Introduction
As the world population grows, meat consumption per capita also increases (Alexandratos and Bruinsma, 2012; van Huis et al., 2013). Meat is an important protein source in the human diet because it contains adequate and balanced amino acid composition (van der Weele et al., 2019; Wu, 2022). However, it is challenging to meet the demand for meat (animal protein) due to climate change and the reduction of agricultural land (Abbasi and Abbasi, 2016; Premalatha et al., 2011; van Huis and Tomberlin, 2017). Therefore, there is a need to use an alternative protein source that can replace meat to meet the growing demand for proteins.
Protein is an essential macronutrient that must be obtained from animals (meat, dairy, etc.) and vegetables (legumes, etc.). Adequate intake of protein is necessary for health (WHO, 2007; Wu et al., 2014). Edible insects are often proposed as a substitute for animal proteins because they are known to be high in protein, more than 50% (Beets, 1997; Bukkens, 1997). Edible insects provide protein and energy and are high in monounsaturated fatty acids and polyunsaturated fatty acids. They are also rich in micronutrients, such as copper (Cu) and iron (Fe; Rumpold and Schlüter, 2013). They produce less greenhouse and ammonia gas as well as with efficient land use, and productive yield (Klunder et al., 2012; Oonincx et al., 2010). As a result, edible insects show great potential to be used as an environmentally friendly future food resource (Akhtar and Isman, 2018).
It has been reported that 10 species of insects are consumed in Korea and more than 1,900 species of insects are consumed worldwide (Lange and Nakamura, 2021). The representative insects that are eaten in Korea include Tenenbrio molitor L., Protaetia brevitarsis seulensis L., Gryllus bimaculatus and Allomyrin dichotoma L. T. molitor L. is a type of Carabidae that is cultivated all over the world. It is mainly used as an animal feed or protein supplement because it is rich in protein and essential fatty acids (Kim et al., 2021; van Broekhoven et al., 2015). P. brevitarsis seulensis L. is a phytophagous insect belonging to Cetoniinae and has traditionally been used to treat inflammation, breast cancer, and liver disease. Its larvae have been proven to have various physiological effects such as antioxidant and antibacterial effects (Choi et al., 2019; Song et al., 2017; Suh and Kang, 2012; Yoon et al., 2003). G. bimaculatus belongs to Orthoptera and has the highest protein content and unsaturated fatty acid (UFA) content (Churchward-Venne et al., 2017; Wang et al., 2004). A. dichotoma L. belongs to Scarabaeidae and has anti-tumor, anti-hepatic fibrosis, and antibacterial effects (Miyanoshita et al., 1996; Sagisaka et al., 2001; Yoshikawa et al., 1999).
This study aimed to determine the physicochemical and antioxidant properties and storage stability of pork patties added with edible insect powder (EIP) of four species. The results of this study can pave a fundament for the future development of meat products with EIPs as protein substitutes.
Materials and Methods
Freeze-dried edible insects of four species (T. molitor L., P. brevitarsis seulensis L., G. bimaculatus, A. dichotoma L.) were provided by Chungcheongbukdo Agricultural Research and Extension Services. Edible insects were ground for 1 min using a mixer (Hanilelec, Cheongju, Korea) to prepare T. molitor L. powder (TMP), P. brevitarsis seulensis L. powder (PBP), G. bimaculatus powder (GBP), and A. dichotoma L. powder (ADP). The EIPs that were ground were stored frozen at –50°C until use. Minced pork (Manpyeong Livestock Products, Cheongju, Korea), ice, salt (Beksul, Haenam, Korea), pepper (Ottogi, Anyang, Korea), sodium tripolyphosphate (Samchun Chemical, Peongtak, Korea), and ascorbic acid (ES Food, Gunpo, Korea) was used to manufacture pork patties.
One mL of 95% methanol and 10 g of EIP were mixed. After stirring for 3 h in a constant temperature water bath at 37°C, the extraction was filtered using Whatman No. 2 filter paper (Advantec®, Tokyo, Japan). Three replications were carried out.
Reversed-phase (RP) high-performance liquid chromatography (HPLC) analysis was performed using a 4.6×250 mm RP spherisorb ODS2 column based on the method of Dimitrova et al. (2007) with minor modifications. Chromatographic analysis was performed using a Young Lin HPLC. The 20 mM potassium dihydrogen phosphate buffer (pH 2.92) was used as mobile phase A and methanol was used as mobile phase B. Elution was started with 3% methanol and allowed to reach 100% methanol in 65 min. The flow rate was at 1.0 mL/min. Phenol content was monitored at 220 nm and 280 nm. A total of seven phenolic acids (gallic acid, vanillic acid, caffeic acid, syringic acid, p-coumaric acid, phenylacetic acid, and benzoic acid) were determined with authentic standards.
