Introduction
Processed cheese (PC) is traditionally manufactured by adding natural cheeses along with emulsifying salts (ESs), water, NaCl, fat and other ingredients to the cheese system, followed by stirring and heating the blend. The commonly used temperature and time ranges in PC manufacture are from 70°C to 95°C and from 4 to 15 min, respectively. Moreover, mixing speeds also range from 50 and 3,000 rpm in PC manufacture. However, these ranges depend on the formulation of the desirable resultant product (Fox et al., 2000).
ESs, which are basically one of the main ingredients for PC manufacture, are used to improve emulsifying capacity of milk proteins. They have many properties to solubilise and hydrate milk proteins during PC manufacture after chelating Ca2+ from para-casein network. Moreover, the solubility of milk proteins relates to surface hydrophilic and hydrophobic interactions. However, the protein is more hydrated than in the natural cheese because of the addition of ESs to the PC blends. Therefore, the addition of ESs gives greater differences to processed cheeses (PCs) (Fox et al., 2000; Pelegrine and Gasparetto, 2005).
Natural cheese is one of the other important ingredients. Various types of natural cheeses are used to manufacture PC, depending on the country, availability of natural cheeses and marketing (Kapoor and Metzger, 2008). Guinee et al. (2004) reported that the functionality of natural cheese is dynamic for PC manufacture, with the different functional attributes undergoing particular changes during ripening.
Traditional village cheese is also made from semi-skimmed milk and/or skimmed milk with rennet casein by local people in various parts of Turkey in order to obtain economic value and/or cost reduction for their life-expenses. This cheese is a common and semi-soft cheese with a white or whitish colour. It sometimes contains small eyes in the inside of the cheese depending on the quality of milk, and is held in brine (sodium chloride 12%) during ripening for 30 d. The flavor of the traditional village cheese tends to become slightly tasteless and salty. Moreover, the village cheese with a low price is used to reduce the expenses of processed Kashar cheeses in Turkey. It also abounds in everywhere in Turkey to use it in the PC manufacture. A semi-hard and popular traditional Kashar cheese with a yellow colour is one of the most consumed cheeses in Turkey. There is no small eyes in the inside of Kashar cheese. Kashar cheese is also classified either as fresh Kashar or aged Kashar in terms of ripening according to Turkish Standards (Anonymous, 1999). Nowadays, because of reducing expenses of Kashar cheese, it is manufactured with a processed cheese cooker instead of the production of traditional Kashar which has less yield and longer manufacturing time as compared to the production of processed Kashar cheese.
PC has also manufactured for diversities, convenience, preservation and cost reduction for more than two decades. Recently, the use of food ingredients has widened the window for incorporation into PC. All ingredients, processing methods and equipments used in PC manufacture are quite versatile (Guinee et al., 2004; Lee and Anema, 2009). The compositional properties of the ingredients can also use to improve some functional and textural properties of the desired resultant PC (Lee and Anema, 2009). Besides these, texture is one of the substantial quality evaluations of PC, with its development being completely associated with the biochemical and physicochemical changes, such as in ionic equilibria occurring during ripening and/or storage (Lucey et al., 2003; O’Mahony et al., 2005). However, one of the main factors influencing characteristics of processed cheese is also pH values of natural cheeses. pH has marked influences on the structure and texture of PC. Due to these reasons, PC is usually prepared at a particular pH value of the resultant product. Final pH of PC also regards to the solubility and interactions of milk proteins, as well as the hydrolysis of ESs. It usually ranges from pH 5.4 to pH 5.9, mainly depending on all the types of PCs (Guinee et al., 2004; Lee and Anema, 2009; Lu et al., 2007). Recent studies have shown that the cooking pH values can markedly influence the interactions of milk proteins. These interactions could have a remarkable effect on the textural characteristics of the PC (Anema and Li, 2003; Donato and Dalgleish, 2006; Lee and Anema, 2009; Rodriguez del Angel and Dalgleish, 2006). Moreover, some experiments were also conducted in PCs with a variation of many treatments (Kapoor and Metzger, 2008). Lee and Anema (2009) reported that the textural properties of the processed cheese spread samples can be manipulated by the cooking pH, which can have a marked effect on the textural properties of the processed cheese spreads to be. The kinetics of protein denaturation and aggregation is also controlled by the heating conditions and the chemical environment with the pH which is probably the most important factors in determining the rate and extent of protein denaturation and the degree of the subsequent interaction of the whey proteins with the casein micelles (Anema and Li, 2003; Corredig and Dalgleish, 1996). All these differences could be likely due to the usage of different pH values of cheese, different ESs, different types and ages of cheeses, and different conditions for PC manufacture.
