Introduction
Domestic beef consumption per capita increased from 10.2 kg in 2011 to 11.6 kg in 2016; however, the degree of self-sufficiency for beef decreased from 42.8% in 2011 to 38.9% in 2016, with an increase in imports (KMTA, 2017). In Korea, the production frequency of Hanwoo beef with quality grades 1–3 is 59.8% (KAPE, 2016). However, demand in the market is low, with low prices due to low quality for consumption. Aging technology has become essential to enhance the tenderness and flavor of meat and to meet the high demand and quality expectation for fresh beef (Smith et al., 2008). There are two aging methods, i.e., wet aging and dry aging. In the wet-aging process, wholesale primal/subprimal cuts are vacuum-packaged and stored in refrigerated conditions. In the dry-aging process, beef carcasses or primal/subprimal cuts are directly exposed to environmental conditions under strictly controlled ambient conditions, including temperature, relative humidity, and airflow, without packaging (DeGeer et al., 2009). Wet aging is commonly used in Korea owing to the easy handling and economic advantages. Dry aging is not commonly used because it places additional emphasis on environmental control practices to achieve a consistent product. The primary parameters for dry aging are drying temperature, relative humidity, aging days, and air flow. Each of these parameters influence the development of flavor, shelf-life, product shrinkage, microbial spoilage, other quality and economic properties (Savell, 2008). The drying temperature affects enzymatic processes, which in turn influence the palatability and shelf-life (susceptibility to microbial spoilage) (Warren and Kastner, 1992; Campbell et al., 2001; Smith et al, 2007; Laster et al., 2008). Relative humidity also plays a role in the growth of spoilage bacteria and product shrinkage (Parrish et al., 1991; Campbell et al., 2001; Smith et al., 2007). Aging days are related to the Warner-Bratzler shear force (WBSF) values and meat tenderness (Campbell et al., 2001; Smith et al., 2007). Several trials have been conducted on the effects of various dry aging durations, but scientific information is still limited. Air flow is important for cooling the product, allowing for uniform drying; however, it’s effects have never been investigated scientifically. A survey by Kim et al. (2015) showed that the dry aged products used in the domestic distribution companies or restaurants are mainly produced at a temperature of –1℃–4℃, relative humidity of 65%–90%, and aging period of 14–60 d. Most studies on dry aging have used storage temperatures from 0 to 4℃, relative humidity of 62%–87%, aging period of 14–62 d, and air velocity of 0.2–0.5 m/s (USMEF, 2014).
There is a growing consumer demand for different types of beef products. Retailers are constantly searching for new products to generate consumer appeal. Dry-aged beef is characterized as premium meat owing to its unique and distinctive flavor and product quality. Practitioners of dry aging strive to provide a consistent, high-quality, safe product, and the conditions used for dry aging are critical to minimize shrinkage loss, trim loss, risk of contamination, and quality deterioration (Campbell et al., 2001; Smith et al., 2008).
However, there is very limited research on dry aging of Hanwoo beef. In particular, no scientific studies have been conducted on the effects of critical parameters such as the temperature and relative humidity applied during the dry-aging on shrinkage, quality, and palatability of dry aged beef. Therefore, the objective of this study was to investigate the effects of dry aging temperature and relative humidity on the yield, microbial growth, water activity, meat quality, storage stability, and sensory properties of loin muscles from Hanwoo beef cattle.
Materials and Methods
Two treatment types comprising four dry-aging treatments (T1–T4) were used. (1) Drying at constant temperature and relative humidity: T1 (2℃, 85%, 60 d) and T4 (4℃, 85%, 60 d). (2) Drying with gradual increases in the temperature and relative humidity: T2 (initial drying at 2℃, 65% for 20 d, followed by drying at 2℃, 75% for 20 d, and final drying at 4℃, 85% for 20 d) and T 3 (initial drying at 2℃, 75% for 20 d and then at 4℃, 85% for 20 d). For the entire duration of the dry-aging process (60 d), uniform air flow at a velocity of 0.3 m/s was maintained. Fifty-four pairs of Hanwoo beef loins (6th–13th ribs, bone-in, and fat cover) (Fig. 1) from Hanwoo steer carcasses with quality grade 1 at 2 d postmortem were obtained from a local meat processing plant. They were randomly assigned to 4 groups (T1–T4) and hung in a dry-aging room to control and monitor the temperature, humidity, and air velocity (Table 1). On each sampling date, the loin muscles (m. longissimus dorsi) were deboned and trimmed the dried surface and non-edible fat for analysis of chemical composition, meat quality, sensory properties, and storage stability.
