Introduction
Meat contains high nutritional components that could be utilized by humans to fulfill their regular body requirements. Meat contains nutrition such as protein, vitamin B, iron, and fat to improve the human health (Heinz and Hautzinger, 2007). Amaral et al. (2018) reported that fat play an important role of meat products since it affected the flavor, tenderness, and juiciness of meat products. However, fat was prone to be easily oxidized during processing and storage, resulting in the damage of gastrointestinal tract. Total unsaturated fatty acids that lead to oxidation are increased during storage time. These unstable double bonds of polyunsaturated fatty acid can react with oxygen and lead to lipid oxidation (Min and Ahn, 2005). Pateiro et al. (2015) have found that alcohols, aldehydes, ketones, lineal, and other volatile compounds were increased during storage time, leading to changes of flavor and aroma of dry-cured sausages.
Currently, the food industry has focused on adding natural antioxidants and antimicrobials from plants to meat products. Plant contents such as phenolics, carotenoids, and vitamin can inhibit lipid oxidation by donating electrons and suppressing reactive oxygen species (ROS) (Kalt, 2005). Imparted natural antioxidants such as rosemary extract could reduce total bacteria of fresh beef sausage. The synergistic effect between rosemary extract with mint extract increase the shelf life of beef sausage by preventing microbial growth and inhibiting lipid oxidation during storage (Azizkhani and Tooryan, 2015). Burri et al. (2020) have examined antioxidant abilities of various plants for preventing lipid oxidation and found that sea buckthorn leaves, beet root, onion skin, savory, pine heartwood, olive powder, rhubarb root, black currant leaves, and ramson bulb can significantly retard lipid oxidation of sarcoplasmic protein meat model system from pork knuckle, during storage for more than two weeks.
Eggplant (EP) or brinjal eggplant (Solanum melongna L.) is a vegetable that originally from Africa and domesticated in area between northeastern India and southwestern China. However, nowadays EP were consumed in the world due to the nutritional value of EP and low cost. Domestication of EP primarily involves the expansion of fruit shape, size, and color diversity (Daunay et al., 2001). EP contains ascorbic acid, phenolic, and fiber at around 59–129 g/100 g, 0.74–1.43 g/100 g, and 9–12 g/100 g, respectively. It has various color such as purple, green, and white. Due to its high contents of antioxidant compounds such as ascorbic acid, tyrosine, chlorogenic acid, caffeic acid, and ferulic acid, EP could provide antioxidants for human health (Hanson et al., 2006; Scorsatto et al., 2017). EP peel contains high contents of anthocyanins that give different color to various EPs (Azuma et al., 2008). Various types of delphinidin and nasunin compounds account for large portions of anthocyanins in the peel of EP (Sadilova et al., 2006).
Drying is one method that can be used to increase the shelf life of food. Removing the moisture from food could inhibit the growth of spoilage microorganisms, thus increasing the shelf-life (Ahmed et al., 2013). Drying the African eggplant in oven-dryer (50°C, 60°C, and 70°C) and freeze-dryer (−4°C) improved the ascorbic equivalent antioxidant capacity of eggplant (Mbondo et al., 2018). Martini et al. (2021) reported that the total phenolic compounds and antioxidant activity of dark purple eggplant were higher in cooking eggplant as compared to the fresh eggplant. Drying eggplant using infrared method also improved the total phenolic compounds, potassium, and color properties of eggplant (Jafari et al., 2020). However, the application of the eggplant to the meat products were not widely studied. Therefore, the purpose of this study was to evaluate antioxidant activities of EP powder using different drying methods and its application to meat products with improved the shelf-life during storage.
