Introduction
Scientific evidence during the past decade has demonstrated the vital roles of human gut microbiota on human health, general well-being and brain function through the gut-brain axis (Claesson et al., 2012; Davari et al., 2013; Hsiao et al., 2013). The beneficial microbes in the gut are defined as ‘probiotics’. They are living bacteria that, when administered in adequate amounts, confer a health benefit on the host (FAO and WHO, 2001). Probiotics should exhibit the following properties: antibiotic susceptibility, high autoaggregation, high hydrophobicity, high bile and acid tolerance, and absence of gelatin hydrolysis, virulence gene and hemolytic activity, etc. in order to survive in the human gastrointestinal tract and to exert human health benefits. Fermented foods are well known as rich sources of probiotics. A great number of lactic acid bacteria (LAB) such as Lactobacillus pentosus, L. plantarum, L. fermentum, L. brevis, L. casei, Leuconostoc mesenteroides, L. fallax, L. kimchii, Weissella koreenis, W. cibaria, W. confusa, and Pediococcus pentosaceus (Swain et al., 2014), potent probiotics, were isolated from a variety of Asian fermented foods (Anandharaj and Sivasankari, 2013).
Since 2013, a novel subclass of probiotics called ‘psychobiotics’ has emerged. These psychobiotics were first defined as probiotics that, when ingested in appropriate quantities, produced positive psychiatric effects in psychopathology (Dinan et al., 2013). They were shown to be able to produce neurotransmitters and also exert psychotropic effects in animal models or patients. For example, Proteus vulgaris, Bacillus mycoides, B. subtilis and Serratia marcescens were able to produce dopamine and norepinephrine (Tsavkelova et al., 2000), Bifidobacterium infantis produced the serotonin precursor, tryptophan (Desbonnet et al., 2008), Achromobacter xylosoxidans and Escherichia coli produced serotonin (Hsu et al., 1986), L. plantarum DSM 19463 produced γ-aminobutyric acid (Di Cagno et al., 2010), L. plantarum produced acetylcholine (Marquardt and Falk, 1957) and B. amyloliquefaciens SB-9 produced melatonin, 5-hydroxytryptophan, serotonin and N-acetylserotonin (Jiao et al., 2016).
To test psychobiotic effect of probiotics, a rat model has been commonly used with antibiotics treatment. In general, antibiotic treatment alters the gut microbiota structure leading to distinct behavioral changes in rodents including anxiety-like and depressive-like behaviors and cognitive changes (Guida et al., 2018; O’Mahony et al., 2014; O’Mahony et al., 2017) due to the gut-brain axis network through alterations of brain activity via neural pathways and immune and endocrine mechanisms (Clarke et al., 2014; Mueller et al., 2015; Rodríguez et al., 2015; Zommiti et al., 2018).
Thus, the aim of this work was to determine the psychobiotic effect of a mixture of six bacterial strains provided as a probiotic cocktail on anxiety and memory in male Wistar rats. These multi-strain probiotics from Thai fermented foods might be used as mind/behavior modulator in future applications.
Materials and Methods
The six bacterial strains with probiotic properties were mostly isolated from Thai fermented foods; Pedicoccus pentosaceus WS11 (LC336439.1) from water kefir (Luang-In et al., 2018b), Lactobacillus plantarum SK321 (MH973186.1) from Pak-Sian Dong (Pumriw, 2020) and Lactobacillus fermentum SK324 (MH973188.1) from Pak-Sian Dong (Pumriw, 2020). The remaining three bacteria: Lactobacillus brevis TRBC 3003 isolated from pickled cabbage, Bifidobacterium adolescentis TBRC 7154 isolated from adult intestine and Lactococcus lactis subsp. lactis TBRC 375 isolated from pickled cabbage were purchased from Thailand Bioresource Research Center (TBRC), Pathum Thani, Thailand. All bacteria were stored in 20% glycerol stocks of De Man, Rogosa and Sharpe (MRS) broth pH 6.8 for LAB, Luria-Bertani broth pH 7.0 for Enterobacter and Gifu anaerobic medium (GAM) broth pH 7.0 for Bifidobacterium at −80°C at the Natural Antioxidant Innovation Research Unit, Department of Biotechnology, Mahasarakham University, Thailand.
Multi-strain probiotic mixture was prepared as in the previous method (Liu et al., 2016) but with modifications. Each bacterial strain was subcultured in the corresponding broth twice every 24 h. Bacterial cultures (20 mL) were then inoculated into 1 L of sterile broth and anaerobically incubated at 37°C without shaking. Cells from each bacterial isolate in the early stationary phase of growth (18–24 h) that reached 1×109 CFU/mL were harvested by centrifugation (6,000 g, 10 min, 4°C) and washed twice with sterile saline. The bacterial cell pellets of each strain at a final concentration of 6×109 CFU/mL were combined and re-suspended in sterile 50 mL of 10% skimmed milk containing 5% sodium glutamate. The re-suspended solution was freeze-dried to produce mixed probiotic powder and then stored at −20°C until use. When in use, the mixed probiotic powder (5 g) containing microbes of 6×109 CFU was suspended in 1 mL sterile water. The probiotics were administered daily via oral gavage to rats in the probiotic (PRO) group at 1 mL (6×109 CFU) per rat (at 11 am) for 14 days. We used one single high dose of 6×109 CFU/mL because it has been reported that a high dose of probiotics for example, VSL#3 mixture at 3×109 CFU/day in a rat study and 4.5×109 CFU/day in a human study; were able to produce a longer term positive effect on health (Kim et al., 2005; Shibolet et al., 2002).