Treatment | Condition | Sampling day |
---|---|---|
T1 | 2℃, 85%, 60 d | 0, 20, 40, 60 |
T2 | 2℃, 65%, 20 d+2℃, 75%, 20 d+4℃, 85%, 20 d | 0, 20, 40, 60 |
T3 | 2℃, 75%, 20 d+4℃, 85%, 40 d | 0, 20, 40, 60 |
T4 | 4℃, 85%, 60 d | 0, 20, 40, 60 |
Changes in the weight of loin sections were calculated by obtaining the initial weight on day 0 and on each sampling day, after which samples were placed in a dry aging room. The percentage of weight loss was calculated as the difference between the initial weight and final weight divided by the initial weight.
The samples were swabbed (5 cm×5 cm area) twice on each sampling day on the surface of the retail cut using 3 MTM Quick Swabs (3M, MN, USA). Letheen broth at the top of the swab was added and the samples were plated on 3M Petrifilm. One sample was plated on a 3M Petrifilm Aerobic Count Plate and the other sample was plated on a 3M Petrifilm E. coli/Coliform Count Plate. The 3M Petrifilm Aerobic Count Plate was incubated at 35℃ for 48 h to examine the growth of mesophilic organisms, and the 3M Petrifilm E. coli/Coliform Count Plate was incubated at 35℃ for 24 h. Microbes were counted following the 3M Interpretation Guide available from http://www.3m.com/intl/kr/microbiology/p_aerobic/use3.pdf.
pH was measured after homogenizing 3 g of each sample with 27 mL of distilled water for 30 s using a homogenizer and a pH meter (Model 340; Mettler-Toledo GmbH, Switzerland). The instrument was calibrated with 3 different standard pH solutions (4.0, 7.0, and 9.25) before the measurements were obtained. The aw was determined at 25℃ using the Novasina measuring instrument (SPRINT-TH 300; Switzerland). Calibration was performed using several saturated solutions with known aw (Ba et al., 2016). pH and aw were measured in triplicate.
Protein, fat, moisture, and collagen contents were analyzed using the Food ScanTM Lab 78810 (Foss Tecator Co., Ltd., Denmark), according to the methods of the Association of Official Analytical Chemists (AOAC, 2012).
Color values were determined two times using a CR-400 Chroma Meter (Konica Minolta Sensing, Inc., Tokyo, Japan) on a freshly cut surface before and after trimming. The standards for lightness (CIE L*), redness (CIE a*), and yellowness (CIE b*) were calibrated after a 30-min blooming period at 2℃ (CIE, 1986). Water holding capacity (WHC) was determined by a centrifugation-based method (Kristensen and Purslow, 2001). The homogenized tissue (0.5 g) was placed in a 2-mL centrifugal filter unit with 5 µm of microporous membrane (BiofilⓇ, Millipore, Japan), and then placed in a 50-mL centrifugation tube, heated in a 70℃ water bath for 30 min in a polyethylene plastic bag (20 cm×20 cm), and centrifuged at 100×g (Hitachi SCR20BA, Japan) for 10 min at room temperature (ca. 18℃). WHC was expressed as the percentage weight loss in a tissue sample during centrifugation. To determine the WBSF, the meat blocks (50×50×25 mm) were cooked in a pre-heated water bath at 80℃ for 40 min until the core temperature reached 72℃, and then cooled under running water (ca. 18℃) for 30 min to achieve a core temperature below 30℃. Eight cores of 1.27 cm in diameter were prepared for each sample, and peak force was determined using a V-shaped shear blade of an Instron Universal Testing Machine (Model 5543; MA, USA) with a cross-head speed of 400 mm/min (Wheeler et al., 2000). Cooking loss (CL, %) was calculated as the percentage weight change during cooking for WBSF measurement. The samples were freshly cut into blocks and weighed (initial weight). Individual cooked meat block samples were removed from the water bath, cooled in cold water, and weighed. CL was then expressed as the percentage of the initial sample weight (Honikel, 1998).
The loin beef strips (50×75×40 mm) were cooked on a tin plate (at approximately 245℃–255℃) at National Institute of Animal Science sensory testing facility. Strips were turned at the first pooling of liquid on the surface of the sample or at the start of shrinkage. The cooked strips were immediately served to 7 trained sensory panelists for evaluation. The panelists evaluated and scored the beef samples for tenderness, juiciness, flavor, and overall acceptability. The scoring was performed using 6-point scale. Tenderness ranged from very tough (1) to very tender (6); juiciness ranged from very dry (1) to very juicy (6). Flavor ranged from dislike extremely (1) to like extremely (6); overall acceptability ranged from dislike extremely (1) to like extremely (6).
