Introduction
Intramuscular fat (IMF) exists around muscle fibers embedded in connective tissue sheaths and is considered the final product of the fat deposition process. It is one of the primary indicators to evaluate muscle quality and positively influences quality traits of meat, including flavor, juiciness, and tenderness (Chikwanha et al., 2018; Pećina et al., 2018; Wang et al., 2020). In addition to the content of IMF in meat, the composition of those fatty acids has also received increasing attention due to its possible implications for human health. In most studies, there was a significant positive correlation between monounsaturated fatty acids (MUFAs) and IMF content, while a negative relationship existed between IMF and most polyunsaturated fatty acids (PUFAs; Razmaitė et al., 2020). There are different sources of fatty acids in the IMF, which are de novo synthesis by the animal body and direct intake of fatty acids from the diet (Bessa et al., 2015). As a meat-type sheep, the contribution of de novo synthesis to fat deposition is relatively small (Laliotis et al., 2010). Therefore, optimizing the deposition and composition of the IMF through sheep production and feeding strategies will provide new opportunities for mutton producers to enhance meat quality characteristics (Juárez et al., 2021).
The probiotics feed improves the digestive and absorption functions of the host animals and enhances their immunity by altering their intestinal microbiota, which has a positive impact on the host animals. Lactobacillus casei has been proven to possess cholesterol-lowering and immune-modulating effects, with beneficial effects on host health (Ayala-Monter et al., 2019; Wang et al., 2021). Lactobacillus plantarum has been confirmed to reduce lipid level, and improve the functionality of the gut microbiota (Bao et al., 2012; Li et al., 2020). The probiotic preparation (L. casei and L. plantarum) possess the ability to simultaneously reduce serum cholesterol levels and improve the fatty acid composition of meat and milk (Rabelo et al., 2016; Toral et al., 2018). Dev concluded that a compound L. casei preparation fed to sheep can increase body weight gain and strengthen immunity (Dev et al., 2021). After the mixed culture of Lactobacillus was fed to creole goats, tissues of the animals in the probiotics group exhibited an increase in MUFAs and PUFAs, including linoleic acid (C18:2), linolenic acid (C18:3), and cis-9, trans-11 conjugated linoleic acid (Taboada et al., 2022). Lamb in the prebiotic group had better antioxidant status and water-holding capacity, and the meat was a tenderer (Nie et al., 2022). From these perspectives, dietary probiotics seem to be a technical method to improve animal performance, fatty acid composition, and meat quality. However, the molecular regulatory mechanism by which probiotics feed affects the IMF content and fatty acid composition in Sunit sheep is unclear.
The adenosine 5′-monophosphate-activated protein kinase (AMPK) signaling pathway is a major metabolic pathway that regulates lipid decomposition and synthesis. On the one hand, when AMPK is activated, the cascade effect of the AMPK signaling pathway that regulates fat metabolism is activated. AMPK is phosphorylated to rapidly inhibit the activity of acetyl-CoA carboxylase (ACC), the expression of ACC is reduced, and the catalyzed malonyl-CoA by ACC is reduced [malonyl-CoA is the main component of de novo lipogenesis, and it is an inhibitor of Carnitine palmitoyltransferase 1 (CPT1; Fang et al., 2019; Ke et al., 2018)]. This cascade reaction controls the rate at which free fatty acids (FFAs) in the organism enter mitochondria for β-oxidation, suggesting a central role for the AMPK-ACC signaling pathway in the regulation of lipolysis and synthesis (Thomson and Winder, 2009; Zhang et al., 2021). On the other hand, as a downstream target gene for AMPK, the sterol regulatory element-binding protein-1c (SREBP-1c) is a transcription factor that regulates lipid homeostasis by controlling the synthesis of cholesterol, fatty acids, triglycerides (TG), and phospholipids (Ferré et al., 2021). AMPK can inhibit the activity of SREBP-1c by phosphorylation, and SREBP-1c can release the active fragment to bind to the SREBP-1c response element on its downstream target gene, fatty acid synthase (FASN), which theoretically regulates fatty acid metabolism (Fang et al., 2019; Tong et al., 2016; Yang et al., 2017). Based on this, we hypothesized that the AMPK signaling pathway is involved in the regulation of IMF and fatty acid composition in Sunit sheep by probiotics feed.
This study assumed that long-term supplementation with probiotics could affect the content of IMF and the composition of fatty acids of Sunit sheep through the AMPK signaling pathway is contribute to improving the nutritional value of meat. This study aimed to investigate whether probiotics feed can be an effective means of promoting IMF deposition in Sunit sheep and to further evaluate the role of the AMPK signaling pathway in this process, thus revealing possible potential mechanisms by which probiotics feed regulates IMF and fatty acid composition in Sunit sheep. This study provides new ideas and strategies for the application of probiotics in animal husbandry.
Materials and Methods
Twelve male sheep of ninety-six three-month-old Sunit sheep of similar live weight were randomly selected and divided into two groups for two different dietary treatments, one without probiotics (CON) and the other with probiotics (PRO). The foundation diet provided by the CON group consisted of a mixture of hay and concentrate composed primarily of cornmeal, fed at a rate of 1.5% of the sheep’s body weight. The PRO group was provided with the same basic diet but with 1% probiotics added to the concentrate. All experimental diets met the nutritional requirements of the sheep (Table 1). All sheep were individually housed in separate pens equipped with feeders. They were fed twice daily at 8:00 and 17:00. All sheep had ad libitum access to feed. Throughout the entire experimental procedure, no dietary selectivity occurred, and no instances of refusal to consume forage and/or concentrate were observed. The probiotics used in this study were a mixture of equal parts L. casei HM-09 and L. plantarum HM-10, which were provided by Inner Mongolia Agricultural University (China), the Key Laboratory of Dairy Biotechnology and Engineering of the Ministry of Education. Both of these probiotic strains were identified by screening fermented mare’s milk from Inner Mongolia.