Twenty-one eight-week old male Wistar rats were randomly divided into 3 groups (Fig. 1). The first group was the control group (n=7), CON, and included normal rats that received sterile distilled water (1 mL per rat daily) as sham treatment via oral gavage without antibiotics or probiotics for 21 days. The second group was the antibiotics group (n=7), ANT, that included rats treated with the four mixed antibiotics (Table 1) (1 mL per rat daily) for 21 days. The third group was the probiotics group (n=7), PRO, that included rats treated with four mixed antibiotics (Table 1) (1 mL per rat daily) for 7 days and were then given probiotics solution (1 mL per rat daily) for the following 14 days. The rat body weight was monitored and rat feces was collected every 7 days. Behavioral testing was performed between 12:00 pm and 18:00 pm. The rats were single- housed for 60 min in the testing room before the test.
Twenty-one male Wistar rats, 8 weeks old (180–220 g), were purchased from the Northeast Laboratory Animal Center (NELAC), Khon Kaen University, Khon Kaen, Thailand. The rats were acclimatized at NELAC for a week before starting the experiment. Three to four rats were housed for each group in each 37.5×48×21 cm polycarbonate cage under standard fluorescent dark-light cycle (12:12 h) at 23±2°C and 30%–60% relative humidity. The rats were allowed free access to a standard food pellet diet and distilled water ad libitum. Every effort was made to minimize animal suffering in accordance with the principles for laboratory use and care of European Community (EEC directive of 1986; 86/609/EEC) and approved by the Animal Ethics Committee at Khon Kaen University, Khon Kaen, Thailand (IACUC-KKU-60/62).
It is known that the use of four mixed antibiotics (ampicillin, neomycin, metronidazole and vancomycin) as an antibiotic cocktail rather than a single antibiotic was able to directly affect gut microbiota in rats by decreasing abundance, modulating community structure, and lowering bacterial diversity and the approach has been established as an antibiotics-treated rat or mice model (Bruce-Keller et al., 2015; Feng et al., 2019). In this work, the experimental procedure was carried out as described in Zhan et al. (2018) with some modifications. After a 1-week acclimatization period, acquired depletion of colonic microbiota in ANT and PRO groups was shown to be achieved by administering four mixed antibiotics dissolved in water via oral gavage (Table 1) as in the previous work (Zhan et al., 2018). Each antibiotic had different mechanisms of action to prevent growth or kill different bacterial targets as shown in Table 1 and thus a synergistic effect of this antibiotic cocktail to reduce gut microbial amount was expected.
Rat fecal samples were collected from each animal on day 0, 7, 14 and on the day before sacrifice were used for viable bacterial cell counting. Briefly, 1 g of fecal content was suspended in 9 mL 0.85% NaCl saline and vortex-mixed for 1 min. Ten-fold dilution series of the samples were carried out in 0.85% NaCl saline diluent and spread plated on Wilkins-Chalgren agar (WCA, Oxoid, Basingstoke, UK). Plates were anaerobically incubated at 37°C for 3 days (Tulstrup et al., 2015). Bacterial colonies were counted and expressed as Log CFU/g fecal content.
The anxiety/compulsive behavior was assessed using the marble burying (MB) test as described in a previous report (Angoa-Pérez et al., 2013). The rats were acclimatized in the test room for 30 min before testing. Each rat was placed in the center of the cage bedding with saw dust (4 cm thick) and having 20 marbles evenly spaced in five rows of four marbles and each rat was allowed to explore freely without disturbance for 30 min while a video record was made (Sony Action Camera FDR-X3000R, Tokyo, Japan). Four measures were determined as indicators of anxiety. MB (%) was calculated from the number of marbles buried (to 2/3 of their depth) by each rat within 30 min over the total of marbles before the test. Time spent digging (s), freezing (s) and grooming (s) were determined from the recorded videos. Three experimenters were blinded to the treatment groups.
The open field test (OFT) is commonly used to measure rodent behaviors to assess the locomotor function, exploratory level and anxiety level which correspond to dysfunction of the central nervous system (CNS). This test was performed in a 50×50×40 cm open field box (Pramoolsilpa et al., 2017). Total distance traveled and mean speeds were recorded to determine the locomotor function of the rats. In addition, the time each rat spent in the center of the box, the time of freezing, rearing and grooming were also measured to compare the anxiety level of each rat. The rats were acclimatized in the box for 5 min before testing. The rats were then placed in the center of the box and allowed to explore it for 5 min. Rat behavior was video recorded (Sony Action Camera FDR-X3000R) and analyzed using ODLog 2 macropod software (http://www.macropodsoftware.com/odlog/).