The thiobarbituric acid reactive substance (TBARS) content was measured according to the method described by Sinnhuber and Yu (1977). The beef samples (0.5 g) were mixed with ca. 0.1 g of antioxidant mixture [54% (w/w) propylene glycol-40% (w/w) Tween 20-3% (w/w) butylated hydroxytoluene-3% (w/w) butylated hydroxyanisole], 3 mL of 1% (w/v) TBA-0.3% (w/v) NaOH, and 17 mL of 2.5% (w/v) TCA-36 mM HCl, boiled at 100℃ for 30 min, and ice-cooled for 10 min. Then, 5 mL of the upper solution was transferred to a conical tube and 3 mL of chloroform was added before centrifugation at 4℃, 3,000×g (Avanti J–E Centrifuge, Beckman Coulter, Inc., Palo Alto, CA, USA) for 30 min. Absorbance values of supernatants were measured at 532 nm and converted into mg of malonaldehyde (MA)/kg of meat.
Results were analyzed using the two-way ANOVA procedure of the SAS program (2012) considering treatment and storage time as the main effects. For the sensory evaluation, the panelists and evaluation session were considered random effects and the treatment and storage time were considered fixed effects in a statistical model. The differences in means between groups were compared by using the Student–Newman–Keuls’ multiple comparison test. The significance level was set at p<0.05.
Results and Discussion
The loin muscles in four different conditions exhibited reductions in dry yield (%) after dry aging for 60 d (Table 2). T1 and T2 showed significantly higher yields than T3 or T4 only at 20 d (p<0.05); however, there was no significant difference among the 4 treatments after 40 d and 60 d. This could be related to the minimal shrinkage at low temperature and surface dehydration at the initial stage. The loin weight loss of loin during dry-aging for 60 d was <10% and the loin muscle exhibited less meat crust and cooler shrink because the vertebra (6th–13th) and subcutaneous fat cover offered protection from excess evaporation of surface moisture during the 60 d of drying. Smith et al. (2014) observed greater percentages of crust and trim loss in dry aged boneless ribeye fillet steaks than in dry aged bone-in strip loin. Laster et al. (2008) showed that steak yields are affected by cut type (generally bone-in ribeye>bone-in strip loin>boneless top sirloin).
Treatments are the same as Table 1.
The aw tended to decrease during the 60 d of the dry-aging period, but difference was not significant (p>0.05). The pH and total aerobic counts (TPC) increased during the aging period (p<0.05) (Table 3). The coliform and E. coli counts were <1 CFU log/cm2 over all aging periods for all four treatments (data not shown). Lee et al. (2015) showed that dried Hanwoo strip loin has a higher pH than that of non-dried fresh strip loin muscles when dried at 2℃ for 21 d (p<0.05). The increase in pH might be due to the nitrogenous compounds from the proteolysis during the aging period (Obuz et al., 2014). DeGeer et al. (2009) reported that total aerobic plate counts increased from 2.89 to 3.51 Log CFU/cm2 for bone-in shell loins during 28 d of dry aging. In this study, total aerobic plate counts increased from 1.68 to 3.86 Log CFU/cm2 for 4 different treatment conditions during 60 d of dry aging. Among the four treatments, T1 had significantly higher TPC than the other conditions (T2–T4) at 20, 40, 60 d (p<0.05). During the 60 d of dry aging, products form T2 and T3, which involved gradual increase in temperature and humidity (2℃ to 4℃, 65%–85%) showed lower TPC at 20, 40, 60 d, with lower aW at 60 d than those from T1 and T4 aging at constant temperature (2℃ or 4℃) and relative humidity (85%). This could be related to the difference in the surface dehydration at different temperature and relative humidity. Ryu et al. (2018) observed a significant increase in total bacteria, with an absence of representative foodborne pathogens during dry aging for 40–60 d in a refrigerated room with a relative humidity of 75%–80% and air-flow.
Treatments are the same as Table 1.
