Introduction
Yogurt, which is consumed worldwide as fermented dairy food, contains protein, omega-3 fatty acids, vitamins, and minerals having nutritive functions (Gao et al., 2018). The primary health effects, such as the antioxidant activity of yogurt, are derived from peptides and amino acids produced by lactic acid bacteria during fermentation (Ye et al., 2013). Along with the nutritional values, the proper physicochemical quality in yogurt, such as adequate texture and non-serum separation, plays an essential role in consumer acceptability (Cardines et al., 2018). Generally, the primary defects of yogurt are low viscosity and syneresis (Vital et al., 2015). The addition of stabilizers such as hydrocolloids and the increase of total solids content using milk ingredients have been shown to prevent the defects of yogurt and improve its texture (Nguyen et al., 2017). Recently, many studies suggested that the fortification of yogurt using natural resources could improve not only the quality properties but also the functionality of yogurt with minimal adverse effects (Gahruie et al., 2015; Liu and Lv., 2019). Particularly, polyphenol-rich ingredients such as plants and fruits have received much attention for fortification. The addition of grape and green, white, and black tea into yogurt resulted in improvements in the antioxidant activities of yogurt (Karaaslan et al., 2011; Muniandy et al., 2015). Adding apple polyphenol to yogurt promoted the growth of starter and was beneficial for pre-fermentation (Sun-Waterhouse et al., 2012). Furthermore, polyphenols in green tea and green coffee enhanced the strength and stability of yogurt structure (Donmez et al., 2017).
Polyphenols are secondary metabolites of plants and have strong antioxidant capacities. Although polyphenols are mainly synthesized by plants to defend against oxidative stress, they can contribute to the pharmaceutical and dietary properties of plant-derived foods by functioning as antioxidants (Csepregi et al., 2016). These compounds exhibit excellent antioxidant activity because of their hydroxyl substituents and aromatic structures, which can scavenge free radicals (Villano et al., 2007). Furthermore, milk proteins such as casein possess a strong affinity for the hydroxyl group of phenolic compounds and form protein–polyphenol complexes (Yuksel et al., 2010). The interaction between proteins and polyphenols is maximized at the isoelectric point of proteins. These specific functional properties of polyphenols may be useful for improving the texture and syneresis of yogurt (Donmez et al., 2017).
Lotus (Nelumbo nucifera Gaertn.), which is rich in polyphenols, is an aquatic perennial plant primarily cultivated in eastern Asia (Huang et al., 2010). This plant is composed of leaves, rhizomes, flowers, and embryos, most of which have been used as foodstuffs or medicine (Jung et al., 2008). The leaves of lotus are extensively used as a traditional medicine in China because of their diverse health-promoting effects including antioxidant, anti-viral, anti-obesity, and anti-hyperlipidemic effects and are used to treat fever, sweating, and strangury (Lin et al., 2009; Sridhar and Bhat, 2007). The bioactivities of lotus leaf are conferred by phenolic compounds such as flavonoids and phenolic acids, including hyperin, isoquercetin, kaempferol, myricetin, and catechin (Limwachiranon et al., 2018). Moreover, alkaloids (nuciferine, nerine, N-nornuciferine, roemerine, and pronuciferine) in lotus have pharmacological effects (Chang et al., 2016). Lotus leaves are mainly consumed as a beverage or in tea bags (Huang et al., 2010) but are not widely utilized in the food industry. The addition of lotus leaf may improve the quality and functional properties of the yogurt. In this study, we investigated the effects of lotus leaf (LL) powder on yogurt quality and antioxidant activity during 14 d of refrigerated storage.
Materials and Methods
LL powder was purchased from a local market (Kyung-gi, Korea). Milk and skim milk powder were obtained from the Seoul Diary Cooperative (Seoul, Korea), and pectin (Jong ro, Korea) and sugar were purchased from a local market. The starter consisted of Streptococcus thermophilus, Lactobacillus delbrueckii ssp. bulgaricus, Lactobacillus acidophilus, and Bifidobacterium animalis ssp. lactis (Lyofast YAB 450 AB, Sacco S.R.L., Italy).
