Introduction
Memory is a cognitive process that can be studied throughout an individual’s life span, and cognitive skills are continually used to adapt to an ever changing environment (Sharma, 2009). With age, reduction in cognitive function is one of the changes from loss of neuronal function. Cholinergic neurons in the brain are associated with learning and memory, executive functioning, behavior, and emotional responses. Acetylcholine (ACh) is the principal neurotransmitter in the peripheral, central, somatic, and autonomic nervous systems. Loss of ACh results in cognitive dysfunction such as dementia or Alzheimer’s disease (AD) (Bierer et al., 1995). Acetylcholine esterase (AChE) catalyzes ACh, and many scientists have identified functional compounds, such as peptides/hydrolysates, flavonoids, and vitamin E, to inhibit AChE (Ali Reza et al., 2018; Chen et al., 2015; Kim et al., 2013; Pei et al., 2010; Pervin et al., 2014; Srividhya et al., 2012). The brain is especially vulnerable to oxidative stress because of its high oxygen utilization (Feng and Wang, 2012). Moreover, oxidative stress induces biological cell damages, such as oxidation of protein, lipid, DNA and glycooxidation, which are associated with AD (Ali Reza et al., 2018). Dietary antioxidants may play an important role in retarding several cognitive disorders associated with neuronal diseases, including dementia according to the experimental and clinical data (Meydani, 2001). For example, the neuronal and cognitive dysfunctions associated with aging have been shown to be retarded in animals fed dietary supplements of vitamin E or extracts of fruits and vegetables (Joseph et al., 1999), defatted walnut meal hydrolysates (Chen et al., 2015), egg white protein hydrolysates (Martinez et al., 2019), fish peptides (Pei et al., 2010) with high antioxidant activity and cholinergic system maintenance properties (Floyd and Carney, 1992). Thus, natural dietary products might retard the AD by concomitantly protecting brain cells from oxidative stress and acting as cholinesterase inhibitors (Costa et al., 2013; Pervin et al., 2014).
Collagen has attracted considerable attention as a bio-material for drug delivery and tissue engineering due to its low antigenicity (Li et al., 2004). Collagen can be transformed into gelatin, which is consumed as a food source, via heat treatment. Gelatin hydrolysates generated using edible enzymes are natural additives and approved by the Food and Drug Administration (FDA) (Dybka and Walczak, 2009). In fact, gelatin hydrolysates containing soluble peptides are manufactured using proteolytic enzymes via controlled hydrolysis. These hydrolysates have been considered to possess beneficial biological properties such as antioxidant (Chang et al., 2013) and bone growth enhancing abilities (Leem et al., 2013). The peptides in gelatin hydrolysates are readily absorbed by the blood from the gastrointestinal tract, thereby becoming available for metabolic processes, whereas gelatin is difficult to absorb (Zague, 2008). Previously, we have shown that pork skin gelatin hydrolysates generated using enzymatic hydrolysis with Flavourzyme®1000L possess antioxidant activity (Kim et al., 2013). Moreover, antioxidants have a significant potential of reducing the symptoms and incidence of AD (Ali Reza et al., 2018; Pervin et al., 2014), According to the study by Ali Reza (2018), potential antioxidants from plant sources showed a high correlation between AChE inhibition activity (r2=0.978) and DPPH radical scavenging activity (r2=0.998). However, studies on the supplementary effect of water-extracted gelatin hydrolysates on learning and memory function of mice are limited.
Therefore, the aim of this study was to elucidate the antioxidant activity and protective effect of water-extracted pig skin gelatin hydrolysates (low molecular weight) generated by food enzymes against scopolamine-induced damage of memory and cognitive function in mice.
Materials and Methods
Pig skin gelatin water extracts (PSW) and low molecular weight pig skin gelatin water extract (LPSW) were prepared by the procedure of Kim et al. (2013) with some modifications. Fat-trimmed pig dorsal skin was extracted thrice in hot water at 100°C for a total of 7 h : 2h (first extraction), 2 h (second extraction), and 3 h (third extraction). After every extraction, fresh water was added and all the extracts were mixed. The fat in the extracts was discarded using Folch’s solution [chloroform: methanol, 2:1(v/v)]. Then, the extract was lyophilized and used as PSW for analysis. For generating LPSW, the lyophilized PSW was swollen in eight-fold excess distilled water at 80°C and stirred for 1 h and adjusted to pH 7.0. Flavorzyme®1000L [0.3% (w/w); Novozymes, Bagsvaerd, Denmark] from Aspergillus oryzae was subsequently added and the mixture was incubated for 12 h at 50°C. Enzymatic hydrolysis was stopped by heating at 95°C for 10 min. The hydrolysates centrifuged at 4,000×g for 30 min using Amicon® Ultra-15 centrifugal filter units (Merck Millipore, Bedford, MA, USA) and the 3 kDa molecular weight filtrate was lyophilized and used as LPSW.