The protein, moisture, fat, and collagen contents of the four Hanwoo steer beef muscles are shown in Table 4. During the dry-aging period, only the moisture content (%) of loin muscle decreased significantly (p<0.05); the contents of protein and collagen did not change significantly during aging for all four treatments (p>0.05). Only T1 exhibited a higher moisture content than those of the other treatments on 20, 40, and 60 d of drying. Hwang et al. (2010) demonstrated that the moisture content of Hanwoo steer muscle was inversely related to the lipid content. In this study, the fat contents increased significantly as the moisture content decreased during the aging period (p<0.05). The fat contents increased from 8.79–10.64% at 0 d to 10.54%–15.06% at 60 d in loin muscles for the four treatments. Dashdorj et al. (2016) reported that one advantage of dry aging is the increase in the concentration of taste-related compounds due to moisture evaporation. Several researchers have reported that the moisture dehydration and chemical breakdown of proteins and fats during the dry aging process impart an intense nutty and beefy flavor to the aged product, improving its palatability and flavor (Campbell et al., 2001; Kim et al., 2016; Lida et al., 2016). Colle et al. (2015) reported that there were no differences in soluble and insoluble collagen between aging periods for loin muscle.
Treatments are the same as Table 1.
In a meat color analysis, before the vertebra and fat cover were trimmed, CIE L*, a*, and b* values for the outside of meat decreased significantly as the aging time increased for all treatments (p<0.05) (Table 5). At day 20, T1 had higher CIE L*, a*, and b* values than those of the other treatments (p<0.05). However, there were no significant differences in CIE L*, a*, and b* values inside of loin muscles among aging durations for all treatments after vertebra and fat cover were trimmed (p>0.05). Colle et al. (2015) reported that the oxygenated lean meat became darker and the color uniformity became less desirable with longer aging and retail display periods. Li et al. (2013) reported that dry-aged beef had higher contents of Met-myoglobin than those of wet-aged beef. However, in this study, the loin muscles had a stable color and were protected from denaturation by exposure to air during the dry aging periods due to the vertebra and subcutaneous fat cover. Kim et al. (2016) reported that dry aging did not adversely impact surface color or water holding capacity after the outer crust and fat were trimmed for the retail display of dry-aged beef steaks.
Treatments are the same as Table 1.
Cooking loss (%) did not differ significantly among aging days for all four treatments (Table 5). T1 and T4 showed significantly greater cooking losses (%) than those of the other treatments on day 60 (p<0.05). Li et al. (2013) reported that there was no significant difference in cooking loss between dry-aged beef and wet-aged beef at 3℃ for 21 d.
The WBSF values for all treatments decreased significantly as the aging period increased (p<0.05) (Table 5). Among the four treatments, there were no significant differences in WBSF force values. Tenderness is one of the most important quality attributes of beef (Devitt et al., 2002). Rhee et al. (2004) reported that protein patterns and degradation rates of structural proteins, such as titin, nebulin, and filamin, vary during refrigerated storage for 28 d. Fast degradation of these myosin family proteins is a key factor for the improvement of meat tenderness. Bechtel and Parrish (1983) reported that more tenderization occurs at an elevated temperature due to enhanced proteolytic enzyme activity. However, Kim et al. (2016) reported that dry-aged loin at 1℃ showed similar shear force values to those of dry-aged loin at 3℃.
The WHC (%) increased as the aging period increased for 4 treatments (Table 5). This could be related to the increase in the pH of the meat during the dry-aging period. When the pH approaches the isoelectric point of myofibrillar proteins (pH 5.2), there is an overall reduction in the reactive groups on the proteins that are available for water holding, thereby resulting in loss of protein solubility. The higher WHC values are associated with the greater net charge on the protein and the greater contents of bound or immobilized water at pH 5.4–6.8. At 60 d, the high pH of T2 and T3 (5.76, 5.61; Table 3) resulted in a significantly higher WHC than those of the other treatments (p<0.05).
The changes in TBARS values increased significantly as the duration of dry aging increased (p<0.05) (Fig. 2). The dry aging of loin muscle in four different conditions resulted increased lipid oxidation as the aging period increased (p<0.05). Lee et al. (2015) reported that dried Hanwoo strip loin had higher TBARS values than those of fresh strip loin muscles (p<0.05) when dried at 2℃ for 21 d. Lipid oxidation is associated with an undesirable off-flavor and discoloration (Gray et al., 1996). Faustman and Cassens (1990) indicated a close relationship between lipid oxidation and myoglobin oxidation. The increase in lipid oxidation and the likely increase in myoglobin oxidation presumably played a role in the discoloration of steaks over the retail display time (Faustman, et al., 2010). In this study, the TBARS values were as low as <0.5 mg of malondialdehyde/kg of sample after 60 d of dry aging. The overall TBARS values were low because the vertebral bone and fat cover in bone-in shell loins protected the loin muscle inside from exposure to air and lipid oxidation during the dry aging. DeGeer et al. (2009) reported that dry-aged bone-in shell loins had lower TBARS values than those of boneless striploin when they were dry-aged for 28 d. They also found that the higher TBARS values did not negatively affect sensory flavor and some lipid oxidation may be associated with the development of the characteristic dry-aged flavor.