Pork patties were prepared by mixing pork meat with EIPs at the ratio as shown in Table 1. Six types of pork patties were prepared with the addition of EIPs (T20, P20, G20, A20; respectively 20%), positive control (PC, 0.1% ascorbic acid), or negative control (NC, without any addition). Equal amounts of ice, salt, pepper, and sodium tripolyphosphate were added to all treatments. Finished patties were divided into 100 g each and molded with a molding machine (diameter of 10 cm, thickness of 1 mm). Manufactured patties were aged for 24 h in refrigeration at 4°C. Samples were wrapped and stored at 4°C for 7 days and evaluated on days 1, 3, and 7.
Proximate composition was measured following AOAC (1990) methods. Water content was determined with a conventional oven at 105°C. Protein content was quantified with Kjeldahl method. Fat content was examined with Folch extraction method. Ash content was determined with a Muffle oven. The carbohydrate content was determined by subtracting the sum of moisture content, protein content, fat content, and ash content from the total sum of 100%.
Sample (5 g) was mixed with 50 mL of distilled water and homogenized with a stomacher (Stomacher® 400 Circulator, BioMed, London, UK) at 200 rpm for 30 s. Then, the pH of the mixture was determined with a pH meter (Orion STAR A211, Thermo Fisher Scientific, Waltham, MA, USA).
Water holding capacity (WHC) of sample was determined with a centrifugation method. After weighing 0.5 g of sample and placing the sample in an upper filter tube of a centrifuge tube (Union 55R, Hanil Science, Daejeon, Korea), the filter tube and sample were subjected to heating in a water bath at 80°C for 20 min. Following heating, the mixture was cooling at room temperature for 10 min. The filter tube was then placed at the bottom of the centrifuge tube and centrifuged at 447×g for 10 min. The upper filter tube was removed and weighed. The WHC value was calculated using the following formula:
After vacuum packaging, the sample was placed in a 70°C water bath and heated for 40 min. Water on the surface of the heated sample was wiped off, and the weight of sample was measured. The weight difference before and after heating was used to calculate the cooking loss (CL) with the following formula:
The center portion of the surface of the uncooked patties was measured. Color parameters of CIE L*, CIE a*, and CIE b*-value were measured with a spectrocolorimeter (CM-26d, Konica Minolta, Tokyo, Japan) in the standards set of Commission Internationale de l’Eclairage (CIE). The average of measured values was obtained and recorded.
To evaluate texture profile analysis (TPA), a rheometer (Model Compac-100, Sun Scientific, Dobbs Ferry, NY, USA) equipped with a probe (No. 3, ϕ20 mm) of area 3.14 cm2 was used. The sample size was 1 cm3 and two compression cycles were used to obtain the force versus time curve. The table speed was 60 mm/min, the crosshead speed was 200 mm/min, and the load cell was 2 kg (max. 4 kg). TPA is expressed as hardness, springiness, cohesiveness, chewiness, and gumminess.
Sensory evaluation was performed with ten trained panelists (male and female, age range 20–29) in the Department of Animal Science at Chungbuk National University. Color, flavor, juiciness, umami, hardness, texture, and overall preference were measured. Sensory scores were assessed on a 5-point scale (1=extremely bad or undesirable, and very weak, and 5=extremely good or desirable, and very strong). The approved consent procedure for sensory evaluation is Institutional Review Board (IRB) of Chungbuk National University (No. CBNU-202302-HR-0017).
A stomacher bag containing 5 g of the sample was combined with 45 mL of a 0.1% peptone solution. The mixture was homogenized in a stomacher (Stomacher® 400 Circulator, BioMed) at 200 rpm for 30 s. After serially diluting the homogeneous sample, it was inoculated into a plate count agar medium (Microgiene, Suwon, Korea) and incubated at 37°C for 48 h. The number of microorganisms was determined using a colony counter. It is expressed as Log CFU/g.