The yogurts were produced as shown in Fig. 1. Milk, skim milk powder, pectin, sugar, and LL powder (0%, 0.2%, 0.5%, and 1%) were homogenized using a homogenizer. The mixture was pasteurized at 85°C for 30 min. After cooling to 42°C, the starter (0.002%) was inoculated and fermented at 42°C until the pH reached 4.5±0.2. The samples were stabilized at 4°C for 12 h after completing the fermentation and stored at 4°C until analyses at 0, 7, and 14 d.
The pH of the yogurt during storage was measured using a pH meter (pH 900, Precisa Co., Dietikon, Switzerland). Titratable acidity (TA) was determined by titrating 5 g of the samples diluted by 10-fold with 0.1N NaOH until the pH reached at 8.3. MRS agar (Oxoid, Ltd., Hampshire, UK) was used to quantify total lactic acid bacteria (LAB). The plate was incubated at 37°C for 72 h.
Color was determined by determining the values of CIE L* (0, black; 100, white), CIE a* (−, greenness; +, redness), and CIE b* (−, blueness; +, yellowness) by using a colorimeter (NR-300, Nippon Denshoku, Tokyo, Japan). Before measuring the samples, the instrument was calibrated using a standard white plate.
Viscosity was measured using a viscometer (Model LVDV-E, Brookfield Engineering Laboratories, Inc., Middleboro, MA, USA) with spindle No. 63 at 30 rpm. The yogurts stored at 4°C were stirred for 5 min and measured until 7 min at an interval of 1 min. The results were presented as an average. The water-holding capacity (WHC) was determined as described previously with slight modifications (Singh and Muthukumarappan, 2008). Approximately 30 g of yogurt sample (Y) was centrifuged at 669×g for 20 min at 20°C. The supernatant (S) was removed and weighed. The WHC was calculated as follows:
Preference testing was conducted with the yogurts after fermentation and storage for 12 h at 4°C. Samples were evaluated by 32 untrained panelists (14 males and 18 females; age range, 21–47 years) who are members of Konkuk University (Seoul, Korea). Each yogurt was provided in a paper cup with a random three-digit code. Assessments included appearance, color, flavor, taste, texture, and overall acceptance and used a 9-point hedonic scale (1, dislike extremely; 9, like extremely). Before evaluating each sample, the panelists rinsed their mouth with water.
Yogurt samples were diluted (1:1 w/v) with distilled water and incubated with shaking at 300 rpm for 2 h. The mixture was centrifuged at 3,900×g for 20 min, and the supernatant was filtered through Whatman paper No. 4 (Maidstone, UK). The filtrate was used to analyze total phenolic content (TPC) and antioxidant activity. The TPC was evaluated as described previously using the Folin-Ciocalteu method with some modifications (Wei, 2011). Briefly, 20 μL of the sample were mixed with 20 μL of 1N Folin and Ciocalteu’s phenol reagent, followed by a 3 min reaction. Next, 60 μL of 1N Na2CO3 was added, and the sample was incubated in the dark for 90 min at room temperature. After incubation, 100 μL of distilled water was added, and absorbance was measured at 725 nm with a spectrophotometer (Multiskan GO, Thermo Fisher Scientific, Waltham, MA, USA). The results were expressed as mg of gallic acid equivalent (GAE) per g of sample (μg GAE/g of sample).
DPPH (2,2-diphenyl-1-picrylhydrazyl) radical scavenging activity was evaluated as described by Blois (1958). First, 40 μL of the sample were mixed with 160 μL of 0.1 mM DPPH (Sigma, St. Louis, MO, USA) and reacted in the dark for 30 min at room temperature. The reactant was measured at 517 nm with a spectrophotometer (Multiskan GO).
Reducing power was assessed according to the method of Oyaizu (1986) with slight modifications. Briefly, 250 μL of yogurt samples, 250 μL of 0.2 M phosphate buffer (pH 6.6), and 250 μL of 1% K3Fe(CN)6 were mixed and reacted in a water bath at 50°C for 20 min. Next, 250 μL of 10% trichloroacetic acid was added to complete the reaction. After the mixture was centrifuged at 865×g for 10 min, 500 μL of clear supernatant was mixed with 500 μL of distilled water, and 100 μL of 0.1% FeCl3 and incubated for 10 min. The results were measured at a wavelength of 700 nm with a spectrophotometer (Optizen 2120UV, Mecasys, Daejeon, Korea).
