Introduction
In 2015, 883,593 heads of Hanwoo beef cattle were slaughtered in Korea for meat production purposes (KAPE, 2016). Korean consumers prefer highly marbled beef, especially from Hanwoo cattle, and the prevalence of beef with the quality grade (QG) of 1 is 65% (QG 1, 31.4%; QG 1+, 26.4%; and QG 1++ 10.1%) (KAPE, 2016). Hanwoo beef with QG 1 has been exported to Hong Kong since December 2015. The export has continuously increased to ca. 44 tons in December 2016; currently, the export is expected to expand to Macao (Kwon et al., 2016). According to Kwon et al. (2016), the meat consumption trend in China has gradually changed from pork to other meats, such as beef and chicken, over the last 20 years. Specifically, beef production has been continuously increasing annually by 3.9%, from 36,400 MT in 1993 to 77,600 MT in 2013. With this increase in beef consumption, the demand of the metropolitan consumer for imported high-quality brand beef or highly marbled beef has also increased. Popular beef dishes, e.g., Shabu-Shabu, Korean barbecue, and steak, have diversified under the influence of foreign countries. However, the maintenance of meat quality over distribution durations and distances is challenging. Most meat is distributed as frozen products, as the cold chain supply system is not commonly employed in China at present. In Hong Kong, 134,000 tons (99% of the total meat consumption) of beef are imported, as the domestic production of beef in this country is only 8 tons. The consumption of beef has gradually increased annually, by 4.86% on average, in the last 18 years. Beef consumption in Hong Kong is 21.5 kg per capita, which is 1.4 times higher than that in South Korea and 2.4 times higher than that in Japan (Kwon et al., 2016). The beef market in Hong Kong is divided into two sectors: midlow quality meat and high-quality meat. Consumers who prefer mid-low quality beef focus on the price, whereas those who prefer high-quality beef focus on meat quality, regardless of the price. For the latter group, marbling is an important factor when choosing beef. These consumers purchase premium beef brands, such as Japanese Wagyu beef, in restaurants and supermarkets, at high prices. Like Wagyu beef, Hanwoo beef is characterized by high marbling and high meat quality, and is comparable with the former on the international beef market (Kwon et al., 2016).
Meat aging improves the tenderness and/or meat water-holding capacity (WHC) by allowing degradation of the cytoskeletal myofibrillar proteins during cold storage. Freezing is an effective method to extend the shelf life of meat. Although several studies have investigated the effect of aging, freezing, and thawing on meat quality attributes, the combined effect of aging and freezing on meat quality characteristics has not been determined. The objective of this study was therefore to establish the shelf life of 1++ grade Hanwoo beef for export targeted at consumers who prefer highly marbled beef. The changes in meat quality during cold aging and freezer storage were evaluated under conditions similar to those during distribution of meat to China or Hong Kong.
Materials and Methods
Fresh 1++ grade loin, striploin, tenderloin and top round muscles from 10 Hanwoo steers were purchased on the day after slaughter, from the Nonghyup Pyeongchang branch of Kangwondo. The meat was vacuum-packaged and aged for 0, 7, and 14 d at 2°C, and continuously stored for 0, 3, 6, or 9 mon at −18°C. For composition and quality analysis, samples were collected on each day of storage and analyzed.
Protein, fat, moisture, and collagen contents were analyzed using the Food ScanTM Lab 78810 (Foss Tecator Co., Ltd., Denmark), according to the methods of the Association of Official Analytical Chemists (AOAC, 2016).
