adjusted at 0.1 mg/mL. Hydrophobicity of the myofibril protein was measured using the Bromophenol Blue (BPB) bound ability according to Chelh et al. (2006). The aggregation of the myofibril protein was determined using Nile Red to fluoresce the aggregates after binding in accordance with the method of Demeule, Gurny, and Arvinte (2007). Optimum excitation (λex) and emission (λem) wavelengths were 560 nm and 620 nm, respectively. The intensity of fluorescence was expressed as arbitrary units (au).
Carbonyl content measurement of myofibril protein was carried out in accordance with the method of Vossen and De Smet (2015). Sulfhydryl content of myofibrillar proteins was determined after derivatization by Ellman’s reagent with 5,5’-dithiobis(2-nitrobenzoic acid) (DTNB), according to Jornberg et al. (2013). The sulfhydryl content was expressed as nM/ mg protein.
The protein degradation (each sample 0.5 mg protein/ mL) in meat was evaluated by 12% sodium dodecyl sulfate-polyacrylamide gel electrophoresis (SDS-PAGE) according to the method of Laemmli (1970). Samples (0.67%) and Laemmli sample buffer (Bio-Rad Lab, Inc., USA) was mixed. The mixtures were heated to 100°C for 5 min, and 15 µL of each sample was injected into the wells of a 12% Mini-PROTEAN® TGX™ Precast Gel (Bio-Rad Lab, Inc., USA). The loaded gel was stained using Coomassie Brilliant Blue R250 (B7920, Sigma, USA). The separated protein bands were identified by comparing with those of standard protein markers (Precision Plus Protein Standards, Catalog number 1610374, Bio-Rad Lab., USA).
Six muscles per treatment (control and dry-aged beef) and cooking process (uncooked and cooked meat) were used as experimental units. Statistical analysis was performed using two-way analysis of variance (ANOVA), followed with paired Student’s t-tests to determine the effects of DA and cooking on protein characteristic differences (p<0.05), whereas one-way ANOVA was carried out on the reduction of diameter and thickness, shear force and CR. Pearson correlation coefficients were collected with the measured variables as a 95% confidence level. The statistical analysis was conducted using SPSS version 19.0.
Results and Discussion
Means for the percentage of thickness and surface reduction, shear force and CR of dry-aged beef loin are shown in Table 1. The thickness of the control group showed greater reduction (p<0.01) relative to dry-aged beef (–10.03% and –0.17%, respectively). In addition, the dry-aged beef lost significantly less in surface (9.91%) compared to the control group (17.02%). Dimensional changes of meat could have occurred by the loss of water, resulting in the protein denaturation and cell membrane destruction during cooking, in agreement with Ritchey and Hosterler (1964), and Rowe (1989). However, the dry-aged beef contained lower moisture content than the non-aged beef due to the evaporation of moisture in meat during aging (Obuz et al., 2014). Decreased moisture content for dry-aged beef were reported by Berger et al. (2018). In agreement with Oillic et al. (2011), the dimensional changes of dry-aged beef might be less affected by cooking procedure since the initial moisture content was low in dry-aged beef, regardless of the protein denaturation.
Dry aging decreased shear force compared to the control group (3.23 and 5.25 kg, p<0.001). The lower shear force of dry-aged beef can be explained by the high proteolysis index compared to the control group, which showed similarity to the results reported by Lee et al. (2017).
The CR from 4°C to 71.1°C of the meat was numerically different between the control and dry-aged beef (8.14°C and 7.23°C/min, respectively), but not significant (p>0.05). The CR could be related to the amount of moisture, which has high thermal conductivity. Similarly, Elansari and Hobani (2009) found that thermal conductivity of meat linearly increased with moisture content.
Aging and cooking effects on myofibrillar fragment index (MFI), protein functionalities (aggregation and hydrophobicity) and in vitro digestibility are presented in Table 1. Interacting effects of treatment and cooking on protein aggregation and hydrophobicity were detected. With cooking, the protein aggregation and hydrophobicity of dry-aged beef rapidly increased, in agreement with Traore et al. (2012). The increase in the aggregation and hydrophobicity in dry-aged beef could be associated with protein cross-linking, fragmentation, and a part of amino acid residual exposure from the protein surface to outside due to protein denaturation (Lund et al., 2011). Therefore, it seemed that the protein surface area increased by the protein fragmentation, with the result that the protein from dry-aged beef may be highly reactive to thermal processing. Similarly, Bax et al. (2012) demonstrated that the number of particles in myofibril extract increased in aged meat due to proteolysis. The significant increase of MFI (p<0.05) in dry-aged beef occurred by proteolysis during aging time, whereas the MFI significantly decreased by cooking, regardless of the treatment. Dry-aged beef showed significantly higher in vitro protein digestibility compared to the control group, and the in vitro protein digestibility of both groups increased after cooking (p<0.05). However, the gap in the in vitro protein digestibility was numerically too small to be significant, although there was a significant difference in the in vitro protein digestibility values between commercial and dry-aged beef (96.67% and 96.74%, respectively). Mora et al. (2017) have described that aging and cooking increased the intensity of small peptides in meat after digestion, and shows functionalities, such as lowering blood pressure and antioxidant effects.