Materials and Methods
Eggplant (Solanum melongna) was purchased from a local market (Gwangju, Korea). It was cut from the calyx, washed with water, sliced using a knife to a round shape, and then dried using two different methods: oven drying at 60°C and freeze-drying at −50°C until a constant weight was reached. The EP was weighed after dried and put again in dry oven until completely dried or freeze dryer for 2 wk and measure the weight every 1 h to make sure that the EP were dried completely. If the weight of EP was constant after continuous weighing, EP then ground using an Ultra-Power mixer (Hanil, Gwangju, Korea). EP was sieved to two different particle sizes (<500 μm and <300 μm) using sievers (Daihan Scientific, Wonju, Korea) to obtain uniform particles. EP powder was then stored at −20°C until used.
EP powder (5 g) was weighed, added with 100 mL of double distilled (dd)-water, and stirred using a stirrer for 8 h (Sukprasansap et al., 2019). The mixture was centrifuged at 1,500×g for 5 min, filtered using Whatman filter paper (# 41), and used to analyze its antioxidant activity. For the different grade of ethanol extraction, the ratio of EP powder extracted with edible ethanol were 1:20. EP powder (10 g) was weighed and added with edible ethanol (200 mL) at different concentrations (50%, 75%, and 100%). The mixture was then stirred for 8 h and centrifuged at 1,500×g for 5 min. The supernatant was filtered with Whatman filter paper (# 41). The filtrate was evaporated in a rotary evaporator (Rotavapor 110, Hwashin Science, Gwangju, Korea) at 50°C to obtain EP ethanol extract. Its antioxidant activity was then analyzed.
The free radical scavenging activity of EP was measured using 2,2-diphenyl-1picrylhydrazyl (DPPH) radical-scavenging assay according to published method described by Huang et al. (2005). Briefly, EP powders (0.1 g) with particle size and ethanol extracts were diluted in methanol and centrifuged at 1,500×g for 5 min. Ascorbic acid was used as a positive control and starch was used as a negative control. Each sample was mixed with methanolic DPPH (0.2 mM) and kept in a dark place for 20 min. The absorbance of each sample was measured at 517 nm with a UV spectrophotometer (UV/VIS Spectrophotometer X-Ma 1200, Seoul, Korea).
Total phenolics in EP powder and ethanol extracts were measured using the method described by Lin and Tang (2007). Briefly, a standard curve was prepared using gallic acid at 0, 25, 50, 100, and 200 mg/L. Each sample (0.1 mL) was mixed with different concentrations of gallic acid, dd-water (2.8 mL), 2% Na2CO3 (2 mL), and 50% Folin-Ciocalteu reagent (0.1 mL). The mixture was then kept at room temperature for 30 min and its absorbance was measured at 750 nm with a UV spectrophotometer (UV/VIS Spectrophotometer X-Ma 1200, Seoul, Korea). Total phenolics were expressed as gallic acid equivalents (GAE)/100 g dried powder.
Ferrous iron-chelating ability was measured according to the method of Le et al. (2007). EDTA was used as a positive control. Briefly, 0.5 mL of each sample was mixed with 0.5 mL EDTA, 0.5 mL dd-water, 0.1 mL ferrous chloride, 0.9 mL methanol, and 0.1 mL ferrozine 5 mM. The mixture was then incubated at room temperature for 10 min. Its absorbance was then measured at 562 nm with a UV Spectrophotometer.
EP powder and ethanol extracts were diluted in dd-water to final concentrations of 0.1%, 0.25%, 0.5%, and 1.0%. Each sample (2.5 mL) was mixed with 2.5 mL of 200 mM phosphate buffer and 2.5 mL of 1% potassium ferricyanide and incubated at 50°C in an oven for 20 min. The mixture was then mixed with 2.5 mL of 10% trichloroacetic acid (TCA) and centrifuged at 1,500×g for 10 min. Then 2.5 mL of the supernatant of each sample was mixed with 2.5 mL dd-water and 0.5 mL ferric chloride (FeCl3) and kept at room temperature for 10 min. The absorbance of each sample was measured at 700 nm with a UV Spectrophotometer (UV/VIS Spectrophotometer X-Ma 1200, Seoul, Korea) (Huang et al., 2005).