Color values were determined using a CR-400 Chroma Meter (Konica Minolta Sensing, Inc., Japan) on a freshly cut surface. The standard for lightness (CIE L*), redness (CIE a*), and yellowness (CIE b*) was calibrated after a 30-min blooming period at 2°C (Commission Internationale de I’Eclairage, 1986). WHC was determined by a centrifugation-based method (Kristensen and Purslow, 2001). The homogenized tissue (0.5 g) was placed in a 2-mL centrifugal filter unit with 5 μm of microporous mem brane (Biofil®, Millipore, Japan), and then placed in a 50-mL centrifugation tube, heated in a 70°C water bath for 30 min, and centrifuged at 100 × g (Hitachi, SCR20BA, Japan) for 10 min at room temperature (ca. 18°C). WHC was expressed as the percentage of weight loss in sample tissue during centrifugation. For determination of Warner-Bratzler shear force (WBSF), the meat blocks (50 × 50 × 25 mm) were cooked in a pre-heated water bath for 40 min until the core temperature reached 80°C, and then cooled under running water (ca. 18°C) for 30 min to achieve a core temperature below 30°C. Eight cores of 1.27-cm diameter were prepared for each sample, and peak force was determined using a V-shaped shear blade of an Instron Universal Testing Machine (Model 5543, USA) with a cross-head speed of 400 mm/min (Wheeler et al., 2000). Cooking loss (CL, %) was calculated as the percentage of weight change during cooking for WBSF measurement. The samples were freshly cut into blocks and weighed (initial weight). Individual cooked meat block samples were removed from the water bath, cooled in cold water, and weighed. CL was then expressed as the percentage of the initial sample weight (Honikel, 1998).
TBARS content was measured according to the method described by Sinnhuber and Yu (1977). The beef samples (0.5 g) were mixed with ca. 0.1 g of antioxidant mixture [54% (w/w) propylene glycol-40% (w/w) Tween 20-3% (w/w) butylated hydroxytoluene-3% (w/w) butylated hydroxyanisole], 3 mL of 1% (w/v) TBA-0.3% (w/v) NaOH, and 17 mL of 2.5% (w/v) TCA-36 mM HCl, boiled at 100°C for 30 min, and ice-cooled for 10 min. Then, 5 mL of the upper solution was transferred to a conical tube and 3 mL of chloroform was added before centrifugation at 4°C, 3000 × g (Avanti J-E Centrifuge, Beckman Coulter, Inc., CA) for 30 min. Absorbance values of supernatants were measured at 532 nm and converted into mg malonaldehyde (MA)/kg meat.
VBN content was analyzed according to the Korea Food Standards Codex (2017) of MFDS. The sample (10 g) was homogenized in 20 mL of deionized water (DW), using a Polytron device (PT-MR 2100, Kinematica AG, Switzerland) with the speed set at 10,000 rpm, for 1 min. The suspension volume was brought up to 50 mL with DW, and then filtered through Whatman filter paper number 1. Borate buffer [1 mL, 1% (w/v] containing 6.6 ppm of methyl red and 3.3 ppm of bromocresol green was placed in an inner section of a Conway dish, and the filtrate was then transferred to the outer section of the same dish. Saturated K2CO3 (1 mL) was poured into the outer section and the dish was incubated at 37°C for 120 min. The green-colored solution in the inner section was then titrated with 0.01 N H2SO4. VBN content was calculated as mg% of ammonia, using the following formula: 0.14 × titration volume of sample (mL) × factor of 0.01 N H2SO4 × dilution factor × [100/sample weight (g)].
Each animal within the same slaughtering age group was treated as a replicate. Data were analyzed by the Student-Newman-Keuls’ multiple comparison test, using the GLM procedure of the SAS program (2015). The significance level was set at p<0.05.
Results and Discussion
The protein, moisture, fat, and collagen contents of the four Hanwoo steer beef muscles are shown in Table 1. The protein content (%) in the loin, striploin, tenderloin, and top round muscles was between 17.72 and 19.26%, with no significant difference between the four muscles (p>0.05). The moisture contents of the tenderloin (63.41%) and top round (62.96%) muscles were significantly higher than that of striploin muscle (57.27%) (p<0.05). Intramuscular fat (IMF) content was highest in the striploin muscle (22.64%), and lowest in the top round muscle (12.42%) (p<0.05). The collagen contents of the loin (2.04%) and striploin (2.01%) muscles were higher than that in the top round muscle (1.66%) (p<0.05) (Table 1).