This test was designed to measure non-spatial memory of rats based on the hypothesis that a rat is more likely to interact with a novel object than an old or familiar object as in previous reports (Huang and Hsueh, 2014; Mclagan and Hales, 2019). Each rat was placed in the middle of a 50×40×40 cm box in the trial session of 10 min without any disturbance and allowed to explore two identical objects called A and B placed distantly from each other. After 10 min, a rat was allowed to rest in its cage for 30 min and then the testing session of 10 min began, but this time a novel object with distinct shape and similar size to objects A and B called C replaced the old object B. Rat behavior was video recorded (Sony Action Camera FDR-X3000R) and analyzed by using ODLog 2 macropod software (http://www.macropodsoftware.com/odlog/). The recognition index was calculated from the formula.
TC: total duration of exploration with object C in testing session
TA: total duration of exploration with object A in testing session
Rats were placed into an anaesthetic induction chamber for euthanasia and isoflurane was piped into the chamber on day 22. After decapitation, two representative rat brains from each group were dissected by an experienced technician and collected for histology in 10% neutral buffered formalin until further analysis. The other five representative rat brains from each group were dissected after perfusion to obtain the rat prefrontal cortex and hippocampus sections which were immediately frozen on powdered dry ice and stored at −80°C until use for antioxidant activity assays.
The two representative rat brains from each group were cut into small pieces (mm thickness) for tissue processing. Brain sections were embedded in paraffin and cut into 5 μm thick sections using a cryostat microtome (Garman et al., 2015), stained with hematoxylin and eosin (H&E) and subsequently examined under a light microscope (Olympus BX51, Olympus, Tokyo, Japan).
Enzymatic antioxidant capacity of brain samples was determined by the catalase (CAT) activity assay. The rat prefrontal cortex and hippocampus (20 mg) were homogenized in cold lysis buffer (50 mM potassium phosphate, pH 7.0, containing 1 mM EDTA) using TissueLyser LT (Qiagen, Hilden, UK). The homogenate was centrifuged at 10,000 g for 15 min at 4°C and the clear supernatant was obtained for CheKine CAT activity assay kit (Abbkine, Shanghai, China) following the manufacturer’s instructions.
Non-enzymatic total antioxidant capacity of brain samples was measured by the ferric-reducing antioxidant power (FRAP) assay according to a modified method (Benzie and Strain, 1996; Nonato et al., 2016). The reducing capacity of the complex ferric Fe3+-TPTZ (ferric-tripyridyl triazine) to ferrous form Fe2+-TPTZ (ferrous-tripyridyl triazine) of antioxidants at acidic pH relates to the antioxidant power in rats’ brains. FRAP reagent was prepared in 300 mM sodium acetate buffer pH 3.6 by adding acetic acid, 10 mM 2,4,6-tri (2-pyridyl)-s-triazine (Sigma-Aldrich, St. Louis, MO, USA) solution in 40 mM HCl and 20 mM iron (III) chloride solution in proportions of 1:1:10 (v/v), respectively. Briefly, a total of 30 μL of brain homogenate was added to 3 mL of the FRAP reagent, mixed well and incubated in the dark at 37°C for 15 min. The samples were analyzed in triplicate in a M965+ microplate reader (Metertech, Taipei, Taiwan) at 593 nm. Brain non-enzymatic total antioxidant capacity was expressed as microgram of FeSO4 equivalents determined from the standard curve of known amounts of FeSO4 and normalized by the amount of protein in the sample (FeSO4 equivalent μg/μg protein).
Non-enzymatic total antioxidant capacity of brain samples was also measured by the free radical scavenging effect on 2,2′-diphenyl-1-picrylhydrazyl (DPPH) radical (Sigma-Aldrich) as previously reported (Luang-In et al., 2018a). One hundred microliters of 0.2 mM DPPH methanolic solution were added to 30 μL of brain homogenate and the mixture was mixed thoroughly and incubated in the dark at 37°C for 15 min. The samples in triplicate were analyzed in a M965+ microplate reader (Metertech) at 517 nm. Brain non-enzymatic total antioxidant capacity was expressed as microgram of Trolox equivalents (TE) calculated from the standard curve of Trolox (Sigma-Aldrich) and normalized for the protein content in the samples (TE μg/μg protein).
The protein concentration in each supernatant was measured using Quick StartTM Bradford Protein Assay (BioRad, Herculanes, CA, USA) according to Bradford’s method (Bradford, 1976) using bovine serum albumin (1 mg/mL) as the standard.
The data were analyzed by a one-way analysis of variance (ANOVA) using GraphPad Prism software (demo version, GraphPad Software, CA, USA). The normality of the data was checked prior to the ANOVA test in GraphPad Prism using D’Agostino-Pearson omnibus K2 normality test at a significance level of 0.05. If the P value was greater than 0.05, the data was normal. If it was below 0.05, the data significantly deviated from a normal distribution. The results were expressed as mean±SD. The differences among groups were assessed by using Tukey’s Multiple Comparison Test. Statistically significant difference was considered at p<0.05 (*), p<0.01 (**) and p<0.001 (***).