The experiments were performed three times, and all samples were analyzed in triplicate. The obtained data were presented as the mean±standard (SD) deviation. Statistical analysis was performed using SPSS statistics 18.0 software (SPSS, Inc., Chicago, IL, USA) by one-way analysis of variance. Tukey’s test was used to compare the differences among all groups (p<0.05).
Results and Discussion
The changes in pH, TA, and total LAB count of yogurts during storage are shown in Table 1. The pH of 0 d yogurts was 4.39–4.49. There were significant differences between control and LL yogurts (p<0.05). The LL yogurts had lower pH values than those of the control, which is similar to the results of a previous study evaluating the addition of lotus leaf extracts to yogurt (Joung et al., 2016). Acids such as tartaric acid, citric acid, malic acid, and succinic acid in LL (Choe et al., 2011) may influence the low pH of yogurts. After 14 d of storage, the pH of yogurts gradually decreased (p<0.05), showing values of 4.13–4.24. This is regarded as a suitable pH range for yogurt, as no pH values were below 4.0, which generally considered a harsh environment for the survival of probiotic organisms (Barkallah et al., 2017).
The TA of yogurts ranged from 0.94 to 1.19 during storage. The TA of LL yogurts was significantly higher than that of the control (p<0.05). During the storage period, the TA steadily increased because of the accumulation of lactic acid and formic acid produced by LAB (Sengul et al., 2012). TA values typically range from 0.72% to 1.20% (Davis, 1970). The TA values of all yogurt groups were within this range.
LAB counts in all yogurt groups were over 7.0 Log CFU/g, which is the minimum requirement according to the Codex Alimentarius. The number of LAB tended to increase with increasing LL concentrations, but no significant differences were observed (p>0.05). The abundant dietary fiber in LL (Choe et al., 2011) may have slightly increased in the number of LAB. The LAB counts tended to increase until 7 d of storage and then decreased in all yogurt groups but not significantly (p>0.05). This variation in the number of LAB could be because of the change of milk composition and pH level during the refrigerated storage (Bakr, 2013). Nevertheless, LL did not adversely affect the growth of LAB in yogurt.
Because the visible appearance of food has a substantial influence on acceptance by consumers, color is an important factor affecting the quality of yogurts (Coggins et al., 2010). Table 2 shows the index values of different color parameters, L*, a*, and b*. The L* values significantly decreased in a concentration-dependent manner (p<0.05). Additionally, the a* values of LL yogurts were lower, and b* values were higher than those in the control (p<0.05). Pigments in LL, such as chlorophyll and flavonoid, cause the yogurt to become dark, green, and yellow (Choe et al., 2010). Suh color changes were noted in yogurt containing green olive powder (Cho et al., 2017). The addition of LL powder did not affect the L* values of yogurts during 14 d of storage (p>0.05). Significant variations in the a* and b* values of LL yogurts were observed during storage (p<0.05). This may be because of the degradation and oxidation of LL pigments (Bakirci et al., 2017).
Viscosity improved when LL powder was added to the yogurts (p<0.05). At least 4-fold higher viscosity was observed in LL yogurts than in the control during storage (Fig. 2). Polyphenols can bind to proteins and form protein–polyphenol complexes (Vital et al., 2015). The abundant phenolic compounds in LL (Limwachiranon et al., 2018) interacted with milk proteins such as casein in the yogurt matrix, resulting in higher viscosity than that in the control.