Pork meat and fat were purchased from a local market (Gwangju, Korea). Excessive fat and connective tissues were trimmed. Ingredients of sausages were shown in Table 1. Pork meat and fat were ground using a grinder (Fujee Plant, Busan, Korea, M-12s) and mixed with non-meat ingredients, then moved to a tube (50 mL) to have 40 g of sausages batter per tube. The tube was then centrifuged at 1,500×g for 2 min, boiled at 75°C in a water bath for 30 min, and cooled. After sausages were removed from tubes, they were packaged under the vacuum and stored at 10°C for 35 days. Physicochemical properties and microbial counts of sausages were analyzed at days 0, 3, 7, 14, 21, 28, and 35. The whole experiment was replicated for three times.
CTL, control without adding antioxidants; REF, sausage mixed with 0.1% ascorbic acid; O1, sausage mixed with 0.25% oven-dried eggplant powder; O2, sausage mixed with 0.5% oven-dried eggplant powder; F1, sausage mixed with 0.25% freeze-dried eggplant powder; F2, sausage mixed with 0.5% freeze-dried eggplant powder.
Cooking losses (CL, %) of sausages were determined by subtracting the weight of the sausages before and after cooked. pH values were determined using a pH meter (Mettle-Toledo, Schwerzenbach, Switzerland). Color values, including lightness (L*), redness (a*), and yellowness (b*), were measured using a Minolta color reader (Model # CR-10, Minolta, Tokyo, Japan).
TBARS values were determined using the method of Sinnhuber and Yu (1977). Briefly, 2 g of each sample was mixed with 3 mL of 2.5% TBA and 17 mL of 1% TCA, boiled at 90°C in a water bath for 30 min, and cooled at room temperature. After cooling, 5 mL supernatant of mixture solution was mixed with 5 mL chloroform and centrifuged at 1,500×g for 5 min. Then 3 mL supernatant of chloroform solution was mixed with 3 mL petroleum ether and centrifuged at 1,500×g for 10 min. The absorbance of the sample was measured at 532 nm with a spectrophotometer (UV/VIS Spectrophotometer X-Ma 1200, Seoul, Korea). The TBARS was calculated based on the equation:
Microbial counts were determined using total plate count (TPC) agar plates and Enterobactericeae determined using violet red bile (VRB) agar plates. Each sample (10 g) was mixed with 90 mL sterilized water using a Stomacher (BigMixer, Interscience, Mourjou, France). After mixing, 0.1 mL sample was spread to TPC and VRB agar plates and incubated at 37°C for 48 h.
Texture profile analysis (TPA) was determined using a Universal Testing Machine (Model 3344, Canton, MA, USA). Each sample was cut into 13 mm in length and 12.5 mm in diameter and compressed with a 500-N load cell at a speed of 300 mm/min. The compression ratio of samples was 70% of sample height. Hardness (gf), springiness (cm), cohesiveness, gumminess and chewiness were analyzed for TPA.
Proximate compositions including moisture, fat, and protein of sausages were determined as described by AOAC (2005).
Expressible moisture (EM) was measured by weighing samples (1.5 g) and three pieces of a three-fourth Whatman filter paper (# 3). The sample was folded in a thimble and centrifuged at 1,500×g for 15 min. The sample and thimble were then weighed again after centrifugation. The EM was calculated with the following formulation:
where ΔW was the subtracted weight of thimble before and after centrifugation, and A was the weight of the sample.