Results and Discussion
This work showed that probiotic supplement did not significantly alter rat body weight in all three groups and the weight gain increased from day 0 by 50%–55% at day 7, 50%–60% at day 14 and 75%–87% at day 22 (Fig. 2A). Initially, microbial populations in rat feces at day 0 in all 3 groups were similar ranging from 8.0 Log to 8.75 Log CFU/g (Fig. 2B). At day 7, after receiving antibiotics for 7 days, both ANT and PRO groups had significant reduction in microbial populations to 6.75 Log and 7.25 Log CFU/g, respectively (Fig. 2B) when compared to the CON group (without antibiotics administration). However, the microbial population in rat feces significantly increased in PRO group to 9.0 Log and 9.5 Log CFU/g at 14 and 22 days, respectively. The microbial population in the ANT group (7.6 Log and 7.5 Log CFU/g at 14 and 22 days, respectively) was significantly lower (p<0.001) than that in the PRO group by 100 fold.
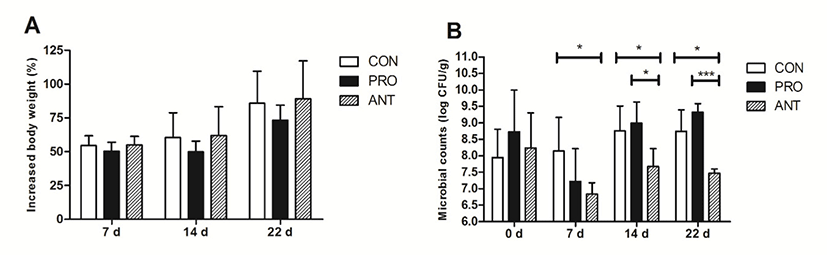
Similar to the previous study (de Sá Del Fiol et al., 2014), no difference in rodent weight gain nor the occurrence of adverse clinical signs in antibiotic-fed rats was observed in this work. In addition, our result was in accordance with the previous finding showing that the administration of an antibiotic cocktail (ampicillin, vancomycin, neomycin, metronidazol and amphotericin-B) to rats led to a minimum of 100-fold decrease in cultivable microbes (Reikvam et al., 2011). However, in the other report (Hill et al., 2010), a 10-fold reduction in the microbial counts was observed when rats were treated with the antibiotic cocktail (ampicillin, neomycin, metronidazole and vancomycin).
Several studies showed that the use of four mixed antibiotics (ampicillin, neomycin, metronidazole and vancomycin) directly affected gut microbiota in rats by lowering abundance, modulating community structure, and decreasing bacterial diversity and thus established an antibiotics-treated rat model (Bruce-Keller et al., 2015; Feng et al., 2019; Yoo et al., 2016; Zhan et al., 2018). Previously, it was shown that ampicillin treatment or antibiotics cocktails significantly reduced bacterial population in rats (p<0.01) during 3 and 7 days based on fecal microbial DNA concentration of 82,502.1+18,255 μg/g in control samples versus 3,417.4+1,212 μg/g in samples following exposure to antibiotics (Zhan et al., 2018). Not only that, but the cocktail also altered microbial diversity and composition. However, the antibiotics regimen was unable to entirely deplete microbiota of the recipient, which could lead to recolonization by specific bacteria (Heimesaat et al., 2013; Reikvam et al., 2011; Ubeda et al., 2013). It is also likely that daily oral gavage could result in some degrees of behavioral changes (McCafferty et al., 2013; Ubeda et al., 2013). In this work, all the three groups of rats received oral gavage daily and presumably all the rats experienced the similar degrees of discomfort during daily oral gavage. Thus, the effect of such potential artifacts was reduced and the behavioral changes in the three groups were likely to be attributed to antibiotic or probiotic administration.
The MB test provided a sensitive and accurate assay of repetitive and compulsive-like behaviors or anxiety-like behavior in rodents (Angoa-Pérez et al., 2013). Grooming behavior was induced by exposure to many stressors and was linked to states of stress or anxiety (Estanislau, 2012) and thus the time spent grooming was measured in this test. Time spent freezing was also recorded as another indicator of fear to explore a new environment (Llaneza and Frye, 2009). Self-grooming was an indirect indicator of rodent repetitive behavior that was translated into abnormality in motor neuron and neural circuit. The lesser time in self-grooming suggested lesser anxious state. However, it is important to note that self-grooming should not be used as a sole or direct indicator of rat anxiety (Kalueff et al., 2016).
The results showed that the ANT group buried significantly more marbles than the PRO group (p<0.05) at 42% and 18% MB, respectively (Fig. 3A); however, the CON group did not show a significant difference in MB when compared with the ANT group suggesting that the two groups with no probiotics administration seemed to be more compulsive and more anxious than the PRO group. Likewise, the longest time of digging, time of freezing and time of grooming at 150 s, 14 s, and 32 s were found in the ANT group corresponding to the most compulsive/anxious state (Fig. 3B, 3C, and 3D, respectively). However, probiotics administration in the PRO group was able to significantly lower the time of digging, time of freezing and time of grooming suggesting that a probiotic cocktail was able to reduce compulsiveness and anxiety.