Dry-aging and cooking effects on protein oxidation of beef loin are shown in Table 1. Sulfhydryl content of myofibril protein from DA was initially higher than that of myofibril protein from the control (p<0.05). Sulfhydryl contents of both groups decreased after cooking, and the interaction effect was detected (p<0.05). Sulfhydryl content of dry-aged beef was more affected than that of the control group by cooking. The disulfide bond between some proteins could be disconnected by proteolysis in dry-aged beef, making it easier to be oxidized by radical species during cooking (Hofmann and Hamm, 1978). There is a trend of the sulfhydryl and disulfide bonds increasing during the initial phase of protein oxidation (Opstvedt et al., 1984). The loss of sulfhydryl and the formation of carbonyl compounds reflected the protein oxidation status (Soyer et al., 2010). Likewise, the carbonyl content of dry-aged beef was significantly higher than that of the control groups, and the cooking process led to the formation of carbonyl content (p<0.05). The carbonyl content of the control group rapidly increased compared to dry-aged beef, which was not significantly increased, during cooking. The formation of carbonyl compounds did not increase further beyond approximately 70°C of cooking temperature (Bax et al., 2012; Gatellier et al., 2007). Watanabe et al. (2015) suggested that the carbonyl compounds, such as aldehydes, alcohol, and ketones, increased when aged beef was cooked at 180°C. In this regard, it could be speculated that the aged beef with different cooking temperature might affect protein denaturation, resulting in different patterns.
The SDS-PAGE of raw and aged beef treated with cooking is shown in Fig. 1. From the heavy myosin chain to light myosin chains (the high molecular weight to low molecular weight), the band segmentation of dry-aged beef was clearly observed. G-Actin (a molecular weight of 42 kDa) decreased in both groups with cooking, in agreement with Murphy and Marks (2000). This result agreed with that of Murphy et al. (1998), in which a decrease in the <43 kDa band was detected at the cooking temperature range of 60°C to 80°C in chicken breast patties. In this study, SDS-PAGE revealed that the protein of dry-aged beef was sensitively affected by cooking, resulting in the high protein degradation of dry-aged beef. In particular, the <20 kDa band, which might include myosin light chain-1 (MLC-1) and myosin light chain fragments, increased in the dry-aged beef. In agreement with the SDS-PAGE protein separation patterns reported by Claeys et al. (2004), a range of 3 to 17 kDa proteins in beef increased with an increasing aging time. This was evident in the degree of protein degradation in dry-aged beef and denaturation by cooking.
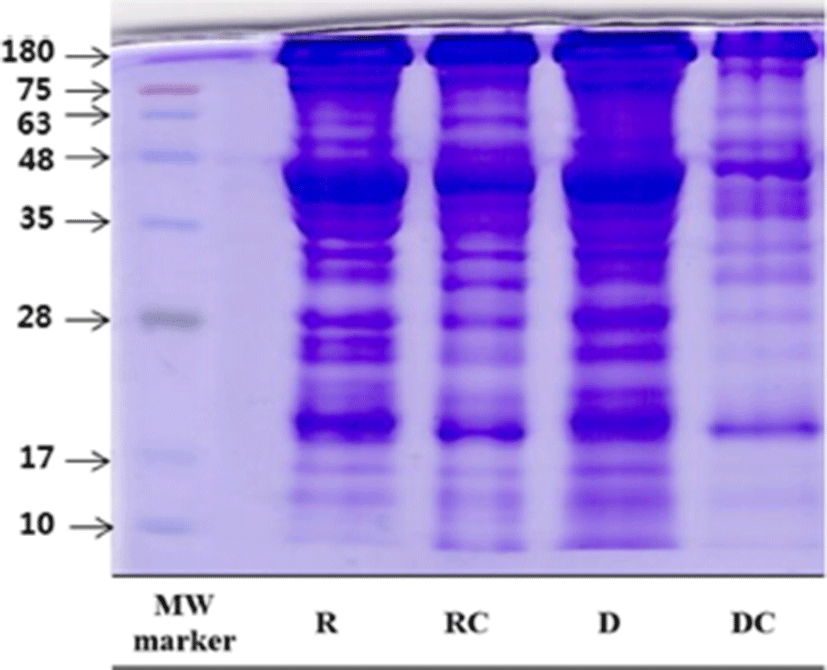
Pearson correlations among the cooking traits and protein characteristics are shown in Table 2. In terms of dimensional changes, there was a negative correlation between surface and dimension reductions (p<0.001). Shear force showed a negative correlation with the thickness reduction and a positive correlation with the surface reduction, respectively (p<0.001). Shear force had a negative correlation with carbonyl content (p<0.001). Moreover, a highly negative correlation between nile red (aggregation) and digestibility was observed (p<0.001). In addition, BPB exhibited a negative correlation with sulfhydryl content (p <0.001), and carbonyl showed a negative correlation with sulfhydryl content (p<0.001).
Conclusion
Myofibrillar protein degradation of dry-aged beef increased, with decreasing shear force compared to commercial beef, as expected. In terms of protein functionalities, the aggregation of dry-aged beef increased, while its hydrophobicity was not affected by dry-aging. Before cooking, the carbonyl content increased in dry-aged beef, but the sulfhydryl content did not show a difference between commercial and dry-aged beef. However, the protein oxidation of dry-aged beef was sensitively affected by the cooking process. Moreover, the in vitro protein digestibility of dry-aged beef was slightly improved compared to the commercial beef.