2) Vitamin and mineral premix provided the following per kg of diet: 20,000 IU of vitamin A; 4,000 IU of vitamin D3; 80 IU of vitamin E; 16 mg of vitamin K; 2.5 mg of vitamin B1; 2.5 mg of vitamin B2; 8 mg of vitamin B6; 3.4 mg of vitamin B12; 54 mg of Ca-pantothenate; 25 mg of Cu; 36 mg of Zn; 58 mg of Mn; 0.5 mg of I; 0.3 mg of Co; 0.4 mg of Se.
All sheep were raised in a well-ventilated farmhouse with the same environmental conditions in Bayan Nur City, Inner Mongolia Autonomous Region, China. The light was provided naturally. The sheep were born on the farm and reared with their ewes until stopping breastfeeding about 90 days of age when they were raised alone. Sheep were allowed access to drinking water and salt blocks throughout the experiment. After 90 days of experimental feeding, all animals fasted for 12 hours and then were transported to a commercial slaughterhouse, where they were immediately slaughtered according to standard commercial ‘halal’ procedures by professional personnel (Abdullah et al., 2019).
On the last day of the experiment, all sheep were subjected to venous blood collection using a 10 mL vacuum blood collection tube containing anticoagulant in the morning. Blood collected was separated by centrifugation at 4°C at 3,200×g for 15 min, and serum was stored at –20°C for determination of TG, total cholesterol (TC), FFAs, high-density lipoprotein (HDL), and low-density lipoprotein (LDL; Childs et al., 2008). Serum indicators were detected using the Hitachi-3110 fully automated biochemistry analyzer.
The initial and final weight (the weight of the sheep at the end of the experiment) of the sheep were recorded to evaluate the average daily gain. The daily feed intake of each sheep was recorded to evaluate the average daily feed intake. After slaughter, the carcass weight of each sheep was used to calculate the dressing percentage of the animals. After carcass segmentation, the characteristics of the relevant carcasses were measured, including the thickness of the back fat at the 12th rib, and the area of the longissimus thoracis (LT) muscle between the 12th and 13th ribs on the left side. The bones were separated from the muscle and fat tissues and weighed to calculate the net weight of meat and carcass yield.
Approximately 5 g of the LT muscle sample was placed in sterile tubes, frozen in liquid nitrogen, and stored at –80°C for further analysis. In addition, the remaining sample of LT muscle was placed in a polyethylene sample bag and stored at –20°C until the analysis of IMF, TG, and the composition of fatty acid.
The IMF content in the LT muscle was detected by the Soxhlet petroleum-ether extraction method based on the Chinese National Standards GB/T 5009.6.2016. The IMF contents in LT muscle were detected by the Soxhlet petroleum-ether extraction method based on Chinese National Standards GB/T 5009.6.2016. Homogenized tissue samples were prepared in physiological saline solution (tissue weight: physiological saline solution=1:9), centrifuged for 10 minutes, and the TG content was determined using the GPO-PAP method reagent kit (A110-1-1 triglyceride assay kit, Nanjing Jiancheng Bioengineering Institute, Nanjing, China).
The composition and contents of fatty acids were detected in the way described by Folch et al. (1957). Samples of fatty acids were extracted from 5 g of meat using chloroform: methanol (2:1, v/v), filtered, and mixed with 20% sodium chloride, after shaking and standing, the chloroform lipid fraction was retained. After rotary evaporation, the lipid extracts were saponified and methylated. The upper solution was absorbed into the injection bottle and ready for gas chromatography analysis. Gas chromatography (Agilent/GC8860, Agilent, Santa Clara, CA, USA), flame ionization detector, automatic sample injection, and capillary column were used for detection. The initial temperature setting of the column oven is 120°C, kept for 5 min, then increased to 250°C at a rate of 10°C/min and maintained for 28 min. The carrier gas used is nitrogen. The fatty acid analysis was performed with a split ratio of 1:20, constant flow mode, with an average speed of 28 cm/s. The relative proportions of fatty acids were identified by comparing the peak areas with the reference standard 37-component FAME mix from Supelco Analytical (Sigma-Aldrich, Darmstadt, Germany).
Total ribonucleic acid was extracted from LT muscle samples using the RNAiso Plus reagent (No. 9109, Takara, Kusatsu, Japan). Reverse transcription was performed using the PrimeScriptTM RT reagent kit (No. RR047, Takara). The Roche LightCycler 96 QPCR system and TB GreenTM Premix Ex TaqTMII (No. RR820A, Takara) were used for real-time PCR. The β-actin gene was used as a reference gene. The PCR data analysis program was used to quantify the Ct values, and the mRNA expressions were calculated using the 2-ΔΔCt method. The primers for quantitative real-time PCR are shown in Table 2.
Following the homogenization of the longissimus dorsi muscle tissue, the supernatant was collected, and the activity of p-AMPKα2, p-ACCα, CPT1B, SREBP-1c, FASN and SCD1 in the LT muscle tissue was measured using ELISA kits from Wuhan Xinqidi Biological Technology (Wuhan, China). The experimental procedures were conducted according to the instructions provided with the kits.
Using IBM SPSS Statistics 22.0 to perform normality tests on the data, independent sample t-test analysis is used to analyze differences in group statistics. Partial least squares discriminant analysis (PLS-DA) was used to identify the main factors that affect the composition of the fatty acids. Data are presented as the mean±SEM. Extremely significant was defined as p<0.01, significance was defined as p<0.05, and trends were defined as 0.05≤p<0.10.
Results
As shown in Table 3, the loin eye area (LEA) of the LT muscle in the PRO was significantly higher than that of the CON (p<0.05). Compared to CON, PRO also showed an increasing trend in final weight and carcass weight, while carcass length showed a decreasing trend (0.05≤p<0.10). There were no significant differences in other traits of growth and slaughter performance (p>0.05).
From Table 4, it can be concluded that the PRO showed significant decreases in plasma levels of TG, HDL, LDL, and FFA compared to the CON (p<0.01). On the contrary, there were no significant differences in TC levels between CON and PRO (p>0.05).