Results and Discussion
DPPH radical-scavenging activities (RSA) of EP samples are shown in Figs. 1A and 1B. At 1% concentration of EP different particle size, freeze-dried EP 500 μm had higher DPPH-RSA value than oven-dried EP 500 μm, however particle size did not affect the DPPH-RSA values of EP. As compared to the different ethanol levels, freeze-dried EP extracted with 75% and 100% ethanol had higher DPPH radical-scavenging activity (p<0.05) than extracted with 0% and 50% ethanol. The DPPH-RSA of EP water extract was lower than other extracts (p<0.05). The DPPH-RSA was increased with an increasing concentration of EP powder. DPPH ranged from 46.2%–98.1% for EP with different particle sizes and 0.36%–94.7% for EPs extracted with different solvents, Sukprasansap et al. (2019) have also reported that the DPPH-RSA of freeze-dried EP ranges from 25.10% to 91.60% for six different EPs. Higher DPPH-RSA of EP might protect the human body from oxidative damage known to cause disorders or non-communicable disease. Cai et al. (2020) have found that DPPH of citrus peel was higher in sieved powder with higher particle sizes (500–710 μm) than with small particle sizes (125–500 μm). Lower antioxidants in smaller particle size might be due to cell damage during grinding and more phenolic contents eluted during processing. Ekin et al. (2017) have stated that DPPH-RSA of Crataegus meyeri ethanol extract was higher than that of its water extract. These results indicated that ethanol extract can inhibit the formation of free radical better than water. In this study, increased the concentration of ethanol increasing the antioxidant activity of EP, however particle size of EP did not affect the activity of antioxidants.
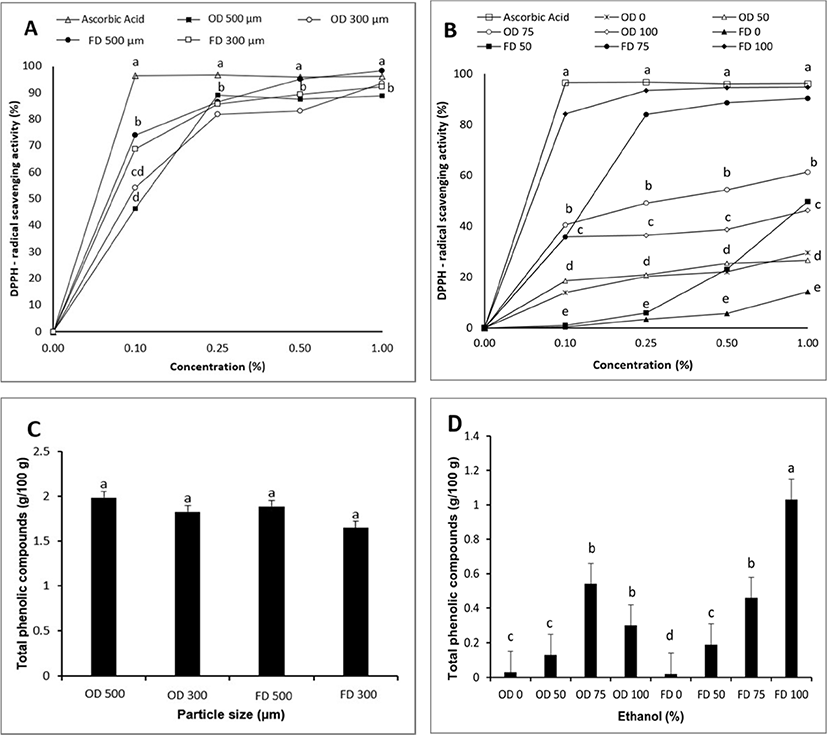
As shown in Figs. 1C and 1D, TPCc of EPs were not different by particle size or drying method. However, they were different by solvent used for extraction. Freeze-dried EP extracted with 100% ethanol (FD 100) had a higher value of TPC than those of extracted with 75%, 50%, and 0% of ethanol. EP extracted with 75% ethanol had higher values than extracted with 0% and 50% ethanol. Ferarsa et al. (2018) have also reported that TPC in EP water extract was lower than that in EP ethanol extract or EP extracted with a combination of ethanol and water. TPC ranged from 0.02–1.99 mg GAE/g in this study. Such differences in TPC were partially due to differences of polarity among solvents. Phenolic contents of various plants were affected by chemicals of plants and polarities of solvents used. Extraction of plants with a solvent could remove non-phenolic content of plants such as sugar and fat (Dai and Mumper, 2010). Sun et al. (2015) have stated that propolis extracted by ethanol or ethanol mixed water had higher antioxidants than that extracted with water alone. Ethanol and ethanol mixed water can extract bioactive compounds of plants with a high polarity. Therefore, higher concentration of ethanol increased the TPC of EP in this study.
