Introduction
The production of poultry meat, including chicken, is predicted to reach 130.7 million tons in 2023, making the poultry meat industry the largest industry in the meat production sector (Skarp et al., 2016). The huge demand for poultry meat is attributed to its nutritional benefits, including high protein content and low cholesterol and saturated fat contents (Lopez-Andres et al., 2018). In chicken meat, the content of n-3 polyunsaturated fatty acids is also higher than that in beef, lamb, and pork, thus providing more health benefits.
However, the short shelf life of chicken and the risk of pathogen contamination are the main problems faced by the chicken meat industry. The short shelf life of chicken is attributed to the rapid oxidation of unsaturated fatty acids, whose content is relatively high in chicken meat (Marcinkowska-Lesiak et al., 2016). Pathogen contamination can occur in slaughter houses. In some countries, the volume of chicken processed in a single slaughterhouse is as high as 12,000 birds/h; therefore, maintenance of sanitary conditions is difficult, and the spread of microorganisms between carcasses is increased (Lin et al., 2004).
Listeria monocytogenes contamination in slaughterhouses has been frequently reported (Schafer et al., 2017). Moreover, ready-to-eat chicken is often associated with L. monocytogenes infection outbreaks (Elmali et al., 2015). Indeed, the pathogen can survive over wide temperature (−0.4°C to 45°C) and pH (4.4–9.4) ranges and is resistant to many disinfectants (Schafer et al., 2017). Foodborne illnesses resulting from L. monocytogenes infection (listeriosis) are a serious public health concern, with 1,600 cases and 260 deaths reported in the United States of America (Garner and Kathariou, 2016) and 2,206 cases (20%–30% mortality) reported in the European Union (Luth et al., 2018) in recent years.
Many attempts have been made to reduce L. monocytogenes contamination in the chicken meat industry. The use of high hydrostatic pressure (Kruk et al., 2011) and ultraviolet-C irradiation (Chun et al., 2010) effectively reduce the population of L. monocytogenes in artificially inoculated chicken breasts. Although chlorine is well known as effective antimicrobial compound, scientist tried to find natural antimicrobial compounds in consumer’s point of view (Brackett, 1987). Additionally, treatments with slightly acidic electrolyzed water (Mansur et al., 2015) and lauric arginate (Sharma et al., 2013) have also been effective in reducing L. monocytogenes contamination in different food products. Extracted oil from members of the genus Allium, including garlic (Allium sativum) and scallions (Allium ascalonicum L.), has been reported to exhibit antimicrobial and antioxidant activities (Yin et al., 2003), owing to the presence of organosulfur compounds, such as diallyl sulfide (DAS), diallyl disulfide (DADS), allicin, and ajoene (Yin et al., 2003) in them. Similarly, gold kiwi (Actinidia chinensis) possesses antioxidant and antimicrobial properties because of the presence of vitamin C and flavonoids (Du et al., 2009) in it. However, reports on the use of those scallions, garlic, and kiwi fruits extracts for reducing L. monocytogenes contamination in chicken meat have been scarce.
Therefore, in the current study, we evaluated the antimicrobial and antioxidant activities of lyophilized scallion, garlic, and gold kiwi extracts (SLE, GLE, and GKE, respectively) as natural antimicrobial or marinating compounds for chicken breast meat inoculated with L. monocytogenes.
Materials and Methods
Scallions, garlic, and gold kiwis were obtained from a local supermarket in Korea. All samples were washed in running tap water before extraction. Extracts were prepared by squeezing in a juice extractor (NJE-3834, NUC electronics, Daegu, Korea), lyophilizing, and storing at −20°C until analysis. The garlic and gold kiwi were peeled before squeezing. The yields of plant extracts in the powder form after lyophilization were 5.77% for SLE, 22.01% for GLE, and 7.15% for GKE.
Escherichia coli (KCCM 11234), L. monocytogenes (KCCM 40307), Staphylococcus aureus (KCCM 12256), and Salmonella Enteritidis (CCARM 8260) cultures, stored at −70°C, were streaked on tryptic soy agar (MB Cell, Seoul, Korea) plates and incubated at 37°C for 24 h. The inocula of the test organisms were prepared by transferring a single colony from culture plates into 10 mL sterile tryptic soy broth (TSB; MB Cell) and culturing at 37°C for 24 h. These cultures were further used for testing the antimicrobial activities and for inoculation of chicken breasts.