The effect of probiotics feed on IMF and TG levels in Sunit sheep is reflected in Table 5. It can be seen that compared to the CON, the PRO showed a significant increase in IMF content (p<0.05). It should be noted that the significant trend in TG and IMF is the same (p<0.01).
Item | Treatment1) | SEM | p-value | |
---|---|---|---|---|
CON | POR | |||
IMF (g/100 g) | 0.46b | 0.57a | 0.04 | 0.024 |
TG (mmol/g prot) | 2.98b | 3.74a | 0.25 | 0.006 |
The probiotics feed to a certain extent ameliorated the fatty acid profiles in the LT of the Sunit sheep, as shown in Fig. 1 and Table 6. Compared to the CON, the proportion of MUFA and n-3 PUFAs in the PRO increased significantly (p<0.05). Further analysis found that probiotics supplementation with feed significantly decreased the proportion of capric acid (C10:0) and (C14:0; p<0.05), greatly increased the percentage of myristoleic acid (C14:1; p<0.01), and dramatically raised the proportion of oleic acid (C18:1n9 cis; p<0.05). At the same time, the effect of probiotics on the profile of PUFAs was more pronounced, with a substantial increase in the proportion of arachidonic acid (C20:4n6; p<0.01), and a considerable increase in the percentage of γ-linolenic acid (C18:3n6), α-linolenic acid (C18:3n3), cis-8,11,14-eicosatrienoic acid (C20:3n6), cis-5,8,11,14,17-eicosapentaenoic acid (EPA, C20:5n3) and cis-15-tetracosenoic acid (C24:1n3; p<0.05), while the proportion of linoleic acid (C18:2n6) showed slowly increasing trend (0.05≤p<0.10).
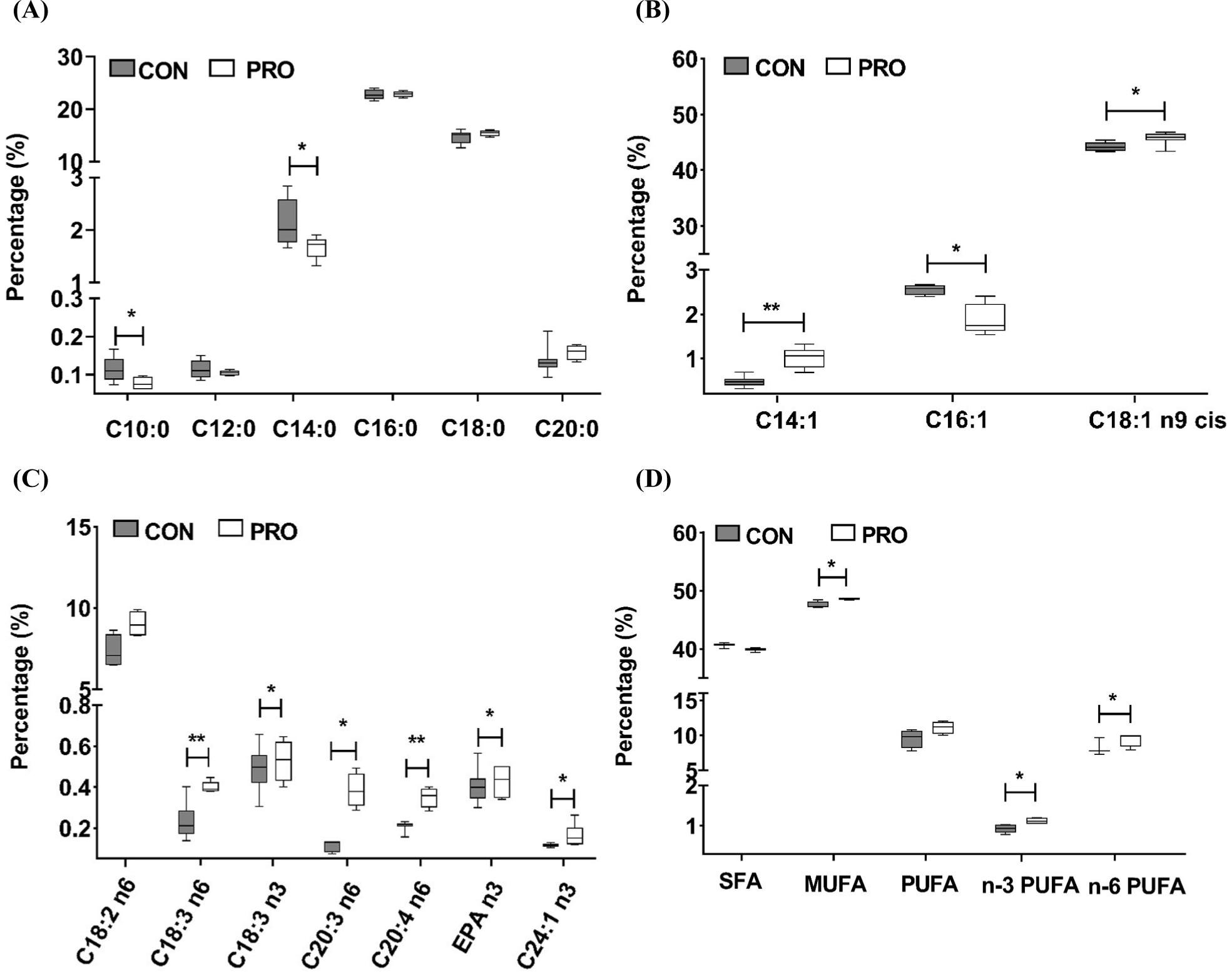
In this study, PLS-DA was applied to elucidate the clustering and overall differences among different groups of fatty acids. There was some overlap between the CON and the PRO groups of fatty acids, but not complete consistency. The scores of the first two factors of PLS-DA are shown in (Fig. 2A). The first principal component factor (PC1) explained 35.7% of the variance, and the second principal component factor (PC2) explained 21.5% of the variance. Based on the PLS-DA loading plot shown in (Fig. 2B), C16:1, C14:1, C24:1n3, 8,11,14-eicosatrienoic acid (C20:3n6), C18:3n-3, and C18:2n6 had higher loading coefficients on PC1, indicating a strong association with PC1. At the same time, C14:1, C24:1n3, and C20:3n6 showed a positive correlation with PC1, while C16:1, C18:3n-3, and C18:2n6 showed a negative correlation with PC1. Meanwhile, PC2 had a close relationship with C18:1n9 cis, arachidic acid (C20:0), C18:3n6, lauric acid (C12:0), C14:0, and hexadacanoic acid (C16:0), among which only C18:1n9 cis showed a negative correlation with PC2, while the other indicators showed a positive correlation with PC2. It is worth noting that PC1 mainly described unsaturated fatty acids (UFAs), while PC2 was described by both saturated fatty acids (SFAs) and UFAs.