Sample (10 g) was combined with cold 10% perchloric acid 15 mL and tertiary distilled water 25 mL using a homogenizer (AM-7, Nissei, Izumichom, Tokyo). After homogenization at 10,000 rpm for 15 s, the homogenate was filtered using Whatman No. 2 filter paper (Advantech, Tokyo, Japan). Subsequently, 5 mL of 0.02 M thiobarbituric acid solution was mixed with 5 mL of the filtrate (5 mL of tertiary distilled water for blank). The mixture was then kept in a cool, dark location for 16 h. Absorbance at 529 nm was then measured using a spectrophotometer (DU-650, Beckman, Brea, CA, USA). Absorbance was converted to malonaldehyde content using a standard curve. The resulting thiobarbituric acid reactive substance (TBARS) level was expressed as mg malonaldehyde per 1,000 g of the sample (mg MDA/kg).
One gram of minced sample added into an Erlenmeyer flask. Then, 10 mL of chloroform was added to dissolve the sample and mixed with 15 mL of CH3COOH. To prepare a saturated KI solution, 99% potassium iodide was dissolved in tertiary distilled water at a ratio of 7:3. 1 mL of saturated KI solution was added into the Erlenmeyer flask, the sample was then homogenized for 1 min and kept in the dark for 10 min. After 10 min, 30 mL of distilled water was added, and the mixture was homogenized again. Then, 1 mL of 1% starch solution was added and the solution was titrated with 0.01 N Na2S2O3 solution until the indicator color was disappeared. A blank sample (distilled water) was conducted with the same procedure.
Sample (5 g) was homogenized with methanol (45 mL) with a homogenizer (AM-7, Nissei), followed by filtration with a Whatman No. 2 filter paper (Advantech). Blank sample was prepared with 5 mL methanol. Reference was prepared with 1 mL of 2,2-diphenyl-1-picrylhydrazyl (DPPH) radical scavenging activity and 4 mL methanol. The testing sample was prepared with 2 mL filtrate, 1 mL of DPPH radical scavenging activity, and 2 mL methanol. After wrapping with an aluminum foil, blank sample, reference, and test samples were kept in a dark room for 20 to 30 min. Then, 250 μL was added to a 96-well plate and measured the absorbance at 517 nm using a microplate reader (DU-650, Beckman). The DPPH radical scavenging activity value was calculated using the following formula:
All experiments were repeated three times. Statistical analysis was conducted by one-way analysis of variance using the generalized linear model of SAS program (SAS Institute, Cary, NC, USA, 1999). Duncan Multiple Range Test was used to evaluate the significant differences among treatments (p<0.05).
Results and Discussion
Phenolic acids, including gallic acid, vanillic acid, caffeic acid, syringic acid, p-coumaric acid, phenylacetic acid, and benzoic acid, were analyzed and identified (Table 2). Phenolic compounds comprise one or more aromatic rings with hydroxyl groups (Balasundram et al., 2006). They exhibit antioxidant, anti-inflammatory, and antiviral effects (Benavente-García et al., 1997). TMP consisted of gallic acid (1.17 μg/L) and p-coumaric acid (18.17 μg/L). PBP included vanillic acid (33.18 μg/L), caffeic acid (10.88 μg/L), syringic acid (17.27 μg/L), phenylacetic acid (17.51 μg/L), and benzoic acid (0.82 μg/L). GBP contained gallic acid (18.17 μg/L) and benzoic acid (0.79 μg/L). ADP had gallic acid (4.46 μg/L), caffeic acid (3.52 μg/L), syringic acid (3.83 μg/L), and phenylacetic acid (5.27 μg/L). It has been proved that the antioxidant capacity of edible insects is mainly provided by phenolic compounds. Aiello et al. (2023) reported that edible insects’ phenolic compounds can exert antioxidant biological activity with potential as bioactive sources. The phenolic chemicals from edible insects could improve food quality and provide antioxidant activity (Torres-Castillo and Olazarán-Santibáñez, 2023). Therefore, phenolic compounds of the four EIPs predicted the potential to be used as natural antioxidants in food.
Fig. 1 displayed that proximate composition of pork patties added with EIPs. Moisture contents were significantly lower in treatments with EIP (p<0.05). Protein contents were higher in groups added with T20, P20, and G20 compared to PC and NC (p<0.05). However, there was no significant difference in the treatment with A20 than PC and NC (p<0.05). As shown in Table 3, the protein contents of TMP (50.65%), PBP (60.35%), GBP (60.38%), and ADP (32.75%) influence the protein contents of patties. Kim et al. (2016) reported that emulsion sausages added with insects have lower moisture content but higher protein content. Carbohydrate content was significantly higher in the group added with A20 than those of PC and NC (p<0.05). This might be due to chitin, a dietary fiber, found in the exoskeleton of insects (Kipkoech, 2023; Montowska et al., 2019). Fat content and ash content were significantly higher in treatments added with EIP than those of PC and NC (p<0.05). Edible insects possess a wealth of UFAs and essential minerals, including copper, iron, magnesium, selenium, and zinc (Lu et al., 2024; Zielińska et al., 2015). These components could influence fat and ash contents in pork patties.