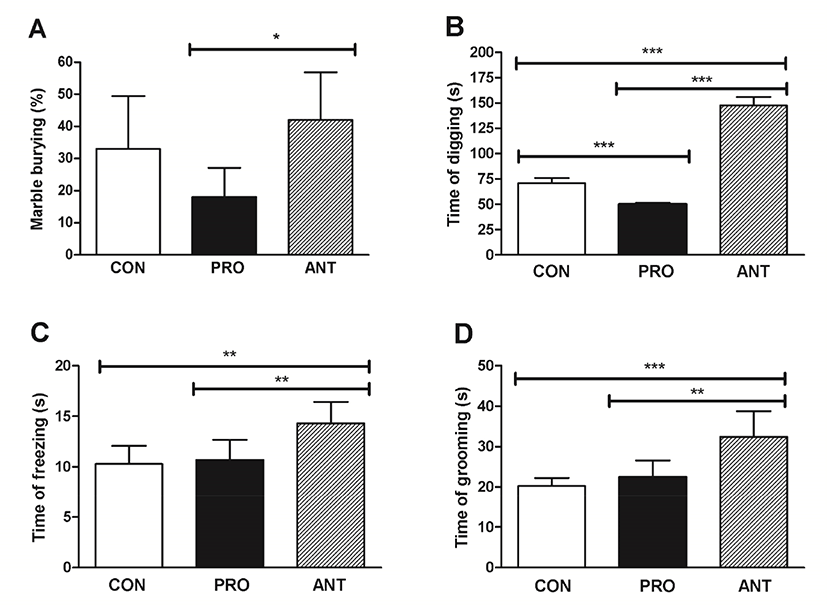
The ANT group showed a distinct behavioral phenotype characterized by repetitive, compulsive-like digging and burying. The overview of the representative cages of the CON group showed few marbles buried (n=7 left and n=1 right in this example) with some degree of displacement from the original marble locations (Fig. 4B) when compared to the marble locations in the cages at the initial time (Fig. 4A). Similarly, the PRO group showed very few marbles buried (n=1 left and n=3 right in this example) and little displacement from the original marble locations (Fig. 4C). In contrast, the ANT group had the greatest number of marbles buried (n=10 left and n=15 right in this example) and an extensive displacement of marbles from the initial marble locations (Fig. 4D). The topographic changes in the bedding surface was assessed, applied as an adjunct to the time spent digging and was also an indicator of burying and digging behavior. Both CON and PRO groups had relatively undisturbed bedding surface appearance (Fig. 4B and 4C) when compared to the bedding surface prior to the test (Fig. 4A). In contrast, the ANT group showed some degree of disturbance on the bedding surface (Fig. 4D).
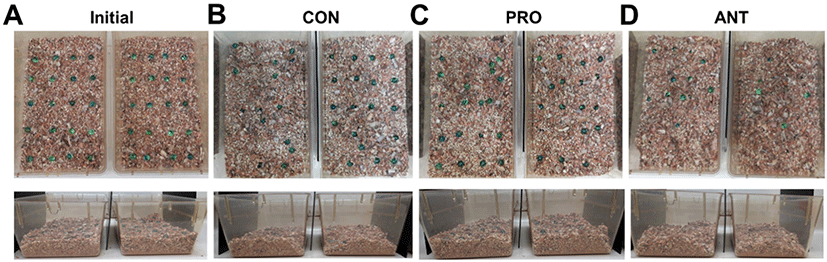
The results showed that the PRO group had significantly greater distance traveled (600 cm), speed (120 cm/min) and time spent in the center of the exploration box (20 s) than those found in the ANT group (370 cm, 74 cm/min and 12 s, respectively) (Fig. 5A, 5B, and 5C) indicating that a probiotic cocktail was able to enhance exploratory behavior, locomotor functions whilst lessen anxiety in rats. The PRO group was also more exploratory than the CON group. In addition, the PRO group spent significantly less time of fear-related freezing (11 s) and less time of rearing (13 s) than the ANT group (14 s and 23 s, respectively) (Fig. 5D and 5E) suggesting that a probiotic cocktail was likely to be able to reduce anxiety. However, the results from the PRO and the CON groups were not significantly different. The time of grooming showed no significant difference among the three groups (Fig. 5F).