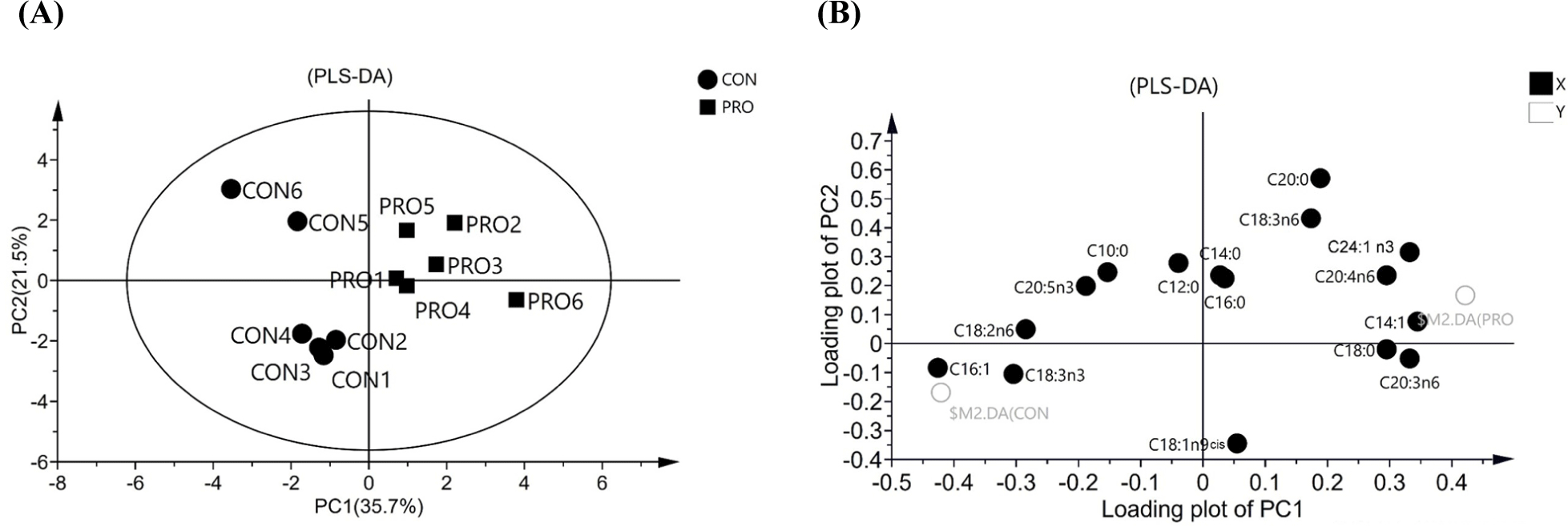
The levels of mRNA expression of genes associated with fatty acid metabolism, including the synthesis and oxidation of fatty acid, are shown in Fig. 3. The expression of AMPKα2 mRNA, a key transcription factor involved in energy metabolism, was significantly lower in the PRO compared to the CON (p<0.05), while the expression of SREBP-1c mRNA, a key transcription factor involved in lipid metabolism, was significantly higher in the PRO (p<0.01). Furthermore, compared to the CON, the addition of probiotics feed significantly upregulated the expression of ACCα mRNA, an intermediate metabolite that plays an important role in fatty acids synthesis and metabolism (p<0.01). Conversely, the expression of CPT1B mRNA, a rate-limiting enzyme in the oxidation of fatty acids, was significantly downregulated by probiotics feed (p<0.01). Similarly, the expression of FASN mRNA, a fatty acid synthesis enzyme, was significantly upregulated in the PRO (p<0.05). Moreover, the expression of stearoyl-CoA desaturase 1 (SCD1) mRNA, the enzyme responsible for desaturating SFAs into MUFAs, was also significantly upregulated in the PRO (p<0.01).