The MIC and MBC were determined using microdilution of TSB in 96-well plates; 130 μL TSB, 20 μL microorganism suspension, and 50 μL extract were added to each well. The plates were incubated at 37°C for 24 h, and the absorbance was detected at 600 nm using a microplate reader (SpectraMax M2e; Molecular Devices, Sunnyvale, CA, USA). The MIC was determined as the lowest sample concentration showing no visible microbial growth in the broth. The MBC was recorded as the lowest concentration that killed 99.9% of the inoculated bacterial cells.
The ABTS scavenging assay was performed according to the method of Re et al. (1999). The activities of the lyophilized plant extracts measured absorbance at 735 nm at 30°C. The percentage of inhibition was shown as IC50 values, which was concentration need for 50% inhibition of ABTS radical scavenging activity.
The FRAP assay was conducted as described by Benzie and Strain (1996). The absorbance of the sample was measured at 590 nm using a microplate reader. The FRAP activity was determined as percentage of the ratio between absorbance of sample against blank and absorbance of sample. The results are expressed as the effective concentration (EC50, in mg/mL) to reduce the FRAP activity by 50%, calculated from the graph of FRAP activity plotted against the concentration of the sample.
Forty skinless chicken breasts (approximately 170–200 g) were obtained immediately after slaughter from a local slaughterhouse and were promptly transported to the laboratory with remaining at 4°C. After chicken breast was decontaminated by wiping with 70% ethanol using a hand towel, the meat was then inoculated by dipping in a 700 mL solution containing 70 mL TSB with 6–7 Log CFU/mL L. monocytogenes and 630 mL sterile saline solution for 15 min at 23°C according to the method of Alonso-Hernando et al. (2015). Then, the chicken breasts were dried for 20 min at 23°C in a clean room.
Total forty inoculated chicken breast were separated into four treatment groups (n=10). Treatment of plant extract on chicken breast performed by the method of Alonso-Hernando et al. (2015) with slight modification. The lyophilized powder of SLE, GKE, and GLE dissolved in distilled water as original concentration of extracts and made final concentration of 1% SLE, GKE, and GLE as a dipping solution. The inoculated chicken breasts in each treatment group were dipped into a 700 mL solution containing 1% SLE (LSLE), 1% GKE (LGKE), 1% GLE (LGLE), respectively, or in distilled water (LCON, used as a control) for 15 min at 23°C in a clean room. The dipping solution was changed with fresh one after dipping every five breast meats. Thereafter, the chicken breasts were removed from the solution and allowed to dry for 20 min at 23°C in a clean room. Subsequently, the samples were placed on styrofoam trays, wrapped with low-density polyethylene, and stored for 9 days at 4°C. Analysis were done on days 1, 3, 5, 7, and 9 of storage.
Ten grams of chicken breast meat was homogenized with 90 mL distilled water for 30 s using a homogenizer (Polytron R PT-2500 E; Kinematica, Switzerland), and the pH of the homogenates was directly measured using a pH meter (Orion 230 A; Thermo Fisher Scientific, Inc., Waltham, MA, USA).
The samples were placed in a polyethylene bag and boiled in a constant temperature water bath until the internal temperature of meat reached 75±2°C. The samples were then cut into 1×3×2 cm pieces and used for measurement of shear force using a texture analyzer (TA 1; Lloyd Instruments, Berwyn, PA, USA). The texture analyzer was set to a 50 kg load cell, 50 mm/min trigger speed, 50 mm/min test speed, and 10 gf trigger force.
The color of the meat was measured using a colorimeter with an illuminant-D65, standard observer-2°, and 8 mm aperture size (Colorimeter CR-400; Minolta Co., Osaka, Japan). The Commission Internationale de l’Eclairage (CIE) color values (lightness CIE L*, redness CIE a*, and yellowness CIE b*) were repeatedly measured on the surface of the meat.