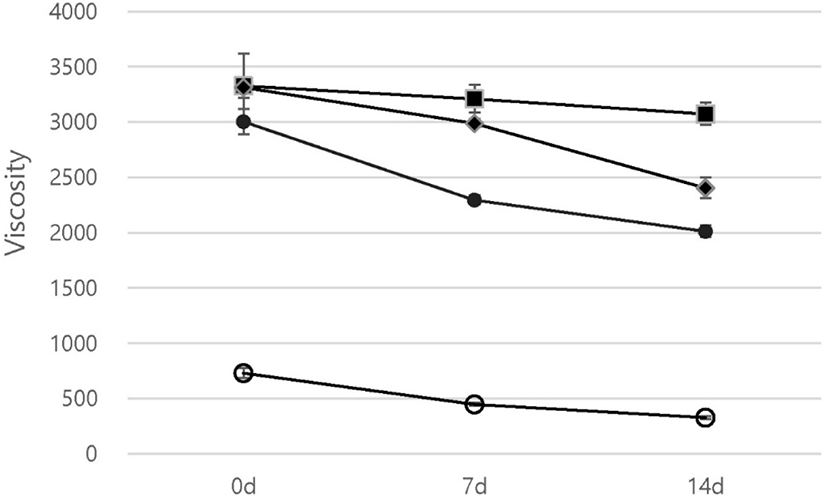
Moreover, the viscosity appeared to decline at high concentrations of LL powder, showing concentration-dependent effects. The 0.2% LL yogurt showed the highest viscosity at 0 d of storage (3,328 cP). Similarly, the viscosity of cherry pulp yogurts was reported to gradually decrease with higher concentrations of cherry pulp (Sengul et al., 2012). The viscosities in all yogurt groups, except for the 0.2% LL group, significantly decreased during storage (p<0.05). Yogurt viscosity decreases during storage because of whey separation (Al Mijan et al., 2014). This indicates that the addition of 0.2% LL to the yogurt can prevent whey separation and increase stability during storage.
WHC is related to the ability of a protein to hold water in the yogurt gel structure (Kinsella and Moor, 1984) and is a critical property in food technology (Kneifel and Seiler, 1993). As shown in Fig. 3, the WHC of LL yogurts was higher than that of the control during storage (p<0.05). Barkallah et al. (2017) reported that dietary fibers could retain water, increasing the WHC of yogurt. The abundant dietary fiber in LL (Choe et al., 2011) may explain the high WHC of the yogurt. Similar to the results for viscosity, the 0.2% LL yogurts showed the highest WHC value during storage (p<0.05). Yogurt is a gel of casein micelle with entrapped serum, and both viscosity and WHC are related to the gel structure of yogurt (Achanta et al., 2006). Donmez et al. (2017) found that when excess polyphenols bind with protein, the number of cages that play a role in limiting serum release from the yogurt gel network increased; however, the volume of the individual cage decreased and caused a reduction in serum trap time. In this study, the amounts of polyphenols present in LL over 0.2% were in excess for the interaction with the protein in the yogurt gel. Therefore, the serum could not be adequately trapped, possibly causing reduced WHC and viscosity. Thus, 0.2% LL contains a suitable concentration of polyphenols to form a stable and robust gel in yogurt.
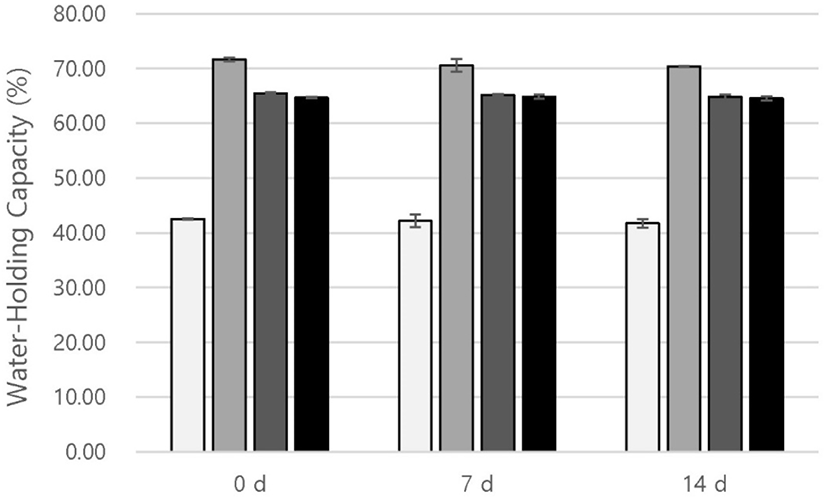
Table 3 shows the results of the preference test. LL significantly influenced the results of the preference test of yogurts (p<0.05). Generally, the control showed a higher value than did the LL yogurt groups for most preference test parameters. Among the treatment groups, preference was in the order of 0.5%<1%<0.2% for LL yogurts, but the differences were not significant (p>0.05). The green color of LL negatively affected the appearance and color of yogurts. Sung et al. (2005) reported similar results in drinkable chlorella yogurt, which had a greenish color. The preferences for the flavor and sweetness of LL yogurts were relatively low. For texture parameters, 0.2% and 1% LL yogurts showed higher values than that of the control, but the difference was not significant (p>0.05). LL concentrations of 0.2% and 1% are suitable for fortifying yogurt based on the result of the preference test, although parameters such as color, flavor, and sweetness must be further optimized.