The main purpose of present study was to investigate the effect of the varying cooking pH values (5.3, 5.4, 5.5, and 5.6) on the textural properties for the processed cheese with village cheese (PCV), in comparison to the processed cheese control (PCC) which contained no traditional village cheese during 90 days of storage.
Materials and Methods
Fresh curd (52.9% dry matter, 27.3% fat, 0.23% salt, and pH 5.30), 2 mon aged traditional village cheese (39.9% dry matter, 1.50% fat, 0.94% salt, and pH 4.85), rennet casein (93.9% dry matter) were used as raw materials for PC manufacture. All chemicals, reagents and used solvents were analytical grades and were purchased from Merck (Darmstadt, Germany). Joha® PZ7 (sodium citrates, potassium orthophosphates, and polyphosphates), Joha® PZ14 (sodium citrates, orthophosphates, and polyphosphates) and Joha® Tneu (orthophosphates) were purchased Joha (Benckiser-Knapsack, Ladenburg, Germany).
The traditional village cheeses were made by local villagers individually in the Eastern Turkey according to same manufacture schedule. After filtrating raw milk, full-fat cow’s milk, which was obtained from a local farm, was separated from milk fat until it was semi-fat milk (~1%). Semi-fat cow’s milk was applied to heat treatment at ~55±1°C for 20 min by local village cheese producers. After heating, it was cooled to ~32±1°C. Then diluted rennet liquid [10 fold (strength 1:10.000)] added to the milk which was stirred into every tinned container of 20 kg milk. Then, it was held on 90 min for coagulation. The curd was cut into small pieces (0.5 cm3), especially square-shaped pieces, with a long knife. Afterwards, it was held on for 15 min. After syneresis, hot water (90±1°C) was added to the curd until the temperature of the curd reached by ~42±1°C. The curd was held for drainage (3 h), and then it was brined (sodium chloride 12%) at 5°C for 1 mon. Afterwards, the village cheeses were packaged with vacuum and stored at 5°C for further 1 mon.
The fresh curd was manufactured in Seker Dairy Plant in Konya. The fat content of milk was standardized to 3.4% as full-fat milk. The milk was pasteurised at 68°C for 30 min. Then, it was cooled to 38°C. Thermophilic starter culture (Christian Hansen TCC-3; 0.7 w/v) and calcium chloride (2 mg/kg) added to the full-fat milk. When pH of the milk was at 6.3, diluted rennet liquid (10 fold) added to the milk. The curd was cut 45 min later with a curd knife in the shape of 1 cm3 cubes. It was also allowed to settle in 10 min. Heating applied to the curd until the temperature of the curd reached from 35°C to 38°C within 20 min. While heating within 20 min, the curd was also being agitated, and rate of the increasing temperature for each 1°C was carried out within 6 min. At the end of heating treatment, whey was drained from the batch at pH 6.0. The fresh cheese curd was fermented by pH 5.2 value. Then, the remaining whey was redrained again.
The processed samples with and without the use of a traditional village cheese were produced in the food processing facilities of Food Engineering Department, Agriculture Faculty, Selcuk University. General target chemical composition for the PCV and PCC blends was 49% dry matter and 19% fat. This chemical composition of the PC samples was targeted to the ideal composition of processed Kashar cheese which is the one of most consumed cheeses in Turkey (Temizkan et al., 2014).