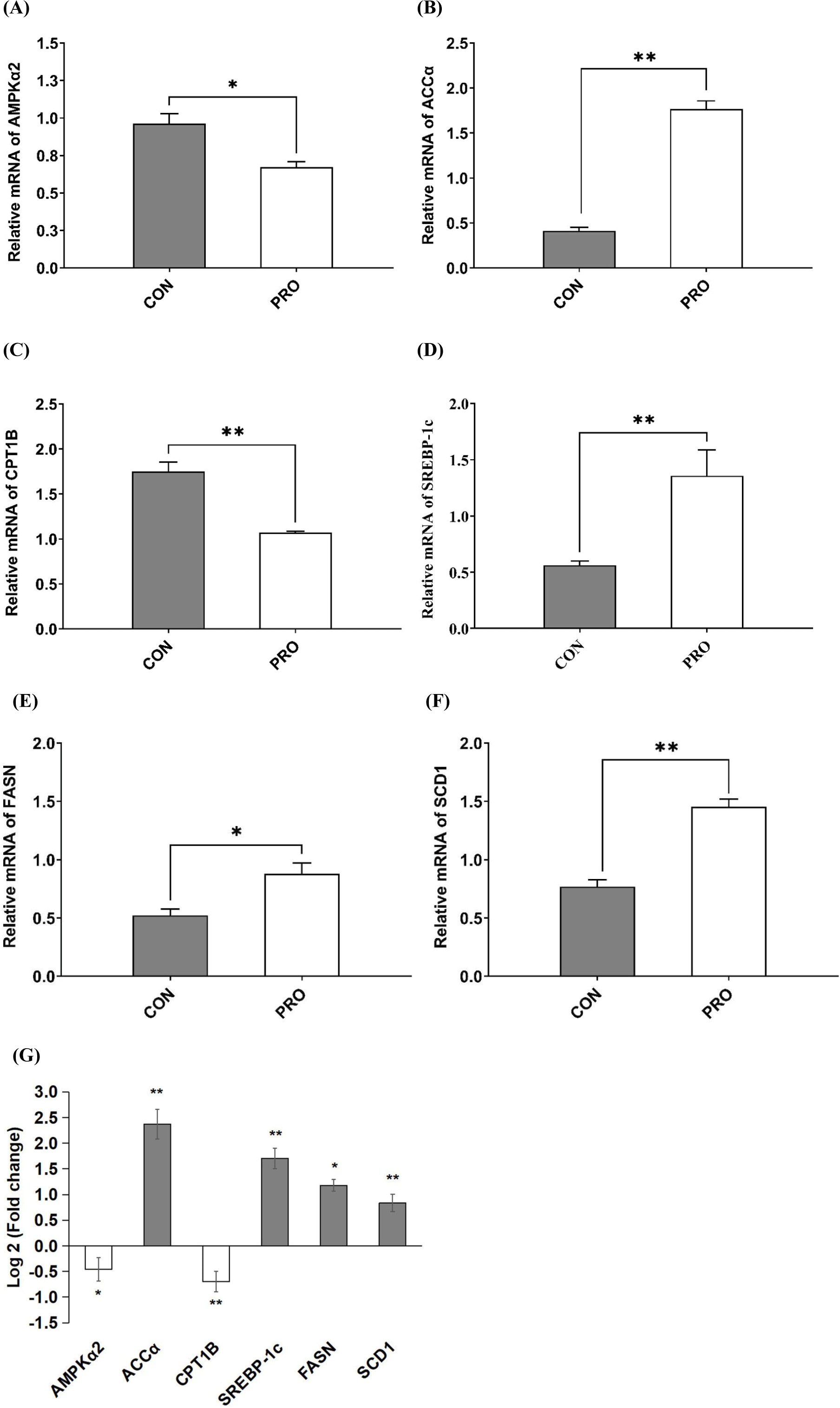
The impact of probiotics feed on the key molecular activity of enzymes related to lipid metabolism in Sunit sheep is illustrated in Fig. 4. Based on the figure, it can be observed that the activity of p-AMPKα2 and CPT1B in the PRO was significantly reduced compared to the CON (p<0.05), whereas the activities of p-ACCα, SREBP-1c, FASN, and SCD1 were significantly increased (p<0.01). These findings demonstrate a similar trend to the mRNA expression results mentioned earlier.
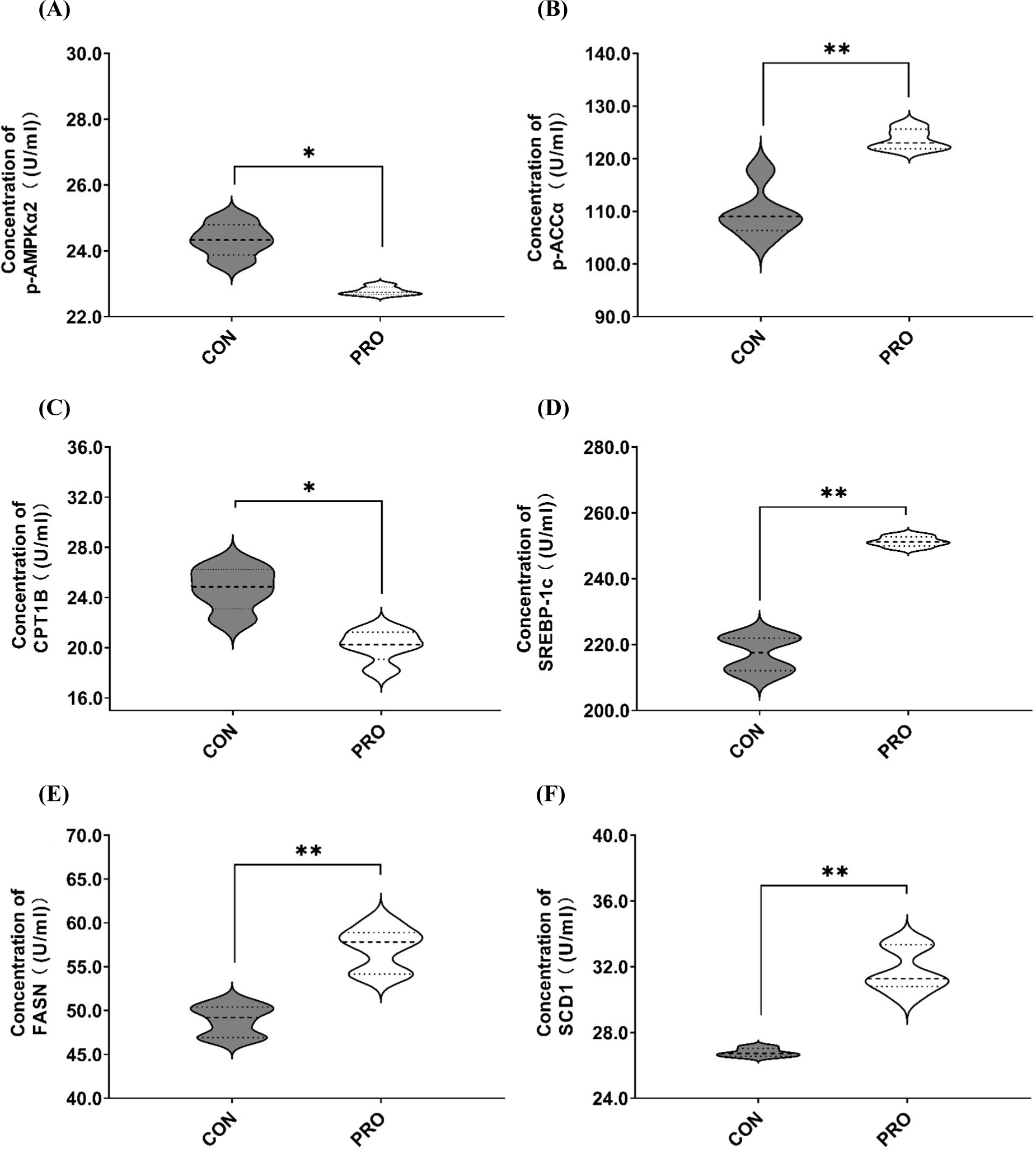
Pearson’s correlation analysis was conducted to determine the correlation between lipid composition parameters and gene expression. As shown in Table 7, AMPKα2 showed significant negative correlations with IMF, C14:1, C18:3n6, C20:3n6, and C20:4n6, while ACCα exhibited significant positive correlations with C14:1, C18:2n6, C18:3n6, C20:3n6, C20:4n6, and C24:1n3. CPT1B showed significant negative correlations with C14:1, C18:2n6, C18:3n6, C20:3n6, C20:4n6, and C24:1n3. Additionally, SREBP-1c exhibited significant positive correlations with C14:1, C20:3n6, and C20:4n6. As predicted, FASN showed a strong positive correlation with IMF, while SCD1 demonstrated a significant negative correlation with C10:0, and significant positive correlations with C18:3n6, C20:3n6, and C20:4n6.
AMPKα2, adenosine 5’-monophosphate-activated protein kinase alpha 2; ACCα, acetyl-CoA carboxylase alpha; CPT1B, carnitine palmitoyltransferase 1B; SREBP-1c, sterol regulatory element binding protein-1c; FASN, fatty acid synthase; SCD1, stearoyl-CoA desaturase 1; IMF, intramuscular fat; TG, triglycerides.
Discussion
The nutritional and dietary value of sheep is largely determined by its feed, which also affects consumer health by influencing the IMF content and the composition of fatty acids. Probiotics, as beneficial feed additives, are widely used in livestock production. Therefore, it is necessary to evaluate the effects of probiotics feed on growth performance, carcass characteristics, plasma biochemical parameters, and muscle lipid indicators, including IMF and TG content, fatty acid composition, and the expression of the lipid-related genes and the activity of the enzyme of sheep, in order to provide reasonable recommendations for intensive livestock production.
The present study revealed that probiotics supplementation in Sunit sheep resulted in an increasing trend in final weight and carcass weight, along with a significant improvement in the LEA, which is consistent with previous research findings (Lu et al., 2021). LEA, as a growth indicator, is highly positively correlated with meat production performance and lean meat percentage and is an important indicator for evaluating the carcass quality of domestic animals. The larger area of the loin eye and the potential higher fat content in the LT may positively influence the taste of the meat. It is noteworthy that the results of this study confirm the higher IMF content in the LT of Sunit sheep in the PRO, supporting the aforementioned interpretation. Similar observations have been made by Nie et