The results of TPC and antioxidant activity analyses of yogurts are shown in Table 4. TPC in LL powder was analyzed as a preliminary test and was found to be 24.0±0.21 mg GAE/g. It was much higher than that of other plants previously used in studies of fortified yogurts, such as blueberry flower (280±1.20 mg GAE/100 g), Pleurotus ostreatus aqueous extract (16.55±0.08 mg GAE/g), and olive leaf (1,370 mg GAE/100 mL) (Liu and Lv, 2018; Peker et al., 2016; Vital et al., 2015). The TPC of 0 d yogurts ranged from 17.94 to 47.94 μg GAE/g. The LL powder significantly influenced the TPC in yogurt in a concentration-dependent manner (p<0.05). Particularly, the 1% LL yogurts showed 2.6-fold higher TPC values than that in the control. The abundant phenolic compounds such as kaempferol, quercetin, and isoquercetin in LL (Choe et al., 2010) influenced the high TPC of yogurts. The Folin-Ciocalteu reactivity of plain yogurts is derived from milk components such as free amino acids, peptides, proteins, and low-molecular-weight antioxidants, as well as polyphenols (Helal and Tagliazucchi, 2018). The TPC of the control and 0.2% LL yogurts significantly increased until 7 d of storage (p<0.05) and then increased slightly. Further, the 0.5% and 1% LL yogurts showed increased TPC during storage (p<0.05). The highest content of TPC was 61.94±1.68 μg GAE/g in 1% LL yogurts at 14 d in this study. The gradual increase in TPC in all yogurts during storage occurred because of the proteolysis of milk protein, which released amino acids with phenolic side chains. Additionally, the metabolism of microbes that may have produced new phenolic acids by utilizing phenolic compounds such as ferulic and p-coumaric acid could contribute to the increased TPC (Muniandy et al., 2016).
To investigate the antioxidant activity of the yogurts, DPPH radical scavenging activity and reducing power were examined, which are commonly conducted for foods (Ferreira et al., 2007; Villano et al., 2007). The gradual increase in antioxidant activity in LL-added yogurts was investigated by measuring both DPPH radical scavenging activity and reducing power (p<0.05). The highest value for each antioxidant activity assay was observed at 7 d of storage in 1% LL yogurt (58.37%, 0.569, respectively). Plain yogurt shows antioxidant activity because of the bioactive peptides generated from the proteolysis activity of lactobacilli (Rutella et al., 2016). The enhanced antioxidant activity of LL yogurts may result from the phenolic compounds, which play an essential role as antioxidants (Csepregi et al., 2016). Moreover, compounds with antioxidant activities such as aporphine, alkaloids, and vitamins of LL (Liu et al., 2014; Lee et al., 2008) may have increased the antioxidant activity of LL yogurts. The antioxidant activity of yogurts tended to increase during 7 d of storage and gradually declined until 14 d of storage. This reduction of antioxidant activity may be related to the degradation of phenolic compounds and increased interactions between milk proteins and polyphenols (Amirdivani and Baba, 2011).
Conclusion
All LL yogurts showed reasonable ranges for pH, TA, and viable lactic acid counts during storage. Improved viscosity and WHC were observed when LL powder was added to yogurt. Notably, the 0.2% LL yogurt remained stable during storage. In the preference test, the texture parameters of LL yogurts (0.2%, 1%) showed higher values than those of the control. The abundant phenolic compounds of LL increased the antioxidant activity of yogurt. In conclusion, this study confirmed that LL enhanced the quality and functional benefits of yogurt, such as antioxidant activity. Addition of 0.2% LL is recommended to improve the gel formation and stability of yogurt.