Results of iron chelating ability (ICA) were shown in Figs. 2A and 2B. ICA values were increased with increasing concentration of EP. ICA values of EP powder with different particle sizes and drying methods were not different (p>0.05) from each other. With different concentration of ethanol, freeze dried EP extracted with 75% and 50% ethanol had higher value than water extract and 100% ethanol extract at level 1% of EP. In oven-dried EP, different concentration of ethanol did not affect the ICA of EP. Boulekbache-Makhlouf et al. (2013) have reported that iron chelating activity of EP peel ranges from 3.71% to 18.53% in EP extracted with 70% methanol, ethanol, and acetone. These results indicated that EP powder might prevent oxidation and interrupted the formation of radical hydroxyl from ferrozine-Fe2+ known to cause oxidation in food. Emanue et al. (2011) have reported that antioxidant activity of Artichoke (Cynara scolymus) was increased when the level of ethanol used for extraction was increased. The antioxidant content in Cynara scolymus was higher when it was extracted with 75% ethanol, but lower when it was extracted with 97% ethanol. Similar results were found in the present study, showing that mixture of ethanol and water affected the antioxidant activity of freeze-dried EP, however, did not affect the ICA of oven dried EP.
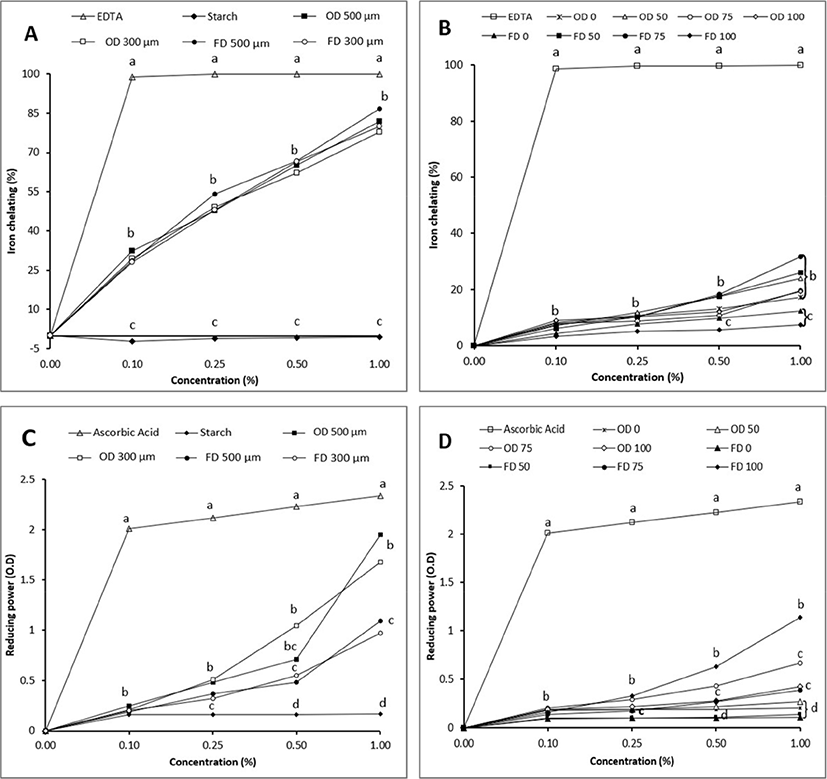
Figs. 2C and 2D shown the reducing power of EP powder in different particle size and concentration of ethanol. Oven-dried EP powder with 300 and 500 μm particle size had higher values than freeze-dried EP (2C). The reducing power of EP was gradually increased with increasing level of EP. In EP different solvent extract, extracting EP with 75% and 100% ethanol had higher reducing power than extracted with 0% and 50% ethanol (2D). The reducing power value was 0.09%–1.95%. Reducing power value of EP ethanol extract was higher than that of water extract. Sukprasansap et al. (2019) have reported that the reducing power ranges from 228.7 to 1,260 μmol TE/g for six different types of freeze-dried EP extracted with 80% methanol. Nisha et al. (2009) have stated that total reducing power was increased with increasing concentration of EP. Reducing power means transformation from Fe3+ to Fe2+ at low pH by antioxidant compounds of plants. Such transformation involves donation of electrons by phenolic compounds to free radicals to protect cells against damage caused by oxidation. Increasing the concentration of ethanol increased the reducing power activity of EP and drying the EP in oven had higher values than freeze-dried EP regardless the particle size of EP.