Chicken meat samples (10 g) were minced and placed aseptically into individual stomacher bags. Ninety milliliters of sterile saline solution were added, and the samples were homogenized for 40 s using stomacher (Bag Mixer 400 P, Interscience, St Nom, France). For each sample, serial dilutions were performed with sterile saline solution. The appropriate dilutions of sample homogenates were spread on the surface of selective agar plates. Plate count agar (Difco Laboratories, Detroit, MI, USA) was used for TVC and the plate incubated for 48 h at 37°C. The L. monocytogenes count was determined using selective PALCAM agar media (MB Cell, Seoul, Korea) after incubation of the plate at 30°C for 48 h.
The VBN content was analyzed by the micro diffusion method, as described by Kim et al. (2018). Ten grams of minced chicken breast meat was homogenized for 30 min in 50 mL distilled water on a magnetic stirrer, and the homogenate was then filtered through filter paper (Whatman No. 1). The Conway unit containing the filtrate was incubated with 0.01 N H2SO4 for 1 h at 25°C. After incubation, 10 μL Brunswik indicator was added to the inner chamber of the Conway unit and titrated against 0.01 N NaOH. The VBN value was calculated using the following equation:
where a is the volume of 0.01 N NaOH added to the sample (mL), b is the volume of 0.01 N NaOH added to the blank (mL), F is the standard factor for 0.01 N NaOH, W is the sample weight (g), and d is the dilution factor.The TBARS value was determined according to the method of Kim et al. (2018). Briefly, the meat samples (5 g) were homogenized with 15 mL distilled water, and a 1 mL aliquot was used for the reaction. One milliliter of sample aliquot was added to 2 mL of 20 mM 2-trichloroacetic acid (dissolved in 15% thiobarbituric acid) and boiled at 90°C in a water bath for 15 min. After cooling in cold water, the sample was centrifuged at 2,000×g for 10 min. The absorbance of the supernatant was measured at 531 nm. The TBARS values were quantified as mg of malondialdehyde (MDA) per kg of meat sample.
Data were analyzed by the SAS program (ver. 9.2; SAS Institute, Cary, NC, USA) using one-way analysis of variance with a general linear model. The significance of differences in the mean values for different samples was determined by Tukey’s test. Differences with p values of less than 0.05 were considered significant.
Results and Discussion
All plant extracts showed inhibitory and bactericidal activities against L. monocytogenes (Fig. 1). The SLE and GLE had the lowest MIC values of 25 mg/mL each. The SLE had the most effective inhibitory activity against L. monocytogenes, as previously suggested by Yin et al. (2003), who showed that scallion oil had high antimicrobial activity. The high antimicrobial activities of members of the genus Allium, such as scallions and garlic, are attributed to the high content of allicin-derived organo-sulfur compounds, including DAS, DADS, and ajoene (Yin et al., 2003).
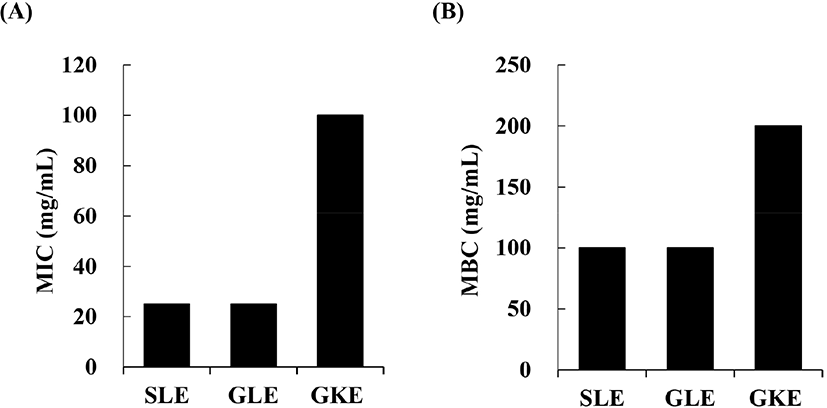
The antioxidant activities of the lyophilized plant extracts were evaluated using ABTS radical scavenging assays and FRAP assays, showed similar trend of antioxidant activity among treatments. GKE had the lowest IC50 value (5.06 mg/mL) in the ABTS radical scavenging assays and the lowest EC50 value (0.67 mg/mL) as determined using the FRAP assay (Fig. 2). The EC50 values of GLE determined using the FRAP assay were significantly higher than that of SLE and GKE. The high antioxidant activity of GKE was reported to be associated with the high content of pyrogallol as the main phenolic compound and vitamin C (Du et al., 2009). It was reported that the antioxidant activity of SLE and GLE was due to its organosulfur compounds such as DAS and DADS (Yin et al., 2003).