The percentage of added ingredients for the PC samples is presented in Table 1. The curd and the traditional village cheese were grinded to a size of <0.5 cm before PC manufacture. The PCV samples were prepared from the curd (~70%), the traditional village cheese (~30%), ESs (2.5% w/w) (ratio of Joha® PZ7: Joha® PZ14=3:2), distilled water and NaCl (2%) under the varying cooking pH values using a pilot-scale processed cheese cooker (10 kg capacity) equipped with indirect steam injection and a scraped surface stirrer (Intermak, Konya, Turkey). The processed cheese cooker had also temperature and speed settings using for heating and stirring the blends. The addition of Joha® Tneu emulsifying salt was used to increase pH values of all PC blends from their pH values to the aimed cooking pH value (5.3, 5.4, 5.5, and 5.6). Before adjustment of the cooking pH value, the blend was stirred at 7,265×g for 3 min at 50°C during processing. After adjusting the pH values of the blend, β-carotene (20% v/v; 0.05% v/v) as a colouring agent was added to the blend at 70°C. The blend was cooked at a temperature setting of 85°C for 4 min at 36,330×g. After cooking, the melted PC samples were filled into 7 tin containers (1,000 g) for each trial. On the other hand, the PCC samples were made with the fresh curd (100%) and rennet casein along with other same ingredients used for the PCV samples, under the varying cooking pH values with same circumstances. After processing, all PC samples therein tins were kept at 20±2°C for 1-d ripening in order to gain a desirable texture, colour and flavor of typical Turkish Kashar cheese. After 1 d of storage, the PC samples were removed from the tins, and packaged with cryovac shrink-vacuum bags. They stored at 4°C up to 90 days. All PC samples as a batch were manufactured in duplicate. All batches of the PCs were prepared randomly by using the same processed cheese cooker. The PC samples were analysed on up to 90 d for the chemical and textural properties. The PC samples (PCV or PCC) were also coded according to the cooking pH values, such as PCV-5.3 or PCC-5.3 for the 5.3 cooking pH value. The rest of other samples were also coded similarly like those, such as PCV-5.4 or PCC-5.4, PCV-5.5 or PCC5.5 and PCV-5.6 or PCC-5.6.
After being received at the laboratory (delivery at 4°C), for chemical compositional analyses, the PCs were sampled at d 1 while PCs were kept at 20±2°C for gaining a desirable texture, colour and flavor. They were also sampled at d 90 of storage. However, for pH measurement the PCs were also measured on different days of storage (at 1, 7, 15, 30, 60, and 90 d). When taking the sample, the particular block was cross-cut and a 200 g inner (core) part and a 200 g outer (adjacent to the foil) part of a slice was taken in the case of every measuring cheese. Moreover, for textural analyses, they were sampled on varying days of storage (at 7, 15, 30, 60, and 90 d). At each chemical compositional sampling, a 5-cm piece of cheese was cut from the exterior face of the block; the outer layer (0.5 cm) of the piece was discarded, and the rest of it was used for the compositional analyses. For textural analyses, a half of rectangular block of the cheese was used for each textural sampling time after the outer layers (0.5 cm) of the block were discarded.
After sampling, grated all PC samples were analyzed for dry matter, fat, protein, ash and salt contents. The samples were analysed for moisture and ash contents using Gravimetric methods, fat content using the Gerber method, and protein content using the Kjeldahl method by using a total nitrogen to protein conversion factor of 6.38 (Marshall, 2005). Salt contents of the PC samples were also determined according to the procedures described by Marshall (2005). pH values of the PC samples were measured directly at ambient temperature using a 315i/SET pH-meter equipped with a sentix glass electrode (WTW, Weilheim, Germany). The measurements of the samples were conducted at least three times for each trial.
The textural properties of all PC samples were measured at 20±1°C at d 7, 15, 30, 60, and 90 by a texture analyzer (model Universal TA.XT2, Texture Technologies Corp., 18 Fairview Road, Scarsdale, NY 10583, USA) fitted with a 5 cm diameter cylinder probe loaded with Nexygen TM software program. The texture analyzer was equipped with a 25-kg load cell for texture profile analysis (TPA). All PC samples were kept at 20±1°C for about half an hour before testing. The dimension of the PC sample was at 5×5 cm. The speed of crosshead was set at 1 mm/s in both upward and downward directions between two cycles. Each sample was subjected to two subsequent cycles (bites) of compression-decompression. The PC samples were uniaxially compressed to 40% of their original heights. The parameters of TPA were calculated as described previously (Van Vliet, 1991). Hardness (g) was measured as the force at maximum compression on the first cycle. Fracturability (g) was measured as the force per unit area at the point of fracture on the first cycle. Adhesiveness (g/s) was calculated as the negative area after the first cycle in the texture profile curve as described and expressed in absolute values (Van Vliet, 1991). Cohesiveness (dimensionless) was calculated as the ratio of the area of the second cycle to that of the first cycle. Gumminess (g) is an energy required to disintegrate a semi-solid food to a state ready for swallowing (Szczesniak, 2002). Springiness (dimensionless) was calculated as the ratio of distance of the second cycle (peak) to the distance of the first cycle (peak). Chewiness (g/cm) was calculated as the product of hardness×cohesiveness×springiness. Resilience is tendency of the PC sample to recover to its original dimensions upon removal of the applied force (Kapoor and Metzger, 2008). Eight textural parameters were obtained from TPA of those force-distance curves. TPA was conducted at least six times for each trial.