There was no interaction between treatment and storage time in pH and color, therefore data were expressed by storage time in a treatment or treatment in a storage time (Table 2). pH values of sausages added with EP were not different among treatments and during storage times (p>0.05). Thus, adding EP did not affect the pH of meat products. Pintado et al. (2018) reported the addition of chia and oat in fresh sausage did not affect the pH of sausages during storage time.
CTL, control without adding antioxidants; REF, sausage mixed with 0.1% ascorbic acid; O1, sausage mixed with 0.25% oven-dried eggplant powder; O2, sausage mixed with 0.5% oven-dried eggplant powder; F1, sausage mixed with 0.25% freeze-dried eggplant powder; F2, sausage mixed with 0.5% freeze-dried eggplant powder.
Lightness (L*) values of sausages added with 0.25% and 0.5% oven and freeze-dried EP tended to be lower than sausages without EP. However, these values were not changed during storage. Redness (a*) values was higher in the control and reference, with F2 had the lower redness (a*) value, which was decreased with increasing storage time. Yellowness (b*) value was higher for sausages added with 0.25% and 0.5% oven and freeze-dried EP powder. They were increased toward the end of storage (Table 2). Different color values of sausages added with EP powder might be due to the presence of anthocyanins in the EP powder. The content of anthocyanin in EP peel was about 80–850 mg/kg (Azuma et al., 2008). Yellowness value was higher for sausages added with EP due to the presence of carotenoid compound in EP. However, they were decreased during cooking. Kim and Chin (2013) have also reported that lightness values of sausages added with tomato powder were increased and color values were decreased during storage time except for lightness. This was due to the pigment content of tomato. Thus, pigment of EP affected the color properties of sausages during storage time.
As shown in Table 3, moisture contents (%) of sausages were not different between treatments, however decreased during storage time. Moisture contents (%) in this study ranged from 64.1%–66.4%. Yang et al. (2010) reported there was no different between low-fat sausages added with hydrated oatmeal and without hydrated oatmeal. Moisture contents (%) of sausages added with EP powder ranged from 60.36% to 61.46%. Fat contents (%) of sausages added with EP were lower (p<0.05) than those of control and reference sausages. However, they were increased (p<0.05) during storage time due to loss of moisture. Fat contents (%) ranged from 17.23% to 18.96 %, with sausages added with chitosan having the lowest fat content (Alirezalu et al., 2019). Sausages containing EP powder did not different (p>0.05) in protein content compared to the control. Protein contents did not change during storage time (p>0.05). Protein contents (%) in the study of Powell et al. (2019) were similar to results of the present study, showing no significant difference between treatment groups. They reported that protein contents (%) of sausages added with citrus fiber were approximately 12%. In this study, adding EP did not affect the proximate compositions of sausages.
CTL, control without antioxidants; REF, sausage mixed with 0.1% ascorbic acid; O1, sausage mixed with 0.25% oven dried eggplant powder; O2, sausage mixed with 0.5% oven dried eggplant powder; F1, sausage mixed with 0.25% freeze-dried eggplant powder; F2, sausage mixed with 0.5% freeze dried eggplant powder.