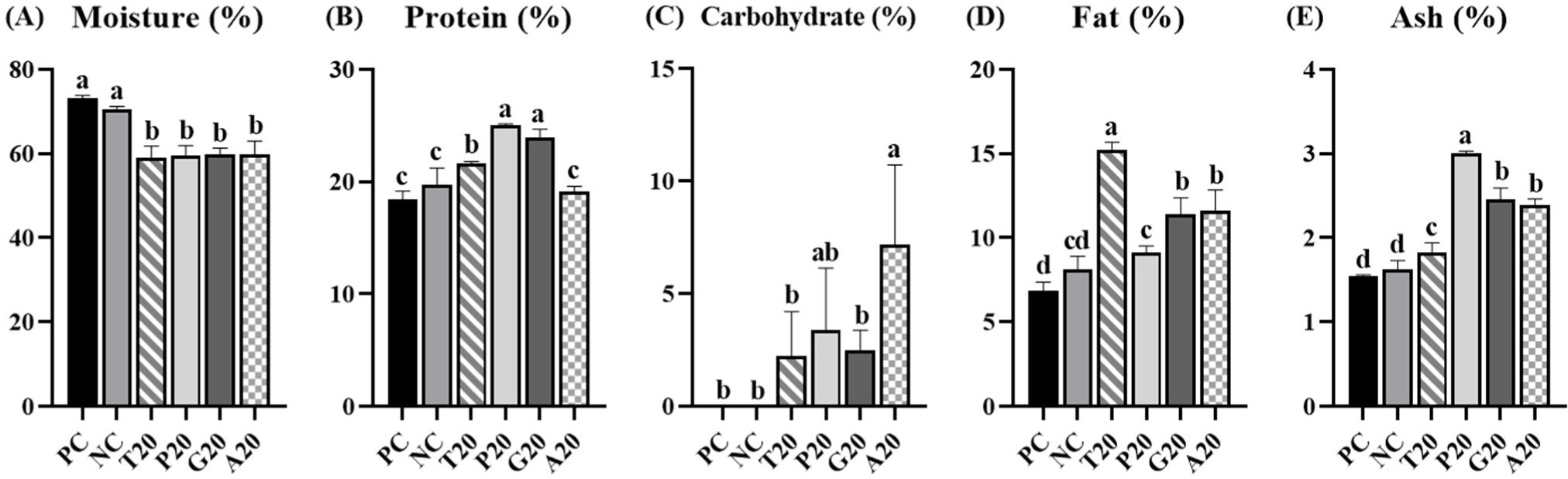
The results of pH, WHC, CL, and patty color with added EIP are presented in Table 4. Additionally, Fig. 2 displays the color (visual appearance) of the patties in photographs after the cooking process. It was found that pH values were significantly higher in treatments added with EIP than those of PC and NC (p<0.05). TMP, PBP, GBP, and ADP had pH values of 6.59, 7.47, 6.84, and 7.19, respectively (Table 3). The pH of EIP might be affected by the pH of the patties. The isoelectric point of meat is 5.2–5.4. A pH higher than the isoelectric point of meat products can increase WHC and lower CL (Honikel, 2008). WHC indicates the ability of meat to retain water. It is an important criterion for evaluating meat quality (Honikel, 2004; Szmańko et al, 2021). Treatments added with EIP showed significantly (p<0.05) higher WHC values than those of PC and NC. When insect protein was added to phosphate-free meat emulsion increased the WHC (Kim et al., 2022). Also, CL was significantly lower in treatments added with EIP than those of PC and NC (all p<0.05). This result is attributed to decreased moisture and increased solid contents of meat emulsion formulation that contains insect powders (Kim et al., 2016). Bessa et al. (2023) have been reported that CL was decreased when Hermetia illucens L. was added as a meat substitute to burger patties.