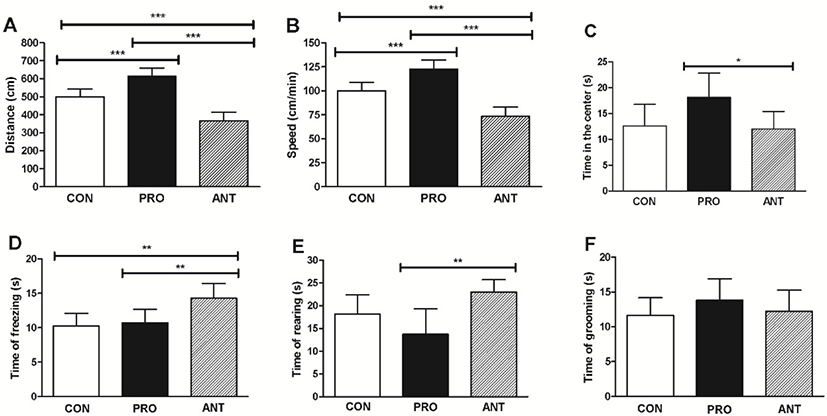
Our findings are similar to the previous works. L. casei 54-2-33 (1×104 CFU/mL of drinking water) was orally administered to male Sprague-Dawley rats for 14 days and the OFT showed significantly higher time spent in the center of the exploration box (Barrera-Bugueno et al., 2017) suggesting that probiotics can reduce anxiety level. Bifidobacterium and Lactobacillus spp. were mostly used as a probiotic cocktail in the previous works and showed the positive effects on some CNS functions with sufficient doses of 109 and 1010 CFU for 2 weeks in animals (Wang et al., 2016).
To investigate whether a probiotic cocktail was linked to an increased preference for novelty as an indicator of a low anxiety level, the performance of rats on a novel object recognition test was assessed. This test was used as an explicit test of novel versus familiar object discrimination and relied on the hypothesis that rats without memory deficit tended to preferentially approach novel objects (Antunes and Biala, 2012). The result showed that the PRO group displayed significantly enhanced recognition index at 89% when compared to the ANT group at 62% and the CON group at 67% (Fig. 6) suggesting that a probiotic cocktail was able to enhance memory performance.
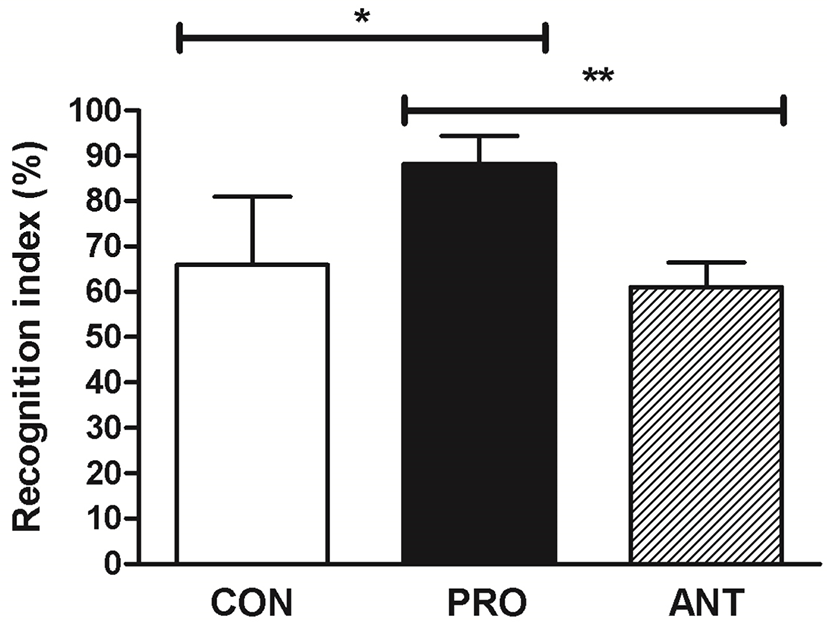
The reason that the ANT group had the lowest memory performance was possibly due to gut dysbiosis caused by antibiotics. The previous reports had demonstrated causal associations between disruption of the gut microbial community and impairments of memory and anxiety-like behavior (Desbonnet et al., 2015; Diaz Heijtz et al., 2011; Fröhlich et al., 2016). The use of a probiotic cocktail in our work produced a similar positive effect on memory as in the previous finding. High-dose (2.5×1010) of commercial probiotics VSL#3 (B. longum DSM 24736, B. infantis DSM 24737, B. breve DSM 24732, L. acidophilus DSM 24735, L. paracasei DSM 24733, L. bulgaricus DSM 24734, L. plantarum DSM 24730, and Streptococcus thermophilus subsp. thermophiles DSM 24731) prevented the diet-induced memory deficits on the hippocampal-dependent place task (Beilharz et al., 2018).
The probiotic cocktail was able to increase a rat brain antioxidant status as supported by a significantly increased CAT activity in the PRO group (65 nmol/min/mg protein) compared to the ANT group (31 nmol/min/mg protein) and the CON group (45 nmol/min/mg protein) (Fig. 7A). No difference in the rat brain DPPH scavenging activity in all 3 groups was observed (p>0.05) (Fig. 7B). However, a significant increase in non-enzymatic total antioxidant capacity estimated by FRAP assay was observed in the PRO group at 0.020 FeSO4 μg/μg protein when compared to the ANT group (0.015 FeSO4 μg/μg protein) and the CON group (0.016 FeSO4 μg/μg protein) (Fig. 7C). Different results from DPPH and FRAP assays may be due to the predominance of probiotics-induced antioxidant molecules with single electron transfer (SET)-based mechanism as detected by FRAP assay over those with hydrogen atom transfer (HAT)-based mechanism by DPPH assay and also ionization potential in rat brains at a particular time might be more favorable for SET mechanism to occur (Ruslan et al., 2018).