The oxygen radical absorbance capacity (ORAC) assay kit (Cell Biolabs, San Diego, CA, USA) was used to determine ORAC value according to manufacturer’s instructions. Results were presented as μM Trolox equivalent (TE).
Seventy male ICR mice weighing 20–25 g were randomly assigned to seven groups (Fig. 1): Control (CON); scopolamine (SCO, 1 mg/kg body weight (B.W.), intraperitoneally (i.p.); tetrahydroaminoacridine 10 [THA 10, tacrine; 10 mg/kg B.W. per oral (p.o.) with SCO (i.p.)]; pig skin water extracts [PSW 10, 10 mg/kg B.W. (p.o.) with SCO (i.p.)]; PSW 40 [40 mg/kg B.W. (p.o.) with SCO (i.p.)]; low molecular weight pig skin water extracts [LPSW 100, 100 mg/kg B.W. (p.o.) with SCO (i.p.)]; LPSW 400 [400 mg/kg B.W. (p.o.) with SCO (i.p.)]. Each group consisted of ten mice, which were housed in wire cages and maintained on a 12 h day/night cycle with free access to food and water at constant temperature (23°C±1) and humidity (50%–60%) for 15 weeks. All measurements were made between 10:00 and 18:00 h. Scopolamine hydrobromide (Sigma-Aldrich, Gillingham, UK), a well-known muscarinic receptor blocker that impair learning and memory functions in both animals and human beings, was dissolved in 0.9% sterilized saline at a dose of 1.0 mg/kg. The dissolved scopolamine (0.2 mL) was then injected intraperitoneally 30 min prior to the experiment. Tacrine, the drug for cure of AD, was used as a positive control to compare the enhancing effect of PSW and LPSW. All animal experiments were performed under Kangwon National University’s Committee on the Care and Use of Laboratory Animals Guidelines (KIACUC-12-0011) and analytical grade of chemicals and reagents were used.
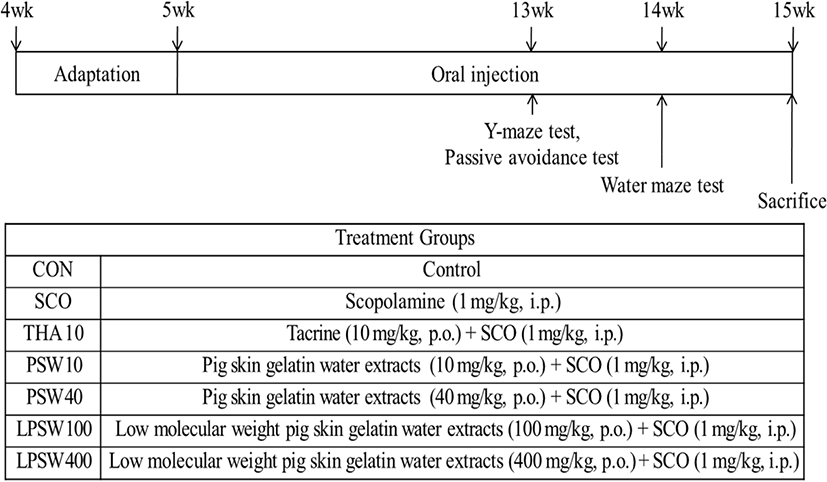
The Y-maze test is used to determine short-term memory (immediate spatial working memory) (Rao et al., 2005). Spatial memory contributes to an animal’s knowledge and exploration of the available resources in its surroundings (Sharma, 2009). The Y-maze comprises of a three-arm horizontal maze (40 cm long and 3 cm wide with 15 cm-high walls) in which the arms, labeled A, B, and C, are symmetrically disposed at 120° to each other. The number and sequence of arm entries made during each 8-min session were recorded. Alternations were regarded as an entry into each arm within three consecutive arm choices such as A-B-C or B-C-A. Percentage of alternation was calculated as the number of alternations divided by the number of total arm entries minus two, as calculated using the equation as follows. The number of arm entries was considered as the indicator of locomotor activity.