All samples were prepared on two separate occasions, with all analytical measurements conducted at least three times. The results are given as mean values±standard errors. A two-way analysis of variance (ANOVA) was performed. SPSS 13.0 for Windows SPSS Inc was used for all analyses (Chicago, IL, USA). Duncan’s multiple comparison test was used to determine significant differences between the various treatments. The results with p<0.05 were considered significantly different.
Results and Discussion
In the present study, the chemical compositions for the PCV and PCC samples are shown in Table 2. These results of the chemical properties are expected because all PC samples were adjusted for dry matter and fat contents inferentially as indicated before. The mean values of dry matter, fat and ash contents for the PC samples increased significantly at d 90 due to having 1-d ripening at the ambient temperature to obtain a desirable texture, colour and flavor of typical processed Kashar cheese after PC manufacture for all PC samples (p<0.05). Moreover, the mean values of dry matter and ash contents (51.9±0.22 and 6.38±0.07, respectively) for the PCV samples were higher than the values (49.9±0.22 and 5.56±0.10, respectively) for the PCC samples. This could possibly result from the further addition of phosphate-based emulsifying salt into the PCV blends to increase their first pH values to the desired cooking pH values, because the pH values for the PCV blends were lower than the values for the PCC blends during processing. Due to historical low pH values of the PCV blends, the further addition of phosphate-based emulsifying salt into the blends would increase the means of dry matter and ash contents in the PCV samples. Besides, the mean dry matter contents for all PC samples were in agreement with dry matter contents of the Kashar cheeses (Temizkan et al., 2014). The increasing in the dry matter contents of the PC samples over time is also in agreement with one study studied by Kocak et al. (1996). Moreover, there were no significant differences between these values of the PC samples according to the differences between the cooking pH values (p>0.05). However, the highest mean of ash contents of the PC samples observed in which cooked at pH 5.6 during processing. Besides all these, the mean values of the other chemical compounds’ of the PC samples were similar to each other’s between the PC samples (p>0.05). The means of fat, protein and salt contents of the PCV samples were 18.3±0.10, 20.9±0.32, and 3.06±0.11 whereas the values of the contents of the PCC samples were 18.3±0.14, 20.9±0.28, and 3.00±0.06, respectively. The changes at the chemical compounds in the present study are also in agreement with previous study by Cheng et al. (1997). Cheng et al. (1997) also reported that an alteration in dry matter content in cheese depended on the type of added salts.
The pH values of the PCV and PCC samples are shown in Fig. 1. The pH values of the PC samples slightly increased over time. The mean value of pH (5.70±0.02) for the PCV samples was higher than the pH value (5.64±0.02) for the PCC samples, due to the further addition of phosphate-based emulsifying salt into the PCV blends in order to increase their first pH values to the desired cooking pH values during PCv-processing. There were significant differences in pH values during storage period (p<0.05). The highest mean pH value (pH 5.71±0.04) of the PC samples was observed at d 30 of storage, but the pH changed slowly after 30 days of storage (p<0.05). The reason for major changes of the pH value seen during d 15 and 30 of storage could possibly be due to higher hydrolysis of ESs. The slight increase in the pH for all PC samples over time could also be due to slow solubilisation of colloidal calcium phosphate (CCP) and the loss of lactic acid. Moreover, the hydrolysis of ESs, especially oligo- and/or poly-phosphates, creates new acid functions thus lowering the pH-value of the product and inhibits an eventual pH increase (Chambre and Daurelles, 1997). The pH values for all PC samples were higher than that pH value (pH 5.49) of Kashar cheese (Oksuztepe et al., 2009). The increase at the pH values for all PC samples is also in agreement with previous studies reported by Lucey et al. (2003) and O’Mahony et al. (2005). They reported that slow solubilisation of CCP causes a slow increase in pH (Lucey et al., 2003). O’Mahony et al. (2005) reported that the amount of insoluble calcium in the aqueous phase could increase the pH values of Cheddar cheese samples after ageing. However, in cheeses, where residual lactose is fermented, the production of lactic acid can balance the usual tendency for a pH increase, with the result that the pH of cheese may be stable (Singh et al., 1997).