al. and Ramzi et al., who reported that adding probiotics to the diet can increase IMF levels in mutton and alter the overall fat distribution in sheep, which is in line with our research results (Amran et al., 2021; Nie et al., 2022). The most plausible explanation for these findings is that probiotics modulate rumen fermentation patterns and alter indicators of lipid metabolism, including the composition of fatty acids, further promoting the synthesis and distribution of IMF. Therefore, it was necessary to explore the differences in the plasma biochemical indicators of sheep influenced by probiotics.
The results of this study on the effects of probiotics feed on plasma lipid biochemical indicators in Sunit sheep are consistent with the findings of Abas, Chiofalo, and Baiomy, who reported that probiotics supplementation can decrease the concentrations of plasma TG and LDL in lambs (Abas et al., 2007; Baiomy, 2010; Chiofalo et al., 2004). The reduction of plasma TG may be attributed to the improved digestive and absorptive functions of ruminant animals by probiotics feed, which can reduce the absorption and synthesis of fats in the intestine, thereby lowering the synthesis and accumulation of plasma TG (Sheikh et al., 2019). The current research findings on the impact of probiotics on cholesterol metabolism in sheep plasma are inconclusive. The research conducted by Mahesh and Jia suggests that probiotics can influence cholesterol metabolism through various metabolic pathways, such as reducing cholesterol absorption and inhibiting the activity of cholesterol synthesis enzymes (Jia et al., 2022; Mahesh et al., 2020). This leads to a decrease in the levels of LDL in the plasma, which has a positive effect on plasma cholesterol metabolism. In this study, the addition of probiotics didn’t significantly alter the levels of TC in the serum of Sunit sheep, but it did reduce the concentration of LDL in the plasma. This suggests that different types of probiotics may have varying effects on cholesterol metabolism in sheep. Therefore, more research is needed to elucidate the specific mechanisms by which probiotics regulate cholesterol lipoprotein levels in sheep plasma. In this study, the PRO showed a reduction in the levels of FFA in the plasma of Sunit sheep. Probiotics were able to decrease the rate of release of FFA, regulate fatty acid metabolism in plasma, and ultimately affect fat metabolism in muscle tissue. The decrease in FFA levels in plasma may lead to a reduction in fatty acid oxidation in muscle tissue. Fatty acid oxidation is the process by which fatty acids are converted into energy within cells. When fatty acid oxidation is reduced, muscle tissue can tend to store fatty acids as fat (Domaradzki et al., 2019; Roque-Jiménez et al., 2021). This could be a possible reason for the increase in IMF content in the muscle of feed with probiotics, as observed by Mackey, who found that supplementation of probiotics components to cattle in feed resulted in an increased marbling score in LT, indicating that probiotics may enhance IMF deposition in cattle (Mackey et al., 2023).
