Introduction
A phase transition of liquid water to solid ice is a spontaneous phenomenon at subzero temperature. Nevertheless, water is often supercooled to low subzero temperature unless ice nucleation is occurred. Ice nucleation is a complex phenomenon which is yet completely understood, but it is an important factor affecting freezing behavior of meat. Up to date freezing techniques such as a pressure-shift freezing, an electromagnetic freezing or an ultrasound wave freezing are based on arbitrary control of ice nucleation (Espinosa et al., 2016; Zhan et al., 2018; Zheng and Sun, 2006). Nevertheless, stochasticity of ice nucleation has been regarded as the main problem in developing an advanced freezing technology (Niedermeier et al., 2011).
In general, ice nuclei cannot grow up to crystals unless the nuclei are larger than critical size. From the thermodynamic point of view, the critical size of ice nuclei is proportional to ice-water surface tension, freezing point, amount of latent heat of fusion, while inversely to ice nucleation temperature (Pereyra et al., 2011). Theoretically, ice nuclei formed in meat has lower critical size than those in pure water which indicates that ice nucleation of meat is occurred at near below freezing point of meat. Therefore, supercooling phenomenon was rarely observed during meat freezing, and our previous study revealed that artificially lowering ice nucleation temperature had potential advantages of not only reducing freezing time but also minimizing tissue damage of pork loin (Kim and Hong, 2016).
Although cryoprotectants can inhibit either ice nucleation or ice crystallization (Boonsupthip and Lee, 2003), usage of these chemicals is limited in unprocessed meat. Since water in meat is held within meat structure (myofibrillar systems and connective tissue), ice nucleation is related with interactions between water and proteins, and hydrophobicity of meat proteins can influence on the ice nucleation phenomenon (Lupi et al., 2014; Zachariassen and Kristiansen, 2000). However, it has never investigated how chemical state of proteins affect the ice nucleation phenomenon. Typically, thermal process is the representative unit process which affects the protein structure, while compositional changes caused by drip generation can act as an additional factor to interpret ice nucleation phenomenon in meat. In this case, high pressure process may be the best tool to modify chemical state of meat proteins without compositional changes (Rastogi et al., 2007). Consequently, this study explored the relationship between pressure-mediated structural changes of proteins and ice nucleation phenomenon of pork loin.
Materials and Methods
Pork loin (Musculus longissimus dorsi) at 48 h post-mortem was purchased from a local market. Visible fat was completely trimmed off, and pork loins were vacuum packaged with poly-nylon pouch. Pork meat was pressurized using a commercial device (IWPM-600MPa-50L, Innoway, Seoul, Korea) from 100 to 500 MPa for 3 min at ambient temperature. Pressure built up and depression rates were 100 and 150 MPa/min, respectively. After treatment, all pork loins were kept at 2°C refrigerator prior to analysis.
Three slices with 20 mm thickness was obtained from middle part of each pork loin for the analyses of moisture content and expressible moisture (EM). From the center of each slices, approximately 1.5 g samples for moisture content and EM analyses were taken and weighed. Moisture content of each treatment was conducted by 105°C hot-air drying method. EM of sample was measured by Boles and Shand (2001) with slight modification. Meat sample was placed on the centrifuge tube with gauze as a moisture absorber and centrifuged at 1,500×g for 10 min under refrigeration mode (4°C). After centrifugation, meat pellet was removed, and tube was weighed. The tube was dried at 105°C for 24 h and weighed again. The EM of treatment was expressed as percent moisture loss from meat weight.
DSC was adopted to measure pressure-induced structural changes and ice nucleation phenomenon of pork loin. Approximately 20 mg meat was sampled from the inside of pork loin and moved to an aluminum pan. The pan was tightly sealed and placed into a differential scanning calorimeter (DSC200F3, Netsch Geratebau GmbH, Selb, Germany) calibrated with an indium. Thermal scan of the pan was conducted under the condition of equilibrium at 20°C for 4 min thereafter heating to 95°C by 5°C/min. For observation of ice nucleation, meat sampling procedure was conducted as the same way described above. Program setting condition was in the order of equilibrium at 20°C for 4 min and cooling to −20°C by 1°C/min. All tests were determined in triplicate, and an empty pan was used as a reference. Data obtained from each experiment was analyzed using a Proteus analysis software (Netsch Gerätebau GmbH).
From each experiment, triplicated determination was conducted, and the whole experiment was repeated three times on three different days using new batches (n=9). Data were analyzed using R software (ver. 3.6.1, The R Foundation, Vienna, Austria). One-way analysis of variance (ANOVA) was performed to evaluate the effect of pressure level (p<0.05), and Duncan's multiple range test was conducted as a post-hoc procedure.