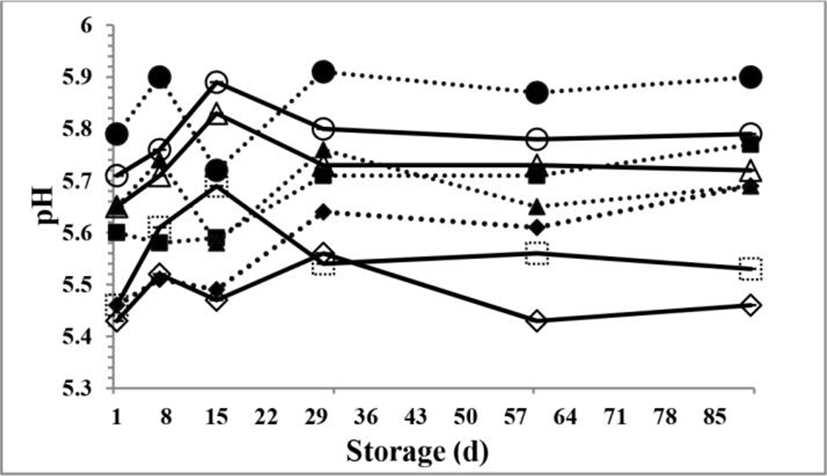
The changes in the textural parameters for all PC samples during 90 days of storage are shown in Fig. 2A, B,Fig. 3A, B,Fig. 4A, B, and Fig. 5A, B, respectively. However, there is no information available about the textural properties of processed Kashar cheese including a traditional village cheese. As seen in the figures, there were generally some changes in the textural properties for all PC samples at d 15 and 30 of storage, possibly due to the hydrolysis of CN and ESs because of the development of cheese texture which depends on the extent of proteolysis and hydrolysis of ESs and other components such as lactose (Fox et al., 2000). The hydrolysis of ESs occurs over time, and involves more gradual changes in the texture due to the small changes of pH values (Fox et al., 2000; Guinee, 2004). Moreover, the texture of processed cheese is influenced by many other factors, especially biochemical and physicochemical changes (Fox et al., 2000; Kapoor and Metzger, 2008; Tamime, 2011).
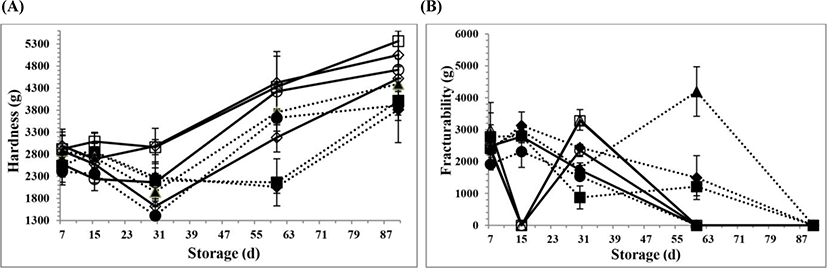
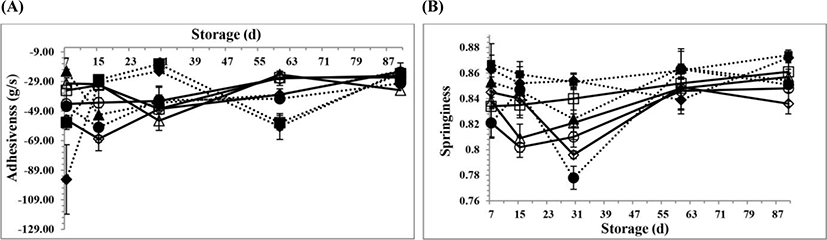
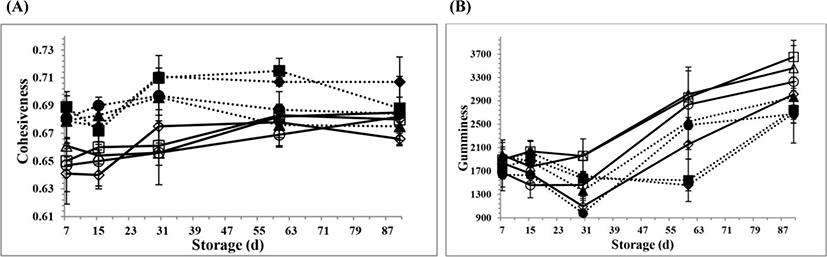
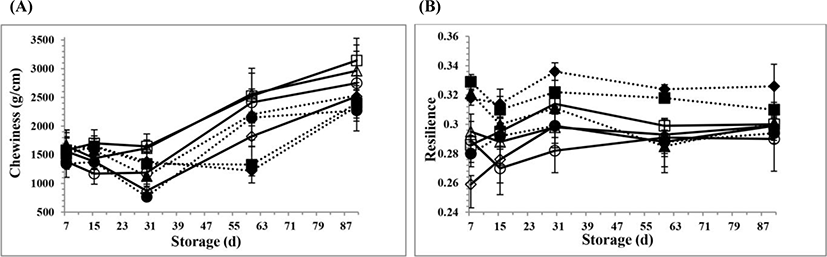
Hardness, which is one of the important parameters for assessment of overall quality, shows a resistance to deformation when the cheese sample is subjected to an external force. The hardness values for all PC samples increased over time due to the decrease of the pH values and the increase in the dry matter contents (Tamime, 2011). The mean hardness value (2,957±327 g) for the PCV samples was lower than the value for the PCC samples (3,377±180 g) because the addition of 2 mon aged traditional village cheese could possibly decrease the mean hardness value of the PCV samples, as a result that including the low level of intact casein content of the village cheese. As it is known, the primary phase of proteolysis occurs during the first 2 to 4 wk and involves weakening of the para-casein network, and finally results in softening of the texture especially hardness (Guinee, 2003; Irudayaraj et al., 1999; Lawrence et al., 2004; Lucey et al., 2003; O’Mahony et al., 2005). This is also related to a previous study reported by Kapoor and Metzger (2008). Kapoor and Metzger (2008) reported that when the intact casein content of the natural cheese used for PC manufacture decreased, hardness of the processed cheese could decrease because of the increasing level of proteolysis. Moreover, an increase in the hardness values for the PC samples over time could also be consistent with the increasing levels of dry matter, which act