*Mean±SE.
a-cMeans in the same row within the same category with different letters are significantly different (p<0.05)
Canto et al. (2016) showed that muscle source did not affect the protein or ash content. Similarly, Mitterer-Deltoe et al. (2012) reported that the protein content of beef muscles generally exhibits low variability. Hwang et al. (2010) demonstrated that the moisture content of Hanwoo steer muscle was inversely related to lipid content. Several groups have reported that IMF is important because of its positive effect on eating quality traits such as tenderness, juiciness, and flavor (Hocquette et al., 2010). Jeremiah et al. (2003a) also suggested that fat and moisture contents are correlated with the juiciness of beef. IMF deposited between the fasciculi disrupts the honeycomb structure of the endomysium and separates the perimysial fibers, thereby increasing tenderness. To ensure consumer acceptability, a minimum level of 5% IMF in grilled cuts of red meat, such as beef and lamb, was recommended by Hopkins et al. (2006).
Collagen content affects beef tenderness. In the study by insoluble collagen was more closely associated with toughness in palatability traits than total or soluble collagen when bovine muscle is cooked (Dutson, 1974; Herring et al., 1967; Jeremiah et al. 2003b). Collagen with few intermolecular cross-likages is converted to gelatin, which improves tenderness, but when more intermolecular cross-links are present, moisture is squeezed out of the muscle, decreasing tenderness (Ledward, 1984). In this study, the insoluble collagen levels of the tenderloin, striploin and loin in this study might be relatively lower than top round muscle, although their total collagen levels in raw meat are higher than that of top round muscles. In addition, the top round has been reported to contain heavy deposits of coarse elastin fibers and they also contribute to meat toughness to about the same extent as denatured collagen (Bendall, 1967).
Most consumers prefer high-quality meat for sensory satisfaction. The quality of raw meat is generally evaluated by assessing the color, WHC, texture, and palatability (Harris et al., 2012; Muhlisin et al., 2014). The meat color (CIE L*, a*, b*) of the loin, striploin, tenderloin, and top round muscles did not significantly change during the aging period; the lightness (CIE L*) values of the tenderloin muscles decreased as the freezer storage period increased (p<0.05) (Table 2). However, most muscles showed no significant changes in redness (CIE a*) and yellowness (CIE b*) values during aging and freezer storage. Color is the most critical quality trait considered during the purchase of fresh beef (Suman et al., 2014). The bright cherry-red color that is attractive to a consumer has been often associated with freshness of meat, whereas brownish discoloration is perceived as indicative of spoilage, leading to product rejection (Suman et al., 2014). Several studies demonstrate that beef color stability is a highly muscle-specific trait (Jeong et al., 2009; Joseph et al., 2012). Joseph et al. (2012) reported that psoas major muscle is a color-labile beef muscle, whereas longissimus thoracis et lumborum muscle is color-stable. These two muscles differ with respect to surface color, metmyoglobin reducing activity, oxygen consumption rate, and lipid oxidation.
*Mean±SE.
a,bMeans in the same cuts among the aging d within the same category with different letters are significantly different (p<0.05)
A,BMeans in the same cuts among the freezing mon within the same category with different letters are significantly different (p<0.05)
WHC, CL, and WBSF of the loin, striploin, tenderloin, and top round muscles during aging and freezer storage are compared in Table 3. The WHC values were increased when four muscle were aged for 14 d in tenderloin, striploin and top round muscles at 3 mon of freezer storage and loin muscle at 3 and 6 mon of freezer storage (p<0.05). However, the WHC values of the four muscles from each aging day did not significantly differ after the 0 to 9 mon of freezer storage.
*Mean±SE.
a,bMeans in the same cuts among the aging d within the same category with different letters are significantly different (p<0.05)
A,BBMeans in the same cuts among the freezing mon within the same category with different letters are significantly different (p<0.05)
CL (%) values after 14 d of aging and 9 mon of freezer storage were as follows: 22.68-28.42% for the loin, 20.49-27.56% for the striploin, 24.99-30.49% for the tenderloin, and 23.32-30.20% for the top round muscle. In the four muscles, these values were significantly increased as the freezer storage period increased (p<0.05); however, they were not significantly different after 0, 7, or 14 d of aging (p>0.05). Only in the case of the striploin muscle, CL values were higher after 6 mon freezer storage with 7 and 14 d of aging than with 0 d of aging (p<0.05).