In meat, IMF is abundant in fatty acids, which can be absorbed by the human body and enter the bloodstream for transportation to various tissues. Changes in IMF content and TG levels in meat lead to corresponding alterations in the relative content of fatty acids. Increasing the IMF content is associated with a decrease in the concentration of PUFAs in meat, while the concentration of SFAs and MUFAs increases (Lukic et al., 2019; Purchas et al., 2005; Razmaitė et al., 2020; Realini et al., 2021). Joo suggests that the C18:1n9 cis levels in beef increase with the marbling pattern, and this elevation of C18:1n9 cis content is beneficial to the human body (Joo et al., 2017). Kazala indicates that the relative content of C14:0 in beef cattle increases with the increase in total lipids and TG. Meanwhile, the relative content of C18:3 in beef is negatively correlated with the total lipid content (Kazala et al., 1999). In this study, the model of PLS-DA was constructed. PC1 was defined as describing UFAs, while PC2 was defined as being able to simultaneously describe both SFAs and UFAs. The variable loadings plot showed that the fatty acids that contribute significantly to PC1 and PC2 were positively correlated in their relative positions in the two-dimensional space, mainly concentrated along the positive axes of PC1 and PC2.
As stated above, the addition of probiotics to the feed has been found to increase the IMF content in Sunit sheep. Furthermore, it has been observed that the use of probiotic feed has led to an increasing trend in the relative proportion of UFAs in the LT of Sunit sheep. Specifically, the proportions of C14:1 and C18:1n9 cis were significantly increased in the PRO. Among them, C18:1n9 cis is the most abundant UFA, which is considered to be positively correlated with the tenderness of fat and the sensory quality of meat. The higher C18:1n9 cis content can enhance the palatability of the meat (Tang et al., 2021). Furthermore, probiotics can modulate the synthesis and oxidation of MUFAs by regulating metabolic enzymes such as fatty acyl-CoA synthetase and enzymes limiting the rate of fatty acid oxidation, thereby altering the composition of UFAs in meat (Gheorghe et al., 2022). In accordance with the findings mentioned earlier, an increase in the relative proportion of MUFAs may result in a decrease in LDL levels in the body. In this study, the relative proportions of C18:3n6, C18:3n3, C20:3n6, C20:4n6, C20:5n3, C24:1n-3, and n-3 PUFA were found to be higher in the LT of Sunit sheep in the PRO. Among them, C18:3n6 and C18:3n3 are important substrates for the synthesis of long-chain PUFAs of n6 and n3 origin (Ganesh and Hettiarachchy, 2016). C20:4, as an important essential fatty acid, plays a role in changing cell membrane stability and participating in cell-mediated lipid metabolism (Calder, 2008). The content of C20:4 is related to the activity of fatty acid desaturase. The addition of probiotics to the diet benefits the activity of fatty acid desaturase. Generally speaking, higher expression levels of fatty acid desaturase are associated with a higher synthesis rate and content of C20:4 (Dev et al., 2021). The composition and relative proportion of fatty acids in meat are key factors that influence its nutritional value. This study suggests that the addition of probiotics to the diet improves the proportion of PUFAs in the LT of Sunit sheep, indicating that probiotics may have a role in improving the nutritional value of fatty acids in meat.
In order to further elucidate the mechanisms underlying IMF accumulation and fatty acid metabolism, we conducted a comprehensive analysis and assessment of gene expression levels and corresponding enzyme activities involved in the regulation of muscle fat deposition and fatty acid metabolic pathways. As widely acknowledged, the signaling of AMPK serves as a critical sensor of cellular energy, capable of participating in and regulating lipid homeostasis and fatty acid metabolism in muscle, including β-oxidation of fatty acid, synthesis of de novo fatty acid, and transport of fatty acid (Kim et al., 2014; Wang et al., 2013). According to research, AMPK is believed to play a crucial role in the regulation of lipid metabolism by modulating downstream enzymes, ACCα and CPT1B, which are involved in the fatty acid β-oxidation pathways (Ronnett et al., 2006). ACCα, as a central regulator of fatty acid metabolism, catalyzes the production of malonyl-CoA, an inhibitor of CPT1B, thus modulating CPT1B activity and regulating β-oxidation of fatty acid, therefore, the AMPKα2-ACCα-CPT1B signaling pathway plays a crucial role in fat deposition and fatty acid metabolism (Kim et al., 2012). The results of this study revealed that supplementation with probiotic feed inhibited the expression of AMPKα2 mRNA and CPT1 mRNA, while promoting ACCα mRNA expression in the LT of Sunit sheep. This suggests that probiotics feed may inhibit the expression of AMPKα2 signaling factor in Sunit sheep, impeding the AMPKα2-ACCα pathway, inhibiting CPT1B mRNA, and weakening the β-oxidation process of fatty acids, leading to increased fatty acid synthesis. The precise mechanism underlying the modulation of CPT1B mRNA by probiotics and its ultimate regulation of fat deposition in Sunit sheep remains unclear. Some studies have proposed that lactate generated as a result of probiotic administration can promote the generation of acetyl-CoA, which directly inhibits the expression of CPT1B mRNA. Subsequent reactions in this pathway ultimately lead to intracellular lipid accumulation (Araújo et al., 2020; Brooks, 2020).