The passive avoidance test was performed by the method of Das et al. (2005). This test was conducted by a shuttle box [(410 (w) × 201 (D) × 300 mm (H)] comprised of two compartments: an illuminated place with a 60 W bulb and a dark compartment consisting of 2-mm stainless steel rods with 1 cm apart. A guillotine door was used to isolate the compartments. One hour after the last administration of the test materials, for the acquisition trial, each mouse was in turn gently placed in the illuminated place and the door was opened after 10 s. When mice entered the dark place, the door was manually closed and an electrical shock (0.5 mA) of 3 s duration was delivered through the stainless steel rods. The time taken for the mouse in the dark place was recorded as the initial latency time. Twenty-four hours after this acquisition trial, the mouse was again placed in the illuminated place for a retention trial. The time taken for the mouse to enter the dark place after the door was opened was regarded as the retention latency time for both trials. The retention latency time to enter the dark place was listed up to 180 s. If a mouse did not enter the dark place within 180 s, it was regarded as a retention latency time score of 180 s.
The Morris water maze is a useful behavioral test for assessing spatial learning ability associated with septohippocampal cholinergic activity (Li et al., 2001). It was conducted in a pool of 107 cm diameter with a circular acrylic platform (10 cm in diameter) submerged 1 cm below the surface of the opaque water at 23±2°C. Mice were allowed two acquisition trials per day for four days. Movement of mice in the water maze was captured using a camera and evaluated manually using a clock timer during each trial. The mice were allowed to stay for 10 s on to the platform, when they found hidden platform beneath the opaque water. When the mice failed to find the platform within 120 s, they were placed on the platform by the experimenter for a maximum of 30 s. A day after the last training trial session, the mice were subjected to the pool in which the platform was removed and the animals were allowed to swim for 120 s searching for it. The swimming time in the pool quadrant where the platform had previously been placed was kept recording.
After completion of the behavior test, mice were anesthetized using diethyl ether and blood was taken by heart puncture. The blood was centrifuged at 890×g for 15 min, and the serum total protein, glutamic oxaloacetic transaminase (GOT), and glutamic pyruvic transaminase (GPT) levels were determined using the ADVIA 2400 chemistry system (Siemens, Malvern, PA, USA). The brain, liver, lungs, kidneys, spleen, and testes were dissected, weighed, and expressed as relative organ weight (with respect to body weight).
Brain homogenates were prepared using the method of Kim et al. (2010). Whole brain tissue (n=5) was homogenized in 12.5 mM sodium phosphate (pH 7.0) buffer containing 400 mM NaCl using a Teflon tissue grinder at 4°C. The whole brain homogenate was used for determination of the ACh content, AChE activity, and monoamine oxidase-B (MAO-B) activity.
Homogenate of the brain tissue was centrifuged at 10,000×g for 20 min at 4°C. The supernatant was used to determine ACh content using the EnzyChrom ACh assay kit (EACL-100, Bioassay System, Hayward, CA, USA).
AChE activity was determined using the method of Ellman et al. (1961) with slight modifications. The brain tissue homogenate was centrifuged at 1,000×g for 10 min at 4°C. For the reaction, 260 μL of 100 mM sodium phosphate buffer (pH 8.0), 20 μL of 10 mM 5-5'-thiobis-2-nitrobenzoic acid (DTNB), 10 μL of brain tissue supernatant, and 10 μL of 100 mM ACh chloride were added. ACh (10 μL, 100 mM) was added before starting the reaction and the absorbance was subsequently detected at 412 nm using a UV/visible microplate reader (Spectra Max M2e, Molecular Devices, Sunnyvale, CA, USA). The reading was repeated at 15 s intervals to verify that the reaction occurred lineally.
After centrifugation (10,000×g for 20 min at 4°C) of the brain tissue homogenate, the pellet was used to assay MAO-B activity in brain tissue using the Amplex Red monoamine oxidase assay kit (A-12214, Molecular Probes, Eugene, OR, USA). In brief, a reaction mixture (500 μL) containing Amplex Red reagent (400 μM), benzylamine (2 mM) as a specific substrate for MAO-B, and horseradish peroxidase (2 U/mL) was prepared. The mixtures were incubated at 23°C in 96-well plates for 1 h. Fluorescence was then measured using a fluorescence reader (Molecular Device, USA) at excitation wavelength of 560 nm and emission wavelength of 590 nm. The MAO-B activity was recorded as μM resorufin/60 min/mg protein. The protein content of the sample was assayed using the bicinchoninic acid (BCA) protein assay kit (Sigma-Aldrich, St. Louis, MO, USA).