With the exception of the tenderloin, the WBSF values of the other muscles in this study decreased significantly as the aging period increased (p<0.05). The WBSF value of the tenderloin muscle changed very little (2.21-2.60 kg) during the 14 d aging period. There were no significant differences in WBSF values during the 9-mon freezer-storage period after 0, 7, and 14 d of aging.
Tenderness is one of the most important quality attributes of beef (Devitt et al., 2002). Fernandez et al. (1999) reported that the high IMF levels are favorable effect on tenderness. Wheeler et al. (2005) determined that a significant negative correlation exists between IMF vs. WBSF, and positive correlation exists between IMF vs. tenderness, juiciness, and flavor scores, respectively. In addition, Rhee et al. (2004) reported that protein patterns and degradation rates of structural proteins such as titin, nebulin, and filamin, vary during refrigerated storage for 28 d. Fast degradation of these myosin family proteins is a key factor for the improvement of meat tenderness.
Freezer storage has many benefits; it inhibits oxidation, delays bacterial replication, retains meat flavor, and is particularly useful for long-distance transport (Lawrie and Ledward, 2006). However, ice crystals formed by freezing lead to desiccation of cell membrane proteins in animal muscles (Love, 1966). This increases the effluence of water-soluble nutritional components and textural deterioration, and decreases WHC (Cho et al., 2014; Lagerstedt et al., 2008). In other words, freezing causes decline of the quality of meat. Kim et al. (2011) reported that quality defects in frozen/thawed meat may be minimized by aging meat prior to freezing. Several studies have supported the notion that employing aging prior to freezing results in meat quality attributes, such as tenderness, WHC, drip loss, and/or color stability, that are better than, or equivalent to, those of aged-only beef and lamb meat (Choe et al., 2016; Kim et al., 2015; Wiklund et al., 2009). However, in this study, CL (%) increased as the freezer-storage period increased. According to Rhee et al. (2004), CL of longissimus dorsi (LD) is lower than that of semitendinosus (ST). These results were, in general, consistent with those of the current study.
The changes in VBN and TBARS values significantly increased with the increasing duration of aging and freezer storage (p<0.05) (Fig. 1). The VBN values for the four muscles were between 16.67 and 18.49 mg% after 14 d of aging and 9 mon of freezer storage. These values were close to the limit for packaged meat suggested by MFDS (2014), i.e., VBN less than 20 mg%. The TBARS values of the four muscles were between 0.75 and 0.82 mg MA/ kg meat after 14 d aging and 9 mon freezer storage (Fig. 2). When defining the shelf life of QG 1++ Hanwoo beef, the distance and facility of distribution for export to China and Hong Kong should be considered.