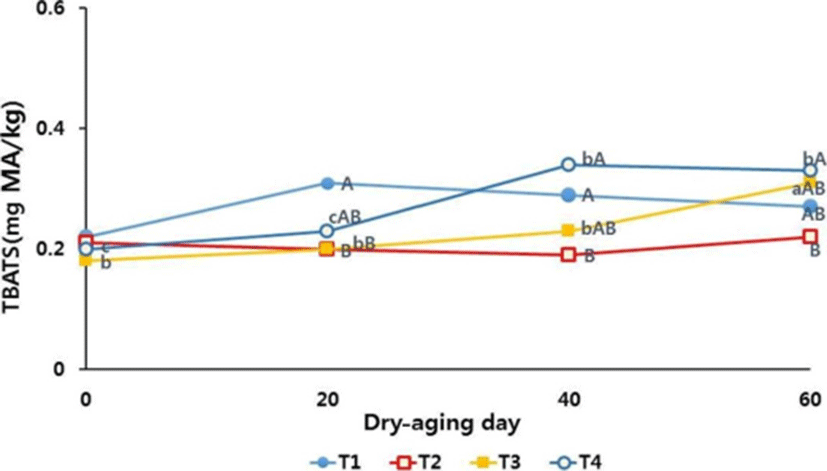
In a sensory evaluation for the four dry-aging conditions, the tenderness, juiciness, flavor, and overall acceptability scores of loin muscles increased as the duration of aging increased (Table 6). Among the four treatments, there were differences in the tenderness scores of loin muscle at 20, 40, and 60 d and juiciness, flavor, and overall acceptability scores at 40 d, and 60 d (p<0.05). T2 and T3 had significantly higher sensory scores and T1 had significantly lower scores for tenderness, juiciness, flavor-likeness, and overall acceptability scores at 20, 40, and 60 d (p<0.05). Significant improvements in sensory tenderness have been observed after meat is dry-aged from 14 d to 35 d (Campbell et al., 2001; Laster et al., 2008; Smith et al., 2008). Additionally, the dry-aged steaks received equal or higher scores than those of wet-aged steaks for juiciness, despite the greater evaporative moisture loss. Lee et al. (2015) reported that dried Hanwoo striploins were preferable with respect to texture, taste, flavor, and overall acceptability than fresh striploin muscles when dried at 2℃ for 21 d (p<0.05). Lida et al. (2016) showed that the contents of glutamic acid and 5'-inosine mono phosphate (5'-IMP) were highest at 40 d of dry-aged Japanese beef during aging for 60 d at 1℃ to 4℃ and the umami intensity was also highest in the sensory evaluation due to the synergistic effect of umami compounds. Enhanced flavor, along with other palatability improvements, contribute to the perception of dry-aged beef as a premium product that commands a higher price in the marketplace (Laster et al., 2008). Dry-aged beef has a distinctive flavor that contrasts with the “wet-aged” flavor of beef. Wet-aged beef has a sour and strong bloody/serumy flavor, whereas dry-aged beef has a beefy, brown/roasted flavor that is considered desirable (Laster et al., 2008; Warren and Kastner, 1992). Kim et al. (2016) reported that many metabolites associated with taste are more abundant in dry-aged beef than in wet-aged beef; this may explain the intensified flavor of dry-aged beef in sensory evaluations. A metabolite analysis showed that 7 metabolites, including several flavor precursors, were more abundant in the dry-aged beef than in the wet-aged beef. Ryu et al. (2018) isolated fungi, such as Penicillium camemberti and Debaryomyces hansenii, which are used for cheese manufacturing, from dry-aged Hanwoo beef and these fungal taxa may be involved in the palatability and flavor development of dry-aged beef.
Treatments are the same as Table 1.
Tenderness, very tough (1) to very tender (6); juiciness, very dry (1) to very juicy (6); flavor, dislike extremely (1) to like extremely (6); overall acceptability, dislike extremely (1) to like extremely (6).
Conclusions
Different dry-aging conditions, including the use of a constant temperature and humidity (T1, T4) or use of gradual increases in temperature and humidity (T2, T3), exerted markedly different effects on the drying yield, microbial growth, storage stability, WBSF, WHC, and sensory characteristics of the Hanwoo beef loins. Further studies of dry-aged products are needed to establish an optimal dry-aging process that is economically feasible for the end processor while still producing high-quality meat.