as an important role on the textural properties of the samples. An increase in the dry matter content could also reinforce the structure of the cheese networks, and increase the coherence of the protein matrix to become harder. However, the mean hardness value for the PC samples at the 5.5 cooking pH was the highest value among the all mean hardness values at the other cooking pH values (p<0.05). This reason may be likely due to the increase of an interaction between the milk proteins, whose structures could be possibly altered at the 5.5 cooking pH value during processing. One of the most remarkable effects on the cheese hardness is also that hydrolysis of peptide bonds releases two new charged groups that compete for water, decreasing the free water content of the cheese (Guinee, 2003; Irudayaraj et al., 1999; Lawrence et al., 2004; Lucey et al., 2003; O’Mahony et al., 2005). The textural changes of the cheese samples also became slower after 4 wk. These textural changes are also possibly attributed to the rate of biochemical changes and increase in pH (Gunasekaran and Mehmet, 2004). Moreover, the increase in the dry matter content on 90 d of storage also caused a restricting solvation of the para-CN matrix of the cheese which leads to an increase in some textural parameters in the cheese system, such as hardness, cohesiveness and springiness (Guinee, 2003; Irudayaraj et al., 1999; Lawrence et al., 2004; Lucey et al., 2003; O’Mahony et al., 2005). Beside all these, this study is also in agreement with one of the previous studies done by O’Mahony et al. (2005). They reported that the primary softening of Cheddar cheese texture occurring during the early stages of ripening (initial 2 wk) is mostly correlated with the transfer of casein-bound calcium phosphate to the aqueous phase of the cheese concentration of insoluble calcium (O’Mahony et al., 2005). There are also some agreements with the previous studies (Marchesseau et al., 1997; Lucey et al., 2003). Lucey et al. (2003) reported that the hardness values of the PC samples decreased with increasing the cooking pH values because of the increased electrostatic repulsions between CN micelles. Marchesseau et al. (1997) studied the microstructure of the processed cheese manufactured with a different resultant pH value. They found that a processed cheese with a lower pH (5.2) had increased interactions between milk proteins since the proteins were closer to the isoelectric point of CN. It was also founded that processed cheese at a higher pH (pH 6.1) had an open structure, and therefore a weaker emulsion observed (Marchesseau et al., 1997).