In addition, the transcription factor SREBP-1c is a key regulatory factor in the synthesis of fatty acids, capable of activating the expression of FASN mRNA, ACCα mRNA, and SCD1 mRNA, while its expression is also controlled by AMPK. Consistent with ACCα, the activity of SREBP-1c is negatively regulated by AMPK. Research has shown that AMPK inhibits the expression of SREBP-1c mRNA, thereby reducing the expression of FASN mRNA and subsequently inhibiting lipogenesis (An et al., 2019; Deng et al., 2015). SCD1, a crucial factor expressed in adipose tissue, is a target gene for SREBP-1c and plays a selective role in the regulation of de novo lipogenesis and fatty acid conversion, mediating adipogenesis and fatty acid metabolism. Deficiency of SCD in sheep is associated with decreased TG levels, as well as reduced expression of SREBP-1c mRNA and FASN mRNA (He et al., 2021; Liu et al., 2020; Tian et al., 2018). In this study, probiotics were found to inhibit the expression of AMPKα2 mRNA while promoting the expression of SREBP-1c mRNA and FASN mRNA, which may result in a tendency to fat synthesis in LT, leading to IMF deposition. Furthermore, probiotics significantly increased SCD mRNA expression, indicating that probiotics have the ability to gradually convert SFAs to UFAs, which may be one of the reasons why the probiotic group had a relatively higher proportion of UFAs as mentioned earlier (Tian et al., 2021).
Further research unveiled a similarity between the enzyme activity outcomes and the mRNA expression level findings. The results indicate that probiotics feed can regulate the ACCα, CPT1B, SREBP-1c, FASN, and SCD1 by inhibiting the AMPKα2, resulting in enhanced IMF synthesis and reduced oxidation of fatty acids in the LT of Sunit sheep. This ultimately leads to an increase in IMF content and improved fatty acid composition in meat.
The correlation analysis between gene expression and IMF, TG, and fatty acid composition has been used to investigate the relationship between gene signaling pathways and lipid composition parameters. Even minor changes in chemical structure can significantly alter lipid signaling activity, so research on gene-regulated fatty acids composition is limited (Tánori-Lozano et al., 2022). The AMPK signaling factor can regulate the cellular uptake of fatty acids through translocation of fatty acids. Studies suggest that inhibiting AMPKα2 may increase key enzyme activities in the fatty acid synthesis pathway, leading to an increase in PUFAs synthesis, while simultaneously reducing cellular fatty acid β-oxidation, resulting in the accumulation of fatty acids (Dzamko et al., 2008; Kohan et al., 2009). In addition, downstream genes of the AMPK signaling pathway, including ACCα, CPT1B, SREBP-1c, FASN, and SCD1, are closely associated with the composition of fatty acids. Studies suggest that elevated expression of ACCα mRNA promotes the deposition of UFAs, and the accumulation of UFAs inhibits the expression of CPT1B mRNA (Zhu et al., 2022). Meanwhile, the main function of SREBP-1c is to promote the synthesis of fatty acids and TG, exerting a positive regulatory effect on adipose deposition and fatty acid metabolism (Guo et al., 2023). In this study, the expression levels of AMPKα2 mRNA and CPT1B mRNA were negatively correlated with IMF, C18:3n6, C20:3n6, and C20:4n6, while the expression levels of ACCα mRNA were positively correlated with their contents. Furthermore, it was further confirmed that inhibition of the AMPKα2 signaling pathway could promote IMF deposition in Sunit sheep by enhancing ACCα and SREBP-1c factors and improving the composition of fatty acids (Fig. 5).
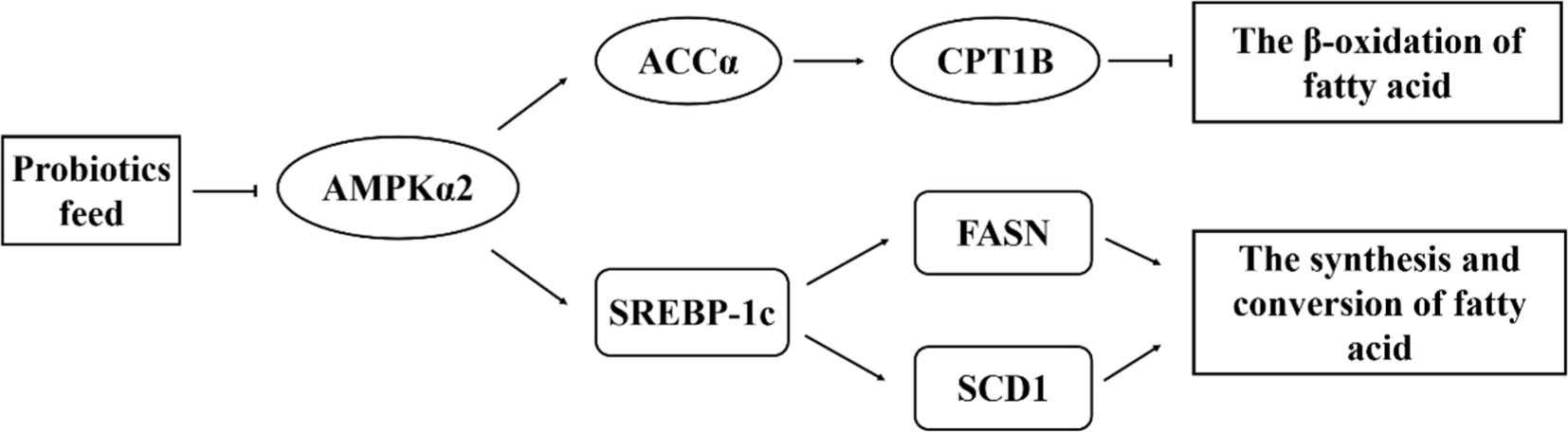
Conclusion
In conclusion, the results of this study indicate that the addition of probiotics to the diet can regulate fat deposition and improve fatty acid composition in the LT of Sunit sheep through the signaling pathways AMPK-ACC-CPT1 and AMPK-SREBP-1c. This regulatory mechanism leads to an increase in the LEA in Sunit sheep, improved growth performance, and altered plasma lipid biochemical indicators. The findings of this experiment highlight the regulatory effects of probiotics added to the diet on fat deposition and fatty acid composition in the LT of Sunit sheep and provide new insights into the nutritional interactions of ruminant animals mediated by the AMPK pathway. The research results contribute to a better understanding of the potential application of probiotics and provide new insights into the future of food science and animal resources.