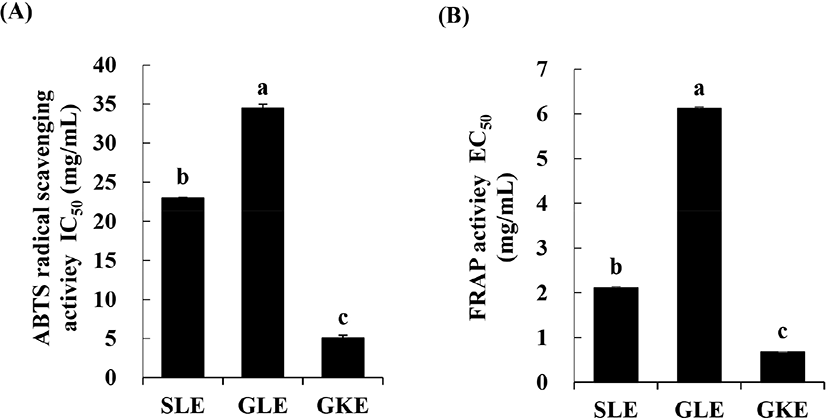
On day 1 of storage, the pH value in the LGKE was 5.99, which was significantly lower than that in the other treatment groups (p<0.05), and the pH values in the LCON, LSLE, and LGLE were not different (Table 1). However, the pH value in the LCON was significantly higher than that in the treatment groups on days 3 and 5. Specifically, the pH in the LCON was significantly increased on day 3 (pH 6.30) of storage and was significantly higher than that on day 1 (pH 6.11) (p<0.05). The pH value in the LSLE was maintained until day 5 of storage. The normal pH value of fresh chicken meat is around 6.09–6.10 (Sujiwo et al., 2018). However, the meat begins to spoil if the pH value reaches 6.20, according to the Food Code of the Ministry of Food and Drug Safety (2019) of Korea. In this study, the LCON sample was found to be decayed after 3 days of storage, whereas in the treatment groups, the decay started only after 5 days of storage. The increase in the pH was related to the growth of spoilage microorganisms. As reported by Knox et al. (2008), spoilage microorganisms, such as Shewanella putrefaciens and Brochothrix thermosphacta, grow at pH values higher than 5.8. The increase in pH may also result from protein degradation (Sujiwo et al., 2018), which occurs during storage via the action of either microorganisms or enzymes (Kruk et al., 2011).
The shear force values on day 1 of storage did not differ among the treatments and were around 2.44–2.63 kgf (Table 1), similar to the values reported by Shim et al. (2018). The shear force value of the chicken breast gradually decreased during storage, indicating that the meat had become tender. On days 3 and 5, the shear force values of LGKE were significantly lower than those of LCON. Actinidin, which is a cysteine protease present in kiwifruit (Christensen et al., 2009), may be responsible for the lower shear force value in LGKE. Moreover, the meat tenderness can be affected by the level of myofibrillar protein degradation in the meat (Marcinkowska-Lesiak et al., 2016).