PLs, EM, and VRB values were shown in Table 4. PL values of sausages added with 0.5% freeze-dried EP powder were lower than those of other sausages. PLs ranged from 3.89% to 4.71%, showing no significant change during storage. PLs of control sausages were higher than those of other treatments due to lower protein content in the control. Lower protein content in meat or meat product could increase water loss. EP contains high protein contents that could retain water during storage (Lonergan et al., 2001). Adding EP to sausage did not affect the EM (%) of sausages, although EM was decreased (p<0.05) during storage time due to protein denaturation with increasing storage time. Water holding capacity was a crucial factor affecting the tenderness, juiciness, and flavor of meat products (Adıbelli and Serdaroglu, 2017). VRB agar plates were used to determine the number of coliform bacteria. In this study, VRB counts were not (p>0.05) different among sausages, although they were increased during storage. These results indicate that antioxidants could inhibit the growth of Enterobactericiae in sausages.
VRB, violet red bile; CTL, control without antioxidants; REF, sausage mixed with 0.1% ascorbic acid; O1, sausage mixed with 0.25% oven dried eggplant powder; O2, sausage mixed with 0.5% oven dried eggplant powder; F1, sausage mixed with 0.25% freeze-dried eggplant powder; F2, sausage mixed with 0.5% freeze dried eggplant powder.
TPA was performed based on hardness, springiness, gumminess, chewiness, and cohesiveness. In this study, the hardness value was higher for sausages added with EP powder. It was increased with increasing level of EP powder added. The lowest hardness value was found for sausages added with ascorbic acid (Table 5). Sausages added with 0.5% EP had higher hardness values than control sausages. Springiness, gumminess, chewiness, and cohesiveness were not different by treatment or storage time. Powell et al. (2019) have also reported that hardness values of sausages added with citrus fiber as a natural replacer were increased with increasing level of citrus powder added and that springiness, gumminess, and chewiness were not changed during storage. Uthumporn et al. (2016) have shown that EP powder had high crude fiber content at 15.66–15.77 g/100 g, including insoluble fiber (25.31–28.01 g/100 g), soluble fiber (11.86–12.28 g/100 g), and total dietary fiber (37.18–40.97 g/100 g).
CTL, control without adding antioxidants; REF, sausage mixed with 0.1% ascorbic acid; O1, sausage mixed with 0.25% oven dried eggplant powder; O2, sausage mixed with 0.5% oven dried eggplant powder; F1, sausage mixed with 0.25% freeze-dried eggplant powder; F2, sausage mixed with 0.5% freeze dried eggplant powder.
As shown in Fig. 3A, TBARS values increased with increasing storage time (p<0.05). Increasing the level of EP powder added to sausages decreased their TBARS values. This result indicated that the addition of EP could retard lipid oxidation during storage. In this present study, O2 sausages (0.5% oven-dried EP) had lower TBA values than others (Fig. 3A). Ruban et al. (2009) have found that there was an interaction between treatment and storage time for TBARS values of sausages added with potato and tapioca flour. TBARS values were increased during storage time (30 days). Sausages added with potato flour showed better inhibition of lipid oxidation than those added with tapioca flour. Kumar et al. (2015) have declared that plants have antioxidants such as tocopherols, flavonoids, and phenolic that could suppress lipid oxidation. The antioxidant activity of EP can be partitioned into different phases of the food matrix. Flavonoids usually transfer hydrogens to make free radical reactions ineffective and stabilize the reaction of lipid oxidation (Mbah et al., 2019). Gürbüz et al. (2018) have reported that EP had high contents of phenolic acids such as flavonoid, hydroxycinnamic acids (HCA), and anthocyanin.