Results and Discussion
The yields and protein content of PSW and LPSW were 27.3% and 11.6%, and 525.7±10.73 and 58.9±0.51 mg/g dry mass, respectively (data not shown). The ORAC values of PSW and LPSW increased in a dose dependent manner (Fig. 2). The ORAC value of PSW and LPSW at 1 mg/mL was 40.14 μM TE and 154.14 μM TE, respectively. The low molecular weight hydrolysates of <3 kDa showed significantly higher ORAC value. This is in accordance with the results of Kim et al. (2013), who reported that the ORAC values of 1 mg/mL pig skin gelatin hydrolysates (more than 50 kDa) and low molecular weight pig skin gelatin hydrolysates (less than 3 kDa) were 29.31 μM TE and 141.39 μM TE, respectively. Joseph et al. (1998) reported that supplementation of AIN93 diet with spinach extracts containing high antioxidant activity, assessed by the ORAC assay, prevented the onset of age related deficits in several indices (signal transduction) and cognitive behavior (Morris water maze performance).
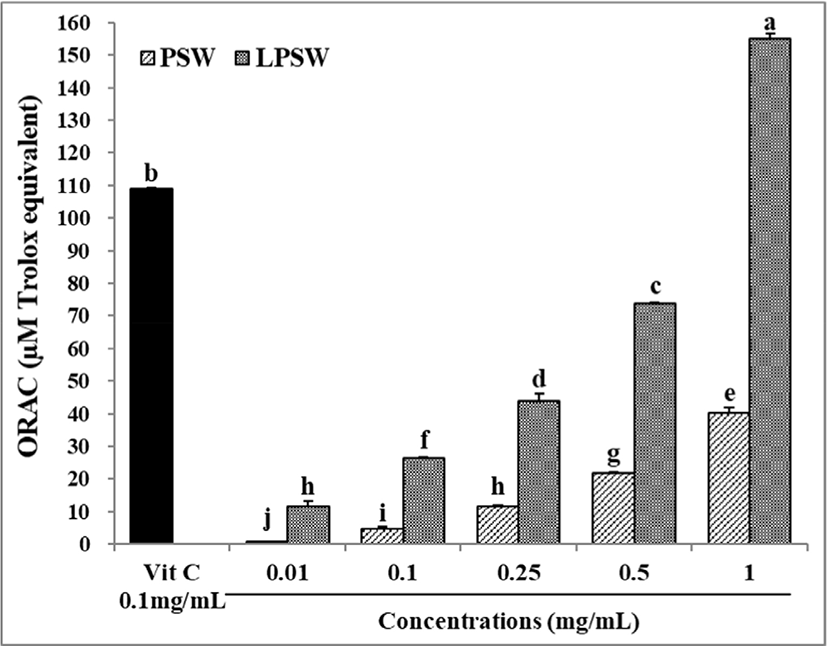
Body weight gain, feed intake, and feed efficiency of mice are shown in Table 1. The results suggested that PSW and LPSW supplementation did not affect the physical condition of mice during the experiments.
1) Control (CON); scopolamine (SCO, 1 mg/kg body weight (B.W.), intraperitoneally (i.p.); tetrahydroaminoacridine 10 [THA 10, tacrine; 10 mg/kg B.W. per oral (p.o.) with SCO (i.p.)]; pig skin water extracts [PSW 10, 10 mg/kg B.W. (p.o.) with SCO (i.p.)]; PSW 40 [40 mg/kg B.W. (p.o.) with SCO (i.p.)]; low molecular weight pig skin water extracts [LPSW 100, 100 mg/kg B.W. (p.o.) with SCO (i.p.)]; LPSW 400 [400 mg/kg B.W. (p.o.) with SCO (i.p.)].