No significant differences were observed in the CIE L*, CIE a*, and CIE b* color values between the control and treatment groups on day 1 and until day 3 of storage (Table 1). However, on day 9, the CIE a* value in the LSLE was significantly lower than that in the LCON. The CIE a* value in the LSLE could be maintained until day 9 of storage with no significant differences from the control. However, the CIE a* value in the other treatment groups and control group increased gradually. On days 5, 7, and 9 of storage, the CIE b* value was lower in the LCON than in the LSLE and LGLE (p<0.05). The CIE b* value of all chicken breast samples was gradually increased during storage. Similarly, in our previous study (Sujiwo et al., 2018), the CIE b* value of chicken breast was increased from 3.66 (day 1) to 5.89 (day 12). The CIE L* values obtained in the current study were similar to those obtained by Sujiwo et al. (2018), who showed that the CIE L* value ranged from 58.30 to 59.06. The color deterioration of meat occurs because of the oxidation of myoglobin, which is converted to metmyoglobin, imparting brown color to the meat (Young and Lyon, 1997). The discoloration of meat is also related to the growth of microorganisms during storage, which reduces the oxygenation of myoglobin and results in discoloration (Zhang et al., 2011).
The initial TVC of chicken breast was not significantly different between the control and treatments (Fig. 3A). However, on days 3 and 5, the TVC in the LSLE and LGKE were significantly lower than that in the LCON. On days 7 and 9, the TVCs in all treatment groups were significantly lower than that in the control group. The initial L. monocytogenes counts in the inoculated chicken breast were not different among the treatment and control groups and ranged from 4.95 to 5.01 Log CFU/g (Fig. 3B). From day 3 to day 9, the L. monocytogenes level in the LSLE and LGKE was significantly lower than that in the LCON. On day 3, the L. monocytogenes counts in the LSLE and LGKE were 5.60 and 5.51 Log CFU/g, respectively, whereas that in the LCON was 5.74 Log CFU/g. On day 7, the L. monocytogenes counts in the LSLE and LGKE were 6.07 and 6.10 Log CFU/g, which were significantly lower than the levels in the LCON (6.41 Log CFU/g). During storage, the L. monocytogenes count was gradually increased in both the control and treatment groups. This could be explained by the fact that the growth temperature of L. monocytogenes ranges from −0.4°C to 45°C (Schafer et al., 2017). The temperature for the growth limit of L. monocytogenes is 0°C–0.3°C (Cole et al., 1990).
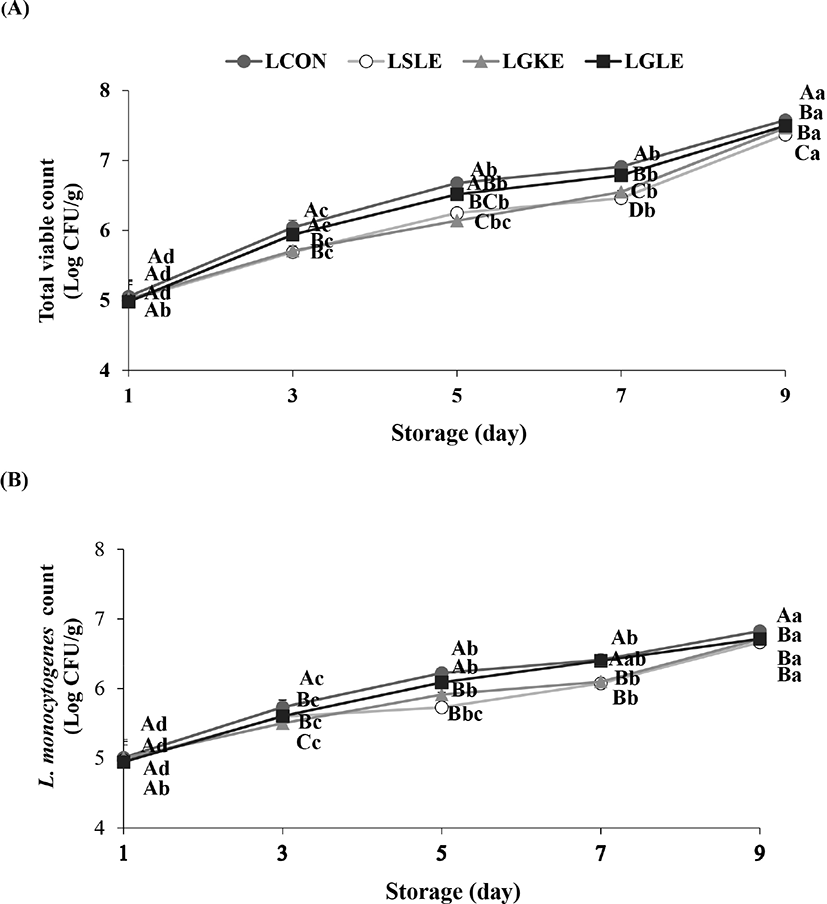
The effectiveness of SLE against microorganisms in the chicken breast as well as against the population of L. monocytogenes is attributed to its content of active antimicrobial allicin-derived organo-sulfur compounds, including DAS, DADS, and ajoene (Yin et al., 2003). In particular, allicin inhibits certain thiol-containing enzymes in these microorganisms (Ankri and Mirelman, 1999).