Fracturability is defined by an initial yield peak (Dimitreli and Thomareis, 2007). The mean fracturability value (1,714±205 g) for the PCV samples was higher than the mean fracturability value (1,186±219 g) for the PCC samples. Nevertheless, the fracturability values for all PC samples decreased over time (p<0.05). The reason of the decrease at fracturability values could possibly be due to the changes in the chemical composition and biochemical changes of the PC samples, especially the decrease of intact casein content. Even if the changes did not show a clear trend, the decrease in fracturability values may also be related to the hydrolysis of the ESs, which could lead to the small changes at the cooking pH values, and affected the textural properties of the PC samples at low temperature over time. Additionally, due to the fat content in the protein matrix, it could possibly make all the PC samples more elastic and less susceptible to fracture upon compression (Awad et al., 2002). Hence, all PC samples could be undeformable at 90 d of storage.
The adhesiveness, which is required a force to remove the material that adheres to the mouth during the normal mastication process, has an opposite trend to chewiness and gumminess (Awad et al., 2002). Adhesiveness also implies a decrease in elasticity, and an increase in water-protein matrix interaction (Costa et al., 2010). The mean adhesiveness values for the PC samples are seen in Fig. 3A. There were no detected significant differences at the adhesiveness values of the PC samples over time (p>0.05). As seen in Fig. 3A, the adhesiveness values for the PC samples during from 15 to 60 d of storage were higher than the adhesiveness values of the PC samples on the other days of storage, and then these values decreased on 90 d of storage. It was also supposed that the adhesiveness values for the PC samples could be probably influenced by the increase at the dry matter content, and the biochemical changes in the components of the PC samples, especially hydrolysis of the ESs and fat, the cooking pH values as well as the proteolysis of protein (Dimitreli and Thomareis, 2007). As seen in the Fig. 3A, the adhesiveness values for the PC samples could also be affected by the cooking pH values during processing (p<0.05). At the lowest cooking pH (5.3), the adhesiveness values for the PC samples were the lowest when compared to the values at the other cooking pH values (p<0.05). The reason may be due to the greater interaction between the milk protein and other components at 5.3 pH because they were closer to isoelectric point of CN. Nevertheless, some researchers have agreed that the pH range for a good-quality processed cheese should be between 5.4 and 5.8, even if it depends on all the types of processed cheese (Marchesseau et al., 1997).
Springiness, which refers to the elasticity, indicates the ability of the cheese samples to go back to its undeformed condition after removing of the external force, and depends on the emulsion state and mainly upon protein (Awad et al., 2002). The mean springiness value (0.849±0.008) for the PCV samples was higher than the value (0.834±0.003) for the PCC samples. This may be likely due to the addition of 2 mon aged village cheese into PCV samples. The springiness values for the PC samples increased over time, as seen in Fig. 3B. However, the springiness values on 30 d of storage for the PC samples decreased gradually due to possibly the increasing levels of hydrolyse of ESs and proteolysis (p<0.05). The PC samples at the highest cooking pH (5.6) had less mean springiness value when compared to the values at the other cooking pH values (p<0.05). This reason may also be due to the biochemical changes, as well as the changes of the bonds which could likely lead to some changes in protein-protein interactions. These mechanisms could also cause the decrease in springiness. This is also in agreement with one study done by Shirashoji et al. (2006). They also indicated that the proteins in the processed cheese are less close to each other’s when the pH was increased, due to the increase of net negative charges on the proteins. However, each type of ESs influences the CN micelles with different mechanisms (Mizuno and Lucey, 2005).
Cohesiveness is influenced by the strength of the internal bonds making up the cheese system (Awad et al., 2002). The cohesiveness values for all PC samples are seen in Fig. 4A. The mean cohesiveness value (0.690±0.003) for the PCV samples was higher than the value (0.664±0.003) for the PCC samples (p<0.05). There were no significant differences at the cohesiveness values according to the cooking pH values for all PC samples (p>0.05). The PC samples had the lowest cohesiveness values until 2 wk of storage. After the 2 wk of storage, the cohesiveness values for the PC samples increased with the increasing of storage (p<0.05). This could be due to excessive interactions between milk proteins. The cohesiveness values for the PC samples had opposite trends when compared to the gumminess and chewiness values for the PC samples, as seen in Fig. 4B and Fig. 5A, respectively. One previous finding done by Lane et al. (1997) has also been in agreement with the decrease in the cohesiveness value on 15 d of storage in this study. Lane et al. (1997) reported that the cohesiveness value among the textural properties of Cheddar cheese was more related to primary proteolysis with a trend of decreasing with increasing proteolysis.