Y-maze spontaneous alternation is a behavioral test for determining the willingness of rodents to explore new environments (Ru and Liu, 2018). Mice prefer to investigate a new arm of the maze rather than returning to an arm that has been visited previously. Many parts of the brain, including the hippocampus, septum, basal forebrain, and prefrontal cortex, are involved in this task (Sharma, 2009). The number of total entries (A) and the spontaneous alternation ratio (B) of mice fed PSW and LPSW are shown in Fig. 3. The spontaneous alternation ratio of mice in the LPSW 400 group was significantly higher than that of the SCO group and was similar to the ratio of the CON and THA groups (p<0.05). This suggests that LPSW 400 attenuated the decline in scopolamine-induced spatial working memory of mice. In our previous study, administration of 2% gelatin hydrolysates (molecular weight 3–50 kDa) isolated from pig skin gelatin after 12 h hydrolysis using a 1:1 mixture of Alcalase and Protamex significantly increased alternation behavior by up to 13.5% (Jang et al., 2011).
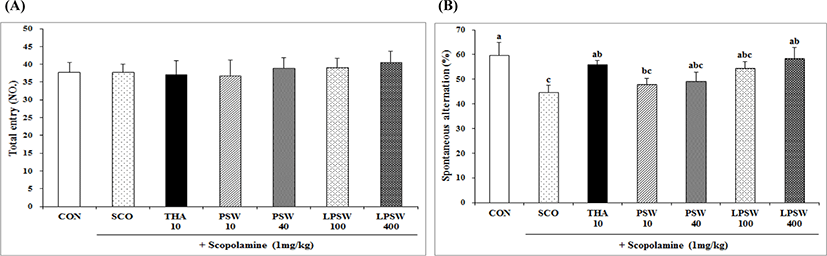
The latency time of mice supplemented with PSW and LPSW in terms of passive avoidance test training and test trial is shown in Fig. 4. During the training, no significant difference was observed; however, the latency time required to resist the black compartment was significantly higher in the LPSW 400 group than in the SCO, PSW 40, and LPSW 100 groups. This demonstrated that dietary administration of LPSW 400 may facilitate the acquisition of spatial leaning and increase passive avoidance ability, increasing these to the level observed for THA, although these values did not reach up to the level of the CON group. Pei et al. (2010) reported that supplementation with 0.22%, 0.44%, and 1.32% (w/w) marine collagen peptide (MCP) for 3 months significantly enhanced the learning ability of aged mice. They also reported that the MCP significantly alleviated oxidative stress, reduced the number of apoptotic neurons, and up-regulated the expression of brain-derived neurotrophic factor.
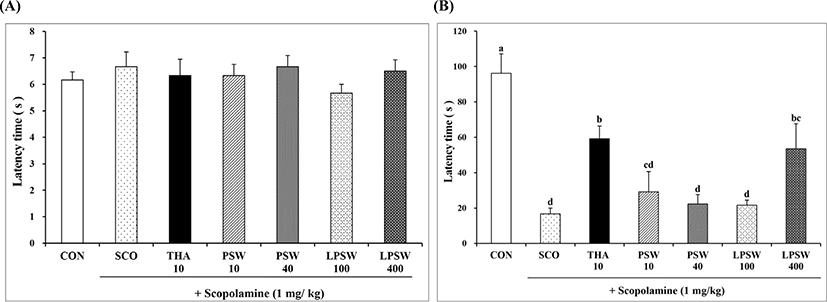
The water maze task is a common and sensitive behavioral test used for assessing the spatial learning and memory of experimental animals (Li et al., 2001). To complete the task, animals must locate a hidden platform during a series of trials. Once animals learn where the hidden platform is located, they can remember this location and swim rapidly to it from any starting point. The time taken to reach the platform was measured. As shown in Fig. 5A, the mean latency time to find the platform decreased progressively during the four training days in all animals. Mice in the control group showed a significant decrease in latency time during the acquisition trial (p<0.05). On the fourth day after training for 3 days, mice in the SCO group showed significantly longer latency time (79.83 s); however, mice in both the LPSW 100 (53.17 s) and LPSW 400 (24.17 s) groups showed significantly shorter latency time than those in the SCO group. The latency time of the LPSW 400 group was significantly shorter than that of the LPSW 100 group, which were similar to those of CON (13.83 s) or THA (31.37 s) groups, respectively. This suggested that LPSW 400 significantly enhanced Morris water maze performance against learning and memory impairment induced by scopolamine, and was as effective as THA. On the day of the test trial, mice swam in the vicinity of the place where the platform had been located during the acquisition trial. As shown in Fig. 5B, mice in the LPSW 400 group showed higher search precision for the platform than mice in the SCO, PSW 40, and LPSW 100 groups did (p<0.05), which was comparable to those of mice of the CON and THA groups. Therefore, supplementation of LPSW at 400 mg/kg appeared to restore the scopolamine-induced loss of spatial memory in mice.