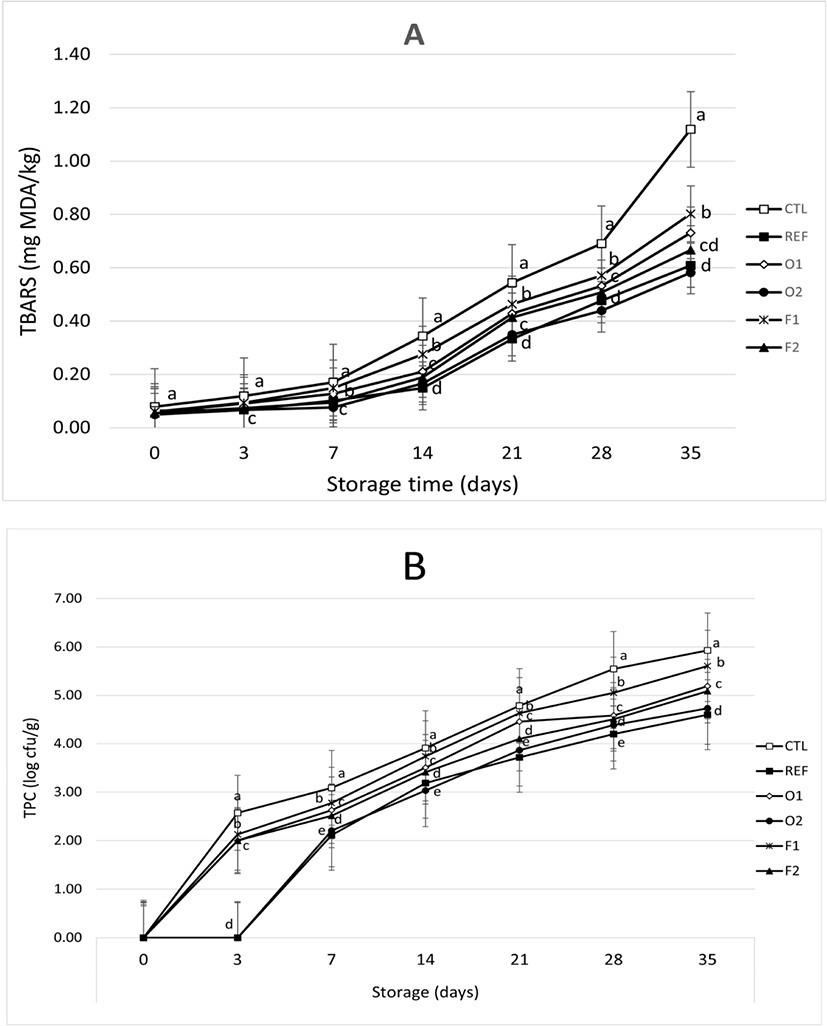
As shown in Fig. 3B, total microbial counts increased during storage time, with the reference (ascorbic acid) having the highest ability to inhibit the growth of bacteria. Control without adding any EP had higher total bacteria counts than other treatments during storage time, ranging from 2.57 Log CFU/g on day 3 to 5.93 Log CFU/g on day 35. Furthermore, total bacteria count could not be detected for reference sausages on day 3. Total bacterial count was 2.11 Log CFU/g on day 7 and 4.5 Log CFU/g at the end of storage time. AL-Janabi et al. (2010) have reported that plants have antimicrobial activities, especially fruits and roots of purple EP. They found that EP fruits could inhibit the growth of Escherichia coli, Staphylococcus aureus, Klebsiella pneumoniae, Proteus vulgaris, Bacillus subtilis, and skin pathogenic fungi. The George Mateljan Foundation (2009) has stated that nasunin and glycoalkaloids of EP could work as antioxidants and antimicrobial agents to protect animal tissues from oxidation.
Conclusion
EP powder could be used as a natural antioxidant in food products. In this study, EP powder was found to possess high antioxidant activities based on analysis results of DPPH-RSA, total phenolic content, ICA, and reducing power. The addition of EP powder at 0.5% improved texture properties of sausages. In addition, EP powder extended the shelf-life of sausage by retarding the lipid oxidation process and inhibiting microbial growth. Compared to freeze-drying, oven-dried EP powder added at 0.5% was effective in extending the shelf-life of sausages.