The gumminess and chewiness values for the PC samples are seen in Fig. 4B and Fig. 5A, respectively. The gumminess and chewiness values for all PC samples had similar trends over time. The mean gumminess and chewiness values (1,964±94.4 g and 1,675±84.2 g/cm) for the PCV samples were lower than the values (2,255±127 g and 1,895±113 g/cm) for the PCC samples, respectively. However, the gumminess and chewiness values for all PC samples increased during 90 days of storage (p<0.05). The reason of the increase at the gumminess and chewiness values over time may be due to the increase in the dry matter content and the biochemical changes between the compounds of the PC samples. These mechanisms may possibly reinforce the protein-protein interactions in the PC samples. However, the interactions could also be slightly affected by small changes at the cooking pH values during processing. The changes at the cooking pH values could also increase the interactions between milk protein and other components in the PC systems over time. The PC samples at the 5.5 cooking pH value had higher gumminess and chewiness values when compared to the values of the PC samples at the other cooking pH values (p<0.05). This reason might be that the quantity to simulate strength of the internal bonds could build up the body of the PC samples. An increase in the interactions of milk proteins could also possibly result in a denser network at the 5.5 cooking pH value. So that, the changes at the gumminess and chewiness values of the PC samples were influenced by the cooking pH values during processing. When the pH value is shifted, different types of interactions in the cheese systems occur to different extents (Fox et al., 2000). Lee and Anema (2009) found that the dependency of pH with thiol-disulphide exchange reactions may also account for some changes of the textural properties for the PCs, depending on the cooking pH values. Therefore, the final pH value of the processed cheese has been found to have a substantial effect on the type of protein interactions in the processed cheese emulsion (Kapoor and Metzger, 2008; Lee and Anema, 2009; Marchesseau et al., 1997). Apart from these, this study could also be in agreement with previous work of Piska and Stetina (2004). They found that an increasing ratio of the mature raw material in the PC blends decreased the rigidity of the processed cheese which was expressed in terms of the textural parameters, such as hardness, gumminess and chewiness (Piska and Stetina, 2004).
The resilience values for all PC samples are seen in Fig. 5B. The mean resilience value (0.309±0.006) for the PCV samples was higher than the value (0.290±0.003) for the PCC samples. This reason may be due to the increasing proteolysis level in the PCV samples as a result of addition of 2-mo aged village cheese. The small difference between the compositions of the PC formulation could affect the resilience values, in regard to the interactions of the proteins. The resilience values for the PC samples also increased at d 30 of storage. However, the resilience values for the PC samples after 30-d of storage generally decreased with increasing of storage. The decrease in the resilience values after this time period may also be due to the decrease of pH values of PC samples and the increase of hydrolysis of ESs, and some biochemical changes such as ionic equilibria of the cheese networks (Tamime, 2011). The changes at the resilience values could be possibly related to over time. The PC samples at the low cooking pH values (pH 5.3 and 5.4 values) had higher resilience values than the values for the PC samples at the other cooking pH values (p<0.05). When the cooking pH was shifted from pH 5.3 to 5.6 during processing, the resilience values of the PC samples decreased, because of not close to isoelectric point of CN. Therefore, the small changes at the cooking pH may also possibly influence of the resilience values for all PC samples.
Conclusions
A small shift at the varying cooking pH values during processing could influence the interactions between cheese chemical compounds in the cheese systems over storage. This reason might be due to slight differences at both the chemical properties of the PC samples and the biochemical changes, which have markedly influenced the textural properties of the PC cheese. Thus, a small shifting at the cooking pH value can be an effective mean to alter the textural properties of a processed cheese. Consequently, the changes at the textural properties for the PC samples could possibly attribute to the varying cooking pH values during processing, especially the 5.5 cooking pH value. Using a traditional village cheese in the processed cheese manufacturing is also of considerable economic importance for processed cheese manufacturers, and gives a marketing opportunity to local cheese makers individually in Turkey. To understand these mechanisms well in the cheese system, furthermore new studies are needed to carry out in the future.