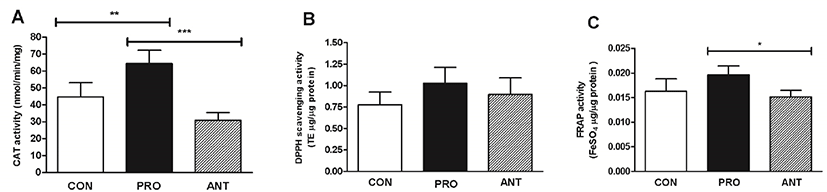
In comparison with the previous work, rats trained by swimming showed no differences in brain antioxidant activity (0.45±0.0 mM/μg protein) assessed by FRAP assay when compared to sedentary rats (0.45±0.0 mM/μg protein) (Nonato et al., 2016). This suggests that probiotic administration is more likely to boost non-enzymatic antioxidant activity in rats than the physical exercise.
The brain is highly susceptible to oxidative stress due to a high density of polyunsaturated fatty acids which are prone to lipid peroxidation. The brain consumes massive amounts of oxygen for energy production, and has lower antioxidant defenses compared to other organs (Adibhatla and Hatcher, 2007). However, the brain expresses CAT enzyme as part of antioxidant defense to decompose hydrogen peroxide, as a result preventing the production of hydroxyl radicals by the Fenton reaction (Reiter, 1995), and protecting the brain from any oxidative damage (Tanko et al., 2013). These results indicated that a probiotic cocktail was able to enhance enzymatic and non-enzymatic antioxidant activities in rat brains and possibly contributed to less compulsive, less anxious, more locomotive behaviors and higher memory performance in the PRO group.
A number of studies suggested that oxidative stress led to anxiogenic behavior; however, the relationship between them was indirect (Xu et al., 2014). Our finding was in accordance with the previous work that showed the enhanced antioxidant activity and total antioxidant capacity in mice with low anxiety-related behavior (Filiou et al., 2011). The previous findings demonstrated that L-buthionine-(S,R)-sulfoximine - induced anxiety-like behavior of rats was preventable by both moderate treadmill exercise and antioxidant tempol supplementation in rats (Salim et al., 2010). In addition, improving learning and memory effects in rats were accompanied by strong antioxidant activity of lipoic acid (Tzvetanova et al., 2018).
The neuroprotective effect of a probiotic cocktail in this work corroborated the finding that displayed the neuroprotective effects of L. buchneri KU200793 isolated from Korean fermented foods (Cheon et al., 2020). In addition, our results were in accordance with the previous work that used eugenol-supplemented diets to treat AlCl3-intoxicated rats and the results showed decreased neuronal cell damage in the cerebral cortex, thereby minimizing damage to the brain tissue (Said and Rabo, 2017).
Probiotics are now well-recognized for their neuroprotective effects; however, the exact mechanisms of actions are still not well established. Evidence on the antiinflammatory activity of probiotics in the CNS has been accumulated. LAB were known to significantly reduce astrocyte reaction in the brain (Kovalenko et al., 2011); Lactobacillus spp. stimulated the release of antiinflammatory cytokines (Villena et al., 2012). Moreover, the neuroprotective effect of L. acidophilus was correlated to higher antioxidant activities (Yang et al., 2011).
In the PRO group, a majority of the pyramidal and granular cells appeared as normal as those found in the CON group (Fig. 8A and 8B); however, certain apoptotic neurons with shrunken acidophilic cytoplasm and deeply stained nuclei were observed possibly as a result of an antibiotic treatment prior to probiotics or naturally-occurring oxidative stress in rat brains. The lesser number of apoptotic cells and shrunken pyramidal and granular cells may be due to the higher antioxidant activity from CAT activity and FRAP activity induced by a probiotic cocktail. Six major layers are recognized in the cerebral cortex and are differently developed in various regions of the cerebral cortex. Pyramidal layers are more developed in the motor centers and granular layers in sensory centers (anxiety and memory) of the cerebral cortex (Swenson, 2006). The less damage in pyramidal layer in the PRO group may contribute to the highest locomotor activity as assessed by OFT and memory performance by NOR.