Results and Discussion
There was no difference in moisture content among treatments with different pressure level, and all treatments contained 72.0%–72.9% of identical moisture content (Fig. 1). Meanwhile, pressure level affected the EM of pork loin. Pork treated at moderate pressure level (100–200 MPa) showed 6.83%–7.54% of lower EM comparing to 9.82% of control (p<0.05). Further increasing pressure level (>200 MPa) caused a gradual increase in EM, and pork pressurized at greater than 400 MPa had 16.05%–17.41% of lower EM than that of control (p<0.05). The former decrease in EM of pork could be explained by structural changes of meat protein under high pressure processing below 200 MPa (Hong et al., 2012). It was reported that proteins underwent reversible unfolding as known to molten globule state under moderate pressurization. Upon depression, unfolded protein was refolded but not recover to its origin which accounted for the improve the water-binding properties of proteins (Boonyaratanakornkit et al., 2002). Alternately, protein unfolding was irreversible when applied pressure level is greater than 300 MPa, and the irreversible unfolding of meat proteins would attribute to high EM of pork loin (Boonyaratanakornkit et al., 2002; Knorr et al., 2006).
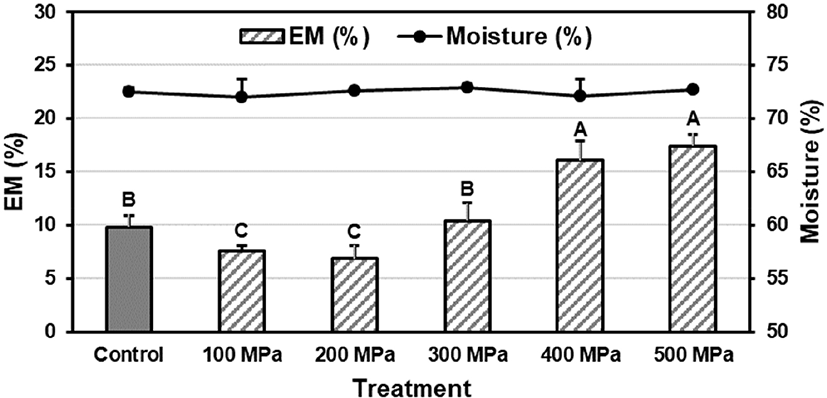
DSC thermal scan profiles revealed that fresh meat had three typical peaks of which maximum endothermic peak temperatures were 57.5°C, 63.8°C, and 80.0°C, respectively (Fig. 2). It was broadly known that the first and third peaks from DSC data were associated with myosin and actin, respectively, while the second peak was related with sarcoplasmic protein and/or connective tissue (Fernandez-Martin et al., 2000; Kazemi et al., 2011). Maximum first peak temperature slightly decreased to 56.3°C after 100 or 200 MPa pressurization, reflecting that myosin structure was affected even by moderate pressurization. The peak was disappeared in pork pressurized at greater than 300 MPa, reflecting that the complete unfolding of myosin structure was occurred at 300 MPa. Second peak showed irregular change in peak temperature depending on applied pressure level. The maximum peak temperature tended to increase with increasing pressure up to 200 MPa, thereafter decreasing with further increasing pressure level. Based on the report of Fernandez-Martin et al. (2000), maximum denaturation temperatures of sarcoplasmic protein and collagen were separated to 60°C and 67°C, respectively. In addition, collagen was stable comparing to other meat proteins against high pressure treatment (Cheftel and Culioli, 1997). Up to 200 MPa of pressurization, therefore, pressure-labile sarcoplasmic fractions would undergo unfolding without changing collagen structure, whereas the collagen structure was affected by pressurization greater than 300 MPa. The third peak was not affected by moderate pressurization (100–200 MPa), while it also completely denatured by pressurization at greater than 300 MPa.
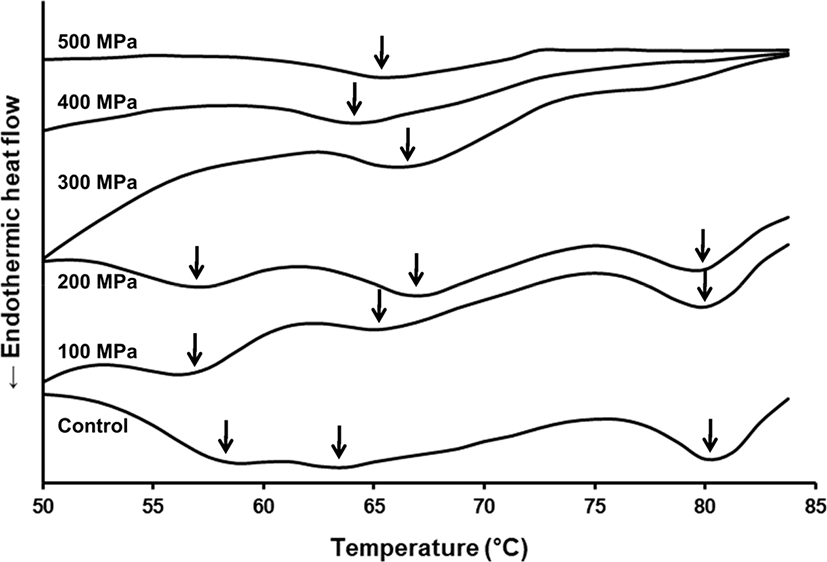