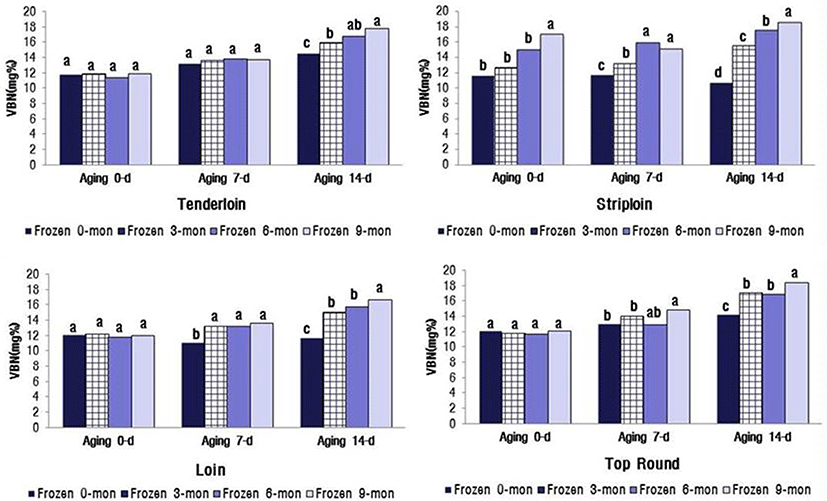
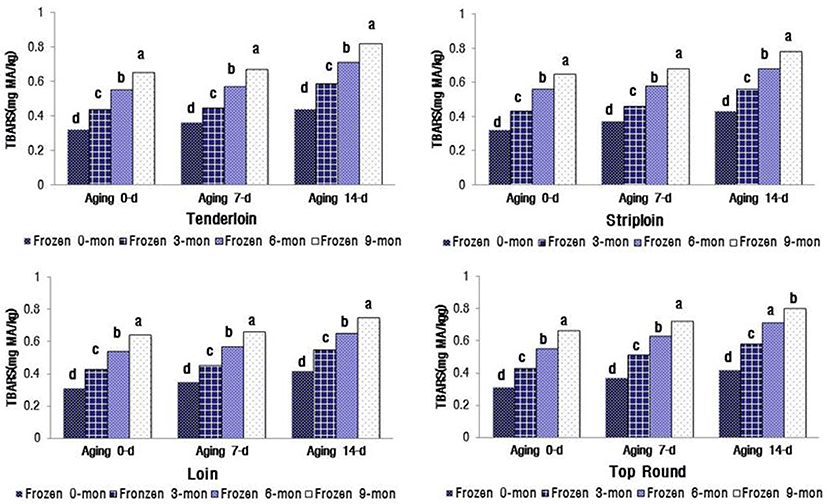
Lipid oxidation in stored meat, which is associated with undesirable off-flavor, toxic substances, and discoloration, is a principal contributing factor to the decline in meat freshness and quality (Gray et al., 1996). Protein oxidation is associated with protein carbonylation, fragmentation, or polymerization (Xiong, 2000). This process decreases WHC and sensory qualities, e.g. juiciness and tenderness, of meat. Lipid oxidation-induced myoglobin oxidation leads to accumulation of metmyoglobin and subsequent surface discoloration (Faustman et al., 2010). Insani et al. (2008) reported that beef discoloration is accelerated by the oxidation of lipids and proteins. Furthermore, several other studies have demonstrated that lipid oxidation and protein oxidation compromise the color stability of fresh meat (Faustman et al., 2010; Lund et al., 2011). In postmortem oxidative muscle, mitochondrial metabolism surpasses the myoglobin’s ability to bind oxygen, leading to accelerated metmyoglobin formation and surface discoloration (King et al., 2010). The myoglobin content (Hwang et al., 2010; King et al., 2011) and mitochondrial concentration are greater in oxidative muscles, such as the tenderloin (PM), than in glycolytic muscles such as the striploin (LL). PM contains a greater proportion of fiber type I than II (Hwang et al., 2010), which is positively correlated with myoglobin content. Furthermore, an increased level of myoglobin in the muscle is reportedly associated with low color stability (King et al., 2010). In general, color-stable muscles, such as LL, exhibited lower TBARS than their color-labile counterparts, such as PM, during 5 d of display. In contrast, Jeong et al. (2009) reported that there was no difference in TBARS between LL and PM muscles from Hanwoo bulls during 7 d storage. The results of the current study are similar to the findings of Jeong et al. (2009).
Conclusions
The current study was conducted to determine the shelf life of 1++ grade Hanwoo beef during distribution by evaluating changes in meat quality and storage stability under distribution conditions for export to Hong Kong or China. The results of this study revealed that the WBSF parameter significantly decreased with increasing aging time. As the freezer storage period increased, the CIE L* values decreased, whereas CL (%) and WHC (%) increased (p<0.05). In addition, protein and lipid oxidation were significantly enhanced when the duration of storage increased. In particular, the VBN limit of the packaged meat should be less than 20 mg%. On the basis of these observations, we conclude that the shelf life of 1++ grade of Hanwoo beef during distribution should be no longer than 9 mon of freezer storage at −18°C after aging for 14 d at 2°C.