Yin et al. (2003) reported that the growth of L. monocytogenes could inhibited by scallion oil extract in a paper disc diffusion assay. Additionally, kiwi fruit has been reported to inhibit the growth of several gram-positive bacteria (Basile et al., 1997), similar to our results demonstrating that GKE inhibited L. monocytogenes. However, we found that the SLE was not quite effective as chlorine on L. monocytogenes, reducing 2 Log CFU/g of L. monocytogenes by chlorine solution at 200 ppm concentration (Brackett, 1987).
No significant differences in VBN values were observed between the control and treatment groups until day 3 of storage (Table 2). However, on days 5 and 7, the VBN values were significantly lower in the LSLE than in the LCON. The VBN value of the chicken breast was increased significantly upon prolonged storage. The spoilage bacteria or enzymatic degradation of proteins in meat resulted in an increase in VBN values (Kruk et al., 2011). These results were associated with the gradual increase in the microorganism population in the chicken breast during storage (Fig. 3). Indeed, the VBN value is highly related to the population of microorganisms (Sujiwo et al., 2018). In Korea, the acceptable VBN value for fresh meat is below 20 mg/100 g (Min et al., 2007). In this study, the VBN value in the LSLE on day 7 of storage was 19.90 mg/100 g, whereas that in the LCON was 24.38 mg/100 g. This result suggested that, according to the VBN value, the chicken breast in the LSLE was still acceptable, whereas in the LCON, the meat started to decay on day 7.
The TBARS values did not show significant differences in any of the samples until day 3 of storage (Table 2). However, the TBARS values in the LSLE on days 5–9 were significantly lower than those in the LCON. The TBARS values in the chicken breast in this study were slightly lower than those reported by Kruk et al. (2011), which were 0.28 mg MDA/kg on day 1 and 0.47 mg MDA/kg on day 7 of storage. The higher TBARS values indicated higher levels of lipid oxidation. On days 5–9, lipid oxidation was higher in the LCON than in the LSLE. Lipid oxidation produces lipid hydroperoxides by fatty acid autoxidation, and these lipid hydroperoxides then decompose into products such as aldehydes, alkenes, ketones, and alcohols, which are responsible for the off-flavor and off-odor of meat (Knox et al., 2008). These off-odors can be detected by consumers when TBARS values are in the range of 0.5 to 1.0 mg MDA/kg (Knox et al., 2008) because of the formation of ketones and aldehydes. From our results, we assumed that SLE reduced MDA formation via suppression of lipid oxidation and reduction of the microbial population, including L. monocytogenes.
Conclusion
In this study, we demonstrated that dipping of chicken breast in lyophilized plant extract solutions is effective for maintaining meat quality and reducing L. monocytogenes contamination in the meat. Treatment with SLE yielded the best results. SLE could maintain the pH value of chicken breast until day 5 of storage, and the L. monocytogenes population in the LSLE was also significantly lower than that in the other treatment group. The VBN and TBARS values in the LSLE were significantly lower than those in the control and other treatment groups. SLE treatment also effectively maintained the quality of chicken breast and reduced the TVC and L. monocytogenes count. Therefore, our findings suggest that SLE could be useful as a natural antimicrobial compound for reducing microbial contamination on the chicken meat surface, while the SLE was not effective as synthetic sanitizer in chicken meat industry. Further study should be needed to find a synergist to improve antimicrobial activity for a large scale in the chicken meat industry.