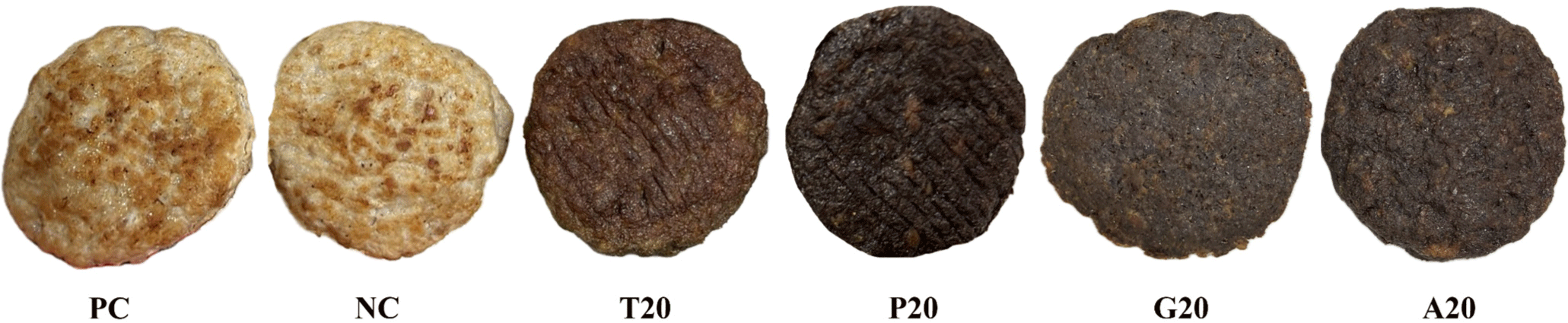
Compared to PC and NC, all treatments with EIP added showed lower CIE L*-value (p<0.05). CIE a*-value was the highest in the group with T20 and it was the lowest in treatment with A20 compared to PC and NC (p<0.05). CIE b*-value was significantly lower in the groups added with P20, G20, and A20, and higher added with T20 (p<0.05). Similarly, Choi et al. (2017) reported that the addition of yellow worms (T. molitor L.) to Frankfurt could reduce CIE L*-value but increase CIE a* and CIE b*-value. Lemke et al. (2023) reported that the addition of 20% insects to sausage products reduced in CIE L*-value compared to 10%. Cruz-López et al. (2022) reported that the CIE L*-value of sausage decreases when insect powder. Edible insects have different melanin contents (Wittkopp and Beldade, 2009). It could contribute color differences in the pork patties.
The TPA values of patties made with various EIPs are shown in Table 4. The treatment with G20 had the highest hardness compared to PC and NC (p<0.05). This might be due to a decrease in water content when EIP was added. Kim et al. (2017) reported that hardness increased when meat was replaced with cricket powder, which was similar to this study. Springiness was significantly higher in the group of PC, NC, and G20 (p<0.05). Ho et al. (2022) reported that an increase in springiness was observed in the sausage partially substituted with cricket powder. Cohesiveness was significantly highest in G20 among the EIP-added treatments (p<0.05) and showed no significant difference from PC and NC. Chewiness and Gumminess were also significantly the highest in the group with G20 added compared to other treatments (p<0.05). Damasceno et al. (2023) found that albumin that can also affect the texture properties of meat is the most highly distributed protein in G.bimaculatus powder. The addition of albumin to meat batter can cause a change in hardness (Pietrasik, 2003). Carballo et al. (1995) reported that the addition of albumin-rich egg white to Bologna sausage increased its hardness and chewiness. Thus, the albumin component of G. bimaculatus could influence the tissue characteristics of pork patties. Our findings indicate that adding EIP as a meat partial substitute can improve the WHC and TPA of patties and reduce CL. Therefore, using EIP could be beneficial in enhancing the quality and texture properties of pork patties.
Table 4 displays the sensory evaluation results of patties added with EIPs. Color, flavor, juiciness, and umami were lower in all treatments added with EIP than in those of PC and NC (p<0.05). The meat juice showed a trend opposite to the WHC results, this might be due to the high-fat content of edible insects with more fat released than those of PC and NC during cooking. Pinter et al. (2021) reported that more fat was released when cooking hamburger patties added with insects. Overall preference was lower for treatments added with EIP than those of PC and NC (p<0.05), which meant that the addition of EIPs to patties decreased acceptability. Megido et al. (2016) reported that beef burger patties added with mealworms had a lower preference than pure beef patties. Also, Cruz-López et al. (2022) stated that pork sausage with locust (Sphenarium purpurascens) powder had low preference. According to the results of this study, adding EIP to patties can lower preference, but it is believed that this can be solved by manufacturing by reducing the amount of EIP or adding auxiliary ingredients.