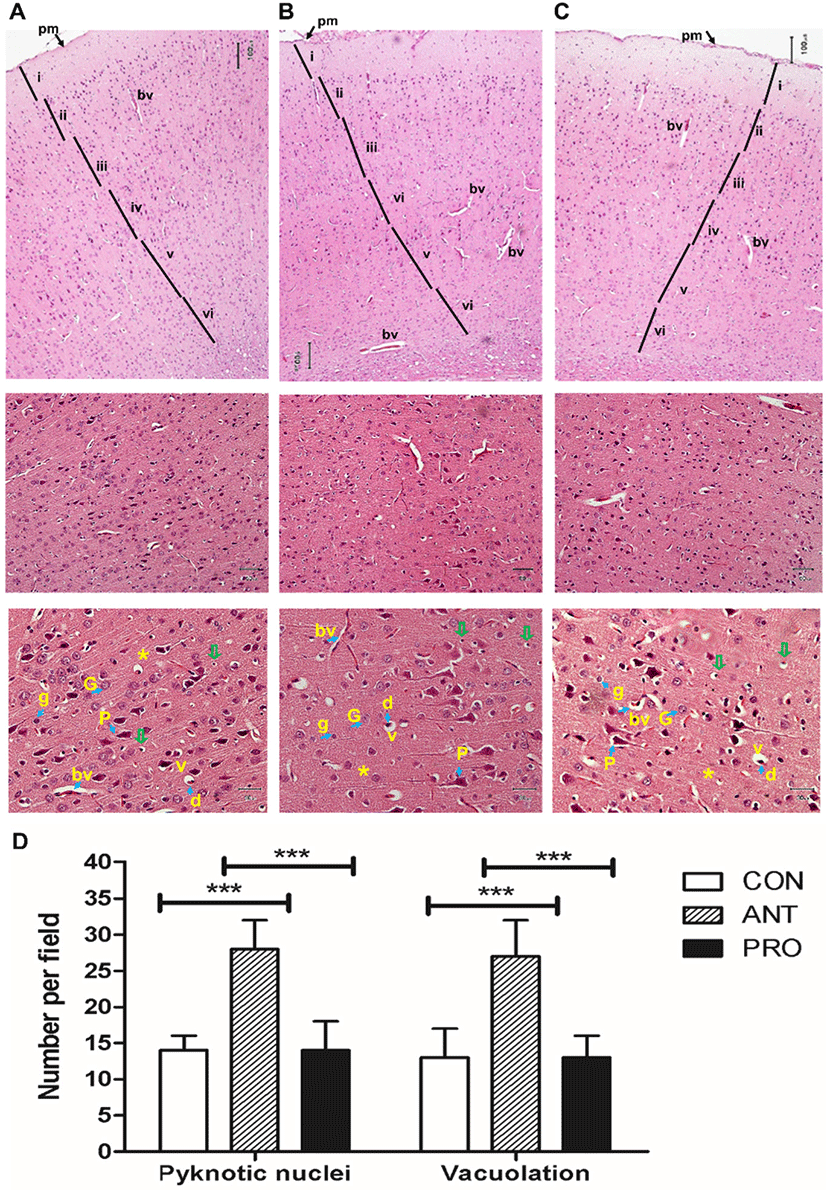
In the ANT group, a greater number of apoptotic pyramidal cells with shrunken acidophilic cytoplasm and deeply stained nuclei was recorded (Fig. 8C) as indicative of neuronal death. This result was similar to the occurrence of neuronal necrosis resulted from the use of adriamycin, a chemotherapeutic drug, in rats (Zickri et al., 2013).
In addition, the ANT group resulted in the highest numbers of pyknotic nuclei and vacuolation per field and significantly different from those found in the CON and PRO groups (Fig. 8D). Vacuolation could be caused by the cell organoid exposure to free radicals (Brown et al., 2004). This effect may be correlated to a reduction in brain non-enzymatic and enzymatic antioxidant causing an imbalance between an antioxidant/oxidant ratio (Brown et al., 2004).
In general, the use of multi-strain probiotics is more favorable than single-strain probiotics due to more effective health benefits (Chang et al., 2017; Chapman et al., 2011). In many cases, multi-strain probiotics were more effective at pathogenic inhibition than individual component species when tested at approximately equal concentrations of biomass. Multi-strain probiotics might be more effective at lowering gastrointestinal infections and may have a broader spectrum of action against different pathogens than that provided by a single strain (Chapman et al., 2012).
In this work, we used six bacterial strains as a probiotic cocktail because we think that mixed probiotics once administered to humans, not only offer synergistic psychobiotic effects as reported in this work, but they individually also offered other health-promoting benefits as reported previously. P. pentosaceus WS11 was proven to be an exopolysaccharide producer (Luang-In et al., 2018b) which exerted antioxidant activity. L. plantarum SK321 and L. fermentum SK324 from Pak-Sian Dong displayed antibacterial activity against four pathogenic bacterial strains; Staphylococcus aureus, Salmonella typhymurium, E. coli and Bacillus cereus (Pumriw, 2020).
Conclusion
The findings in this work are limited but warrant further investigation for advancing scientific knowledge. It is thought that the neuroprotective effects modulated by a probiotic mixture involve antioxidant enzymes, and also non-enzymatic antioxidant defenses. At present, a number of single-strain probiotic and multi-strain probiotics are commercialized worldwide (Ansari et al., 2019). A combination of several species may hold synergistic effects. However, most commercialized probiotics available in Thailand are not derived from strains isolated from Thailand’s origins, most of them are imported from Europe, USA and Japan. To the best of our knowledge, this is the first finding to demonstrate that a multi-strain probiotic formulation derived from bacteria isolated from Thai fermented foods was able to reduce compulsiveness/ anxiety, enhance locomotor function and memory, enhance enzymatic and non-enzymatic antioxidant activities and also offer a neuroprotection in healthy rats. These findings are in agreement with the novel concept of psychobiotics. This work supports the purpose for continuing researches focusing on the use of these probiotic strains for mental health promotion or mind/behavior modulation by the formulation of functional foods.