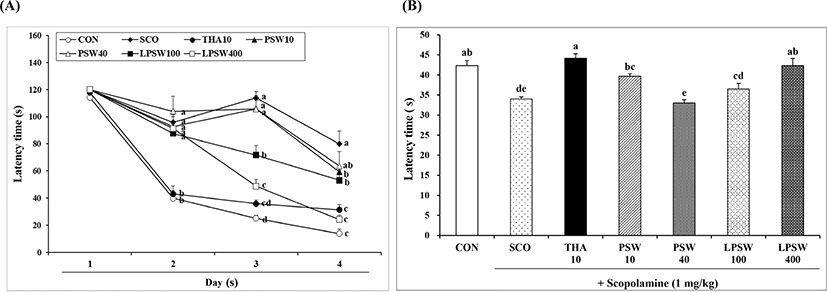
Scopolamine is used as an amnesia inducer due to its adverse effects on cognitive function such as vomiting, nausea, weight loss, and hepatotoxicity (Mendiola-Precoma et al., 2016). Tacrine, used as a positive control in this study, was the first drug approved for the treatment of AD since 1993. However, it was withdrawn in 2013 because of its hepatotoxicity (de los Ríos and Marco-Contelles, 2019). To assess hepatotoxicity in mice injected with tacrine and scopolamine, and that after supplementation with PSW and LPSW, the serum total protein, and GOT and GPT levels of mice were estimated (Table 2). The serum total protein content of mice in the CON and SCO groups did not differ. However, mice in the THA 10 group showed significantly higher total protein content due to hepatotoxicity. Neither concentration of PSW and LPSW induced any significant difference in serum protein content. In addition, LPSW 400 treatment did not significantly alter the serum protein contents of the CON and SCO groups, indicating that administration of LPSW 400 mg/kg did not change serum protein content. The serum GOT level of mice dosed with THA and PSW 10 were significantly higher than those of control animals and those receiving LPSW400. Furthermore, LPSW 100 and 400 significantly reduced the GOT level up to that observed in the control group, whereas no significant difference was observed when compared to the level in the SCO group. Serum GPT level was not affected by scopolamine (i.p.), tacrine (p.o.), PSW (p.o.), and LPSW (p.o.) treatments.
1) Control (CON); scopolamine (SCO, 1 mg/kg body weight (B.W.), intraperitoneally (i.p.); tetrahydroaminoacridine 10 [THA 10, tacrine; 10 mg/kg B.W. per oral (p.o.) with SCO (i.p.)]; pig skin water extracts [PSW 10, 10 mg/kg B.W. (p.o.) with SCO (i.p.)]; PSW 40 [40 mg/kg B.W. (p.o.) with SCO (i.p.)]; low molecular weight pig skin water extracts [LPSW 100, 100 mg/kg B.W. (p.o.) with SCO (i.p.)]; LPSW 400 [400 mg/kg B.W. (p.o.) with SCO (i.p.)].
The relative weights of the liver, kidneys, spleen, lungs, testes, and brain of the test animals are shown in Table 3. There was no significant difference in relative weight among the treatment groups. Organ weight can be the most sensitive indicator of the effect of an experimental compound, as significant differences in organ weight between treated and untreated (control) animals may occur in the absence of any morphological changes (Bailey et al., 2004). Our results indicated that supplementation of PSW and LPSW at 100 and 400 mg/mL concentration did not change the organ weight in mice.
1) Control (CON); scopolamine (SCO, 1 mg/kg body weight (B.W.), intraperitoneally (i.p.); tetrahydroaminoacridine 10 [THA 10, tacrine; 10 mg/kg B.W. per oral (p.o.) with SCO (i.p.)]; pig skin water extracts [PSW 10, 10 mg/kg B.W. (p.o.) with SCO (i.p.)]; PSW 40 [40 mg/kg B.W. (p.o.) with SCO (i.p.)]; low molecular weight pig skin water extracts [LPSW 100, 100 mg/kg B.W. (p.o.) with SCO (i.p.)]; LPSW 400 [400 mg/kg B.W. (p.o.) with SCO (i.p.)].