According to the results, pork loin had different water-binding properties without difference in composition (Fig. 1), and the pressure-mediated structural change of meat proteins would be the main factor influencing on the ice nucleation phenomenon (Fig. 3). Upon cooling to subzero temperature, exothermic enthalpy of all treatments did not differ from one another (mean 684 kJ/kg) which would be resulted from the identical moisture content of treatments. However, ice nucleation temperature was depended on applied pressure levels (p<0.05). Fresh control showed −7.61°C of reproducible ice nucleation temperature (±0.14°C) which was likely due to finely controlled experimental condition. Increasing pressure level up to 200 MPa seemed to decrease the ice nucleation temperature, however, the result was not significantly difference due to high standard deviation (±2.99°C) of 200 MPa treatment. Compared to control, significantly low ice nucleation temperature (−11.4°C) was observed in pork pressurized at greater than 300 MPa (p<0.05). As mentioned, moderate pressurization mediated structural changes of meat proteins provided improved protein-water interactions as shown by low EM, but the change was not uniform within sample matrix probably due to short holding time (3 min). Although, holding time is an important variable in moderate pressurization, the impact of holding time on the protein structure was lesser than that of pressure level (Lee and Hong, 2016). For that reason, reproducibility of ice nucleation was increased again with further increasing pressure level (>300 MPa). According to Okamoto and Suzuki (2002), meat pressurized at 300 MPa lost the integrity of sarcomere structure, hence the completely unfolded contractile systems would form a gel-like structure which retained moisture during pressurization with reducing protein-water interactions. It was supposed that capillary force and surface tension were enhanced by entrapping water molecules within gel matrix, and the force fields would suppress the structuring of ice nuclei (Hruby et al., 2014). Another important feature of ice nucleation phenomenon was the pattern of heat removal from meat. The exothermic heat of sample with ice nucleation temperature higher than −10°C was low at the beginning of ice nucleation, and the heat is gradually and slowly removed during cooling. Contrarily, initial release of heat was steep in sample with lower ice nucleation temperature than −10°C, thereby showing rapid heat removal during cooling. Since the rapid heat removal indicated rapid phase transition (Kim and Hong, 2016), eventually the result indicated that freezing rate of pork meat was proportional to applied pressure levels. Until now, combined effect of high pressure and freezing of meat was focused on pressure-shift freezing, hence there was no comparable study which estimated quality characteristics of meat pressurized followed by freezing. This study would be first trial to provide information regarding the effect of high pressure on the overall freezing characteristics of meat.
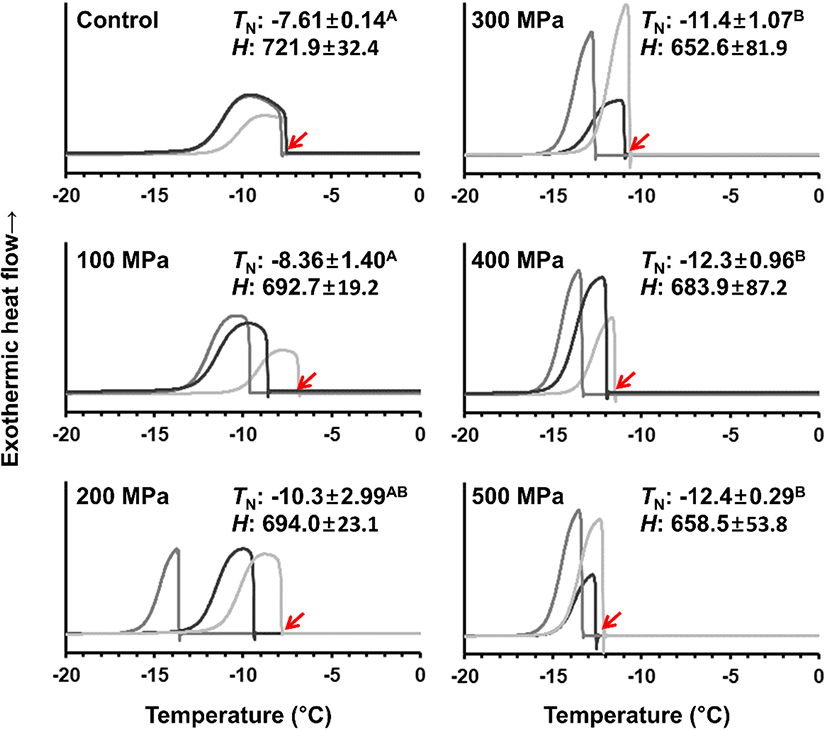
Conclusion
This study demonstrated that ice nucleation phenomenon could be affected by chemical state of proteins, and pressure-mediated structural change (unfolding) of meat proteins suppressed ice nucleation, thereby lowering the ice nucleation temperature of pork loin. However, this study could not explain the actual mechanisms involving how unfolding of proteins suppressed the ice nucleation, findings of this study could suggest useful strategies to produce better quality frozen meat. Eventually, further explorations regarding either the holding time at moderate pressurization or quality comparison after freezing of fresh and pressurized meat were warranted.