Total microbial count (TMC) increased with the storage time (p<0.05; Table 5). Yong et al. (2023) reported that the number of microorganisms in Tteokgalbi added with edible insect extract increased with the storage time. All treatments added with EIPs had significantly higher TMC than PC and NC (p<0.05). By adding EIP, not only protein but also other nutrients increased (Fig. 1). It is thought that the number of microorganisms has increased because this nutrient-rich environment serves as a growth medium for various microorganisms (Anas et al., 2019; Elsharawy et al., 2018). Among treatments added with EIP, the treatment added with G20 had the lowest TMC (p<0.05). Malm and Liceaga (2021) found that the chitosan in the cricket had antibacterial effects. Thus, the reason why treatment with G20 had lower TMC value than other EIP-added treatments might be due to high antibacterial activity of crickets.
TMC, total microbial count; TBARS, thiobarbituric acid reactive substance; PV, peroxide value; DPPH, 2,2-diphenyl-1-picrylhydrazyl; PC, positive control; NC, negative control; T20, 20% Tenebrio molitor L. powder; P20, 20% Protaetia brevitarsis seulensis L. powder; G20, 20% Gryllus bimaculatus powder; A20, 20% Allomyrina dichotoma L. powder.
Since lipid oxidation causes a decrease in the quality of meat and meat products, peroxide value (PV) and TBARS were used as indicators of lipid oxidation to confirm this in this study (Love and Pearson, 1971; Turgut et al., 2017). PV measures the primary product of lipid oxidation (hydrogen peroxide) while TBARS measures the end product of lipid oxidation (Gan et al., 2022; Hadidi et al., 2022; Juntachote et al., 2007; Simic and Taylor, 1987). PV showed increasing until the 3rd day and then decreased, and TBARS increased values in all treatments as the storage period increased (p<0.05; Table 5). This is because hydroperoxides decompose into secondary products (Gunstone and Norris, 1983). All treatments added with EIP showed lower PV values but higher TBARS values than those of PC and NC (p<0.05). Han et al. (2023) reported that the TBARS value of hybrid sausage added with cricket flour increased. Also, it has been reported that adding silkworm pupae flours to emulsion sausage increases the TBARS value (Kim et al., 2016). It is believed to be due to the high fat content of EIP.
Table 5 showed DPPH radical scavenging activity of pork patties with EIPs. The treatments added with EIP showed higher antioxidant activities than those of NC (p<0.05). Yong et al. (2023) reported that Tteokgalbi with edible insect extract had high DPPH radical scavenging activity, but it decreased as the storage period increased. It also reported that the antioxidant activities of Dactylopius opuntiae extract were confirmed when applied to beef patties (Aragon-Martinez et al., 2023). When compared with the PC, the treatment with G20 showed a similar DPPH radical scavenging activity value. This is because GBP had a higher gallic acid content than other EIPs (Table 2). This is similar to the report that cricket (G. bimaculatus) has phenolic compounds, showing excellent antioxidant activity (Baigts-Allende et al., 2021; Kurdi et al., 2021). Di Mattia et al. (2019) reported that water-soluble extracts of crickets showed the highest antioxidant capacities and other insect extracts also showed high antioxidant capacities. From these results, we can assume that when EIP is added to patties, the antioxidant capacity from EIP could be beneficial to pork patties.
Conclusion
This study investigated the effect of adding EIP as meat partial substitute on the physicochemical properties and storage stability of pork patties. With the addition of EIP, the moisture content of the patties decreased while the protein, carbohydrate, fat, and ash contents increased. Additionally, pH and WHC increased, and CL decreased. TPA showed that hardness, chewiness, and gumminess were higher compared to PC and NC, but overall preference decreased. As a result of storage stability with the addition of EIP, TMC, and TBARS were higher compared to PC and NC, while PV was low values. According to the addition of EIP, DPPH radical scavenging activity was higher than NC, and among patties with EIP added, G20 was similar to or higher than PC. Therefore, the addition of EIP as a meat partial substitute showed a positive effect on the physicochemical properties and antioxidant activities of pork patties. Among the 4 species of EIP, G20 was the most promising insect powder to improve the quality of patties and enhance their antioxidant activities. These findings indicated that EIP can serve as meat partial substitute. However, in order to improve storage stability and preference, further research is needed to apply it to patties by reducing the amount of EIP or adding auxiliary ingredients.