The cerebral ACh content in the brains of mice dosed with LPSW 400, THA, and CON were significantly higher than those dosed with SCO (Table 4). However, no significant difference was observed among CON, THA 10, PSW 40, LPSW 100, and LPSW 400. Several recent studies have considered the effect of dietary supplementation on the cholinergic system during aging (Willis et al., 2009). ACh is synthesized in pre-synaptic terminals from choline and is required for cholinergic neurotransmission in the central and peripheral nervous systems (Goodman and Soliman, 1991; Srividhya et al., 2012). Shortage of ACh in the brain has been associated with AD. The cholinergic system is strictly dependent on both oxidative metabolism and choline supply (Pervin et al., 2014; Tucek, 1985).
1) Control (CON); scopolamine (SCO, 1 mg/kg body weight (B.W.), intraperitoneally (i.p.); tetrahydroaminoacridine 10 [THA 10, tacrine; 10 mg/kg B.W. per oral (p.o.) with SCO (i.p.)]; pig skin water extracts [PSW 10, 10 mg/kg B.W. (p.o.) with SCO (i.p.)]; PSW 40 [40 mg/kg B.W. (p.o.) with SCO (i.p.)]; low molecular weight pig skin water extracts [LPSW 100, 100 mg/kg B.W. (p.o.) with SCO (i.p.)]; LPSW 400 [400 mg/kg B.W. (p.o.) with SCO (i.p.)].
The cerebral AChE activity in the brains of mice fed PSW and LPSW is shown in Table 4. THA and LPSW 100 and 400 effectively reduced AChE activity to 25% and 26.7%, respectively, which were equivalent to the activity observed in the CON group (23.4%) compared to the activity of SCO group as 100%. However, no significant effect was observed in the treatment groups. We observed that the ACh content increased when the AChE activity decreased. This observation is in agreement with the fact that AChE is an enzyme that catalyzes ACh, and indicated that LPSW 400 affected the cholinergic system, which is highly dependent on oxidative metabolism and ACh release (Pervin et al., 2014).
The hydrophilic species are the major forms of AChE in the brain, muscle, and other tissues, which forms disulfide-linked oligomers with collagenous or lipid-containing structural subunits (Sussman et al., 1991). AChE plays an important role in the ACh-cycle, including in the release of ACh (Srividhya et al., 2012). Jang et al. (2011) reported that supplementation of 1%, 2%, and 4% pig skin gelatin hydrolysates (molecular weight between 3 kDa and 50 kDa, obtained via hydrolysis of pig skin gelatin by AlcalaseTM and ProtamexTM) for 16 weeks significantly reduced AChE levels in the ICR mice brain to 48.9%, 47.8%, and 52.1%, respectively.
MAOs are enzymes located in the mitochondria of the liver and other tissues and modulate the level of neurotransmitters such as dopamine, norepinephrine, and serotonin. Hence, many studies have been attempted to inhibit the MAOs, the levels of which increase with age, for the treatment of central nervous system (CNS) disorders (Zhang et al., 2019). MAO-A and MAO-B levels increased by 6-fold in cardiac tissue and by 4-fold in neuronal tissue with age (Zhang et al., 2019). This increased the release of hydrogen peroxide, leading to oxidative stress and degeneration of CNS tissue (Edmondson et al., 2007). We observed that the MAO-B activity in SCO significantly increased to 12.37 μM resorufin/60 min/mg protein, whereas MAO-B activity in THA, LPSW 100, and LPSW 400 significantly decreased and showed activity similar to that of CON (Table 4). These results demonstrated the beneficial role of pig skin gelatin hydrolysates in the formation of the neurotransmitter ACh by decreasing AChE levels as shown in Table 4, leading to significant reduction in MAO-B activity. Zhang et al. (2019) suggested that dietary antioxidative phenolics such as resveratrol and pterostilbene can also reduce MAO-A and MAO-B levels, respectively.
Conclusion
The low molecular weight hydrolysates generated from pig skin gelatin using Flavourzyme® 1000L can be used as dietary compounds for protecting ACh in brains from AChE, reducing MAO-B activity, and attenuating memory and learning deficit induced by scopolamine. However, this study is preliminary and additional studies are required to understand the metabolic events and gene expression changes occurring after administration of specific peptides from the hydrolysates and their processing by the intestinal and cognitive systems.