Introduction
Food additives are natural or synthetic substances that are intentionally added during food manufacturing and processing to improve food properties (Yu et al., 2020). Although food additives have been internationally proven to be safe if they are used according to regulations, controversy over the potential harmfulness of synthetic additives continues (Bearth et al., 2014). Therefore, in recent years, interest in and research on natural additives using medicinal and edible plant materials have been actively carried out owing to consumer concerns about safety and improved awareness of natural products (Kang et al., 2009). However, studies on the criteria for selecting natural materials with appropriate characteristics for use as natural additives are insufficient, and further studies are needed.
Listeria monocytogenes, Clostridium perfringens, Salmonella spp., and Escherichia coli are the main foodborne pathogens isolated from meat products (Cetin et al., 2020; Chang et al., 2020; Park et al., 2021). Preservatives in foods can prevent the growth of spoilage bacteria and foodborne pathogens; thus, it is necessary to use preservatives in food manufacturing, particularly meat products. However, most preservatives used in food manufacturing are chemical additives and may have potential harmful effects in humans; this has led to the perception that chemical additives are hazardous (Falleh et al., 2020). Many natural products, such as fruits, vegetables, and seaweed, have antimicrobial activities and may therefore be used to replace synthetic chemical preservatives in the food industry (Choi et al., 2013). Seaweeds, which are mainly used in Asia, have antimicrobial activity and health benefits (Cabral et al., 2021). Schisandra chinensis (Turcz.) Baill. has antimicrobial activity, and its extract can inhibit L. monocytogenes, E. coli, and Salmonella enteritidis (Choi et al., 2013). Thus, the development of natural preservatives that can reduce foodborne pathogens may improve food safety and overcome the negative perceptions of consumers.
Meat products contain a number of food additives, which consumers would prefer to avoid (Lee et al., 2020; Yong et al., 2020). Accordingly, clean-label meat products are becoming more popular (Yong et al., 2021), and replacement of synthetic preservatives, such as butylated hydroxyanisole (BHA) and butylated hydroxytoluene (BHT), with natural preservatives is being investigated (Hwang et al., 2013). Importantly, in meat products, preservatives and antioxidants, including BHA and BHT, can prolong shelf-life by enhancing lipid oxidation and antimicrobial activity (Lee and Paik, 2016). Indeed, lipid oxidation in emulsion sausages results in deterioration of color, flavor, texture, and nutritional value during storage (Domínguez et al., 2019). Accordingly, most studies on the shelf-life of meat products prepared using natural extracts have focused on inhibition of lipid oxidation to improve antioxidant power, and research on improving antibacterial activity is limited.
Accordingly, in this study, we aimed to develop a natural preservative that could replace sorbic acid in emulsion sausage while showing good antimicrobial and antioxidant activities.
Materials and Methods
Plant material of 49 species in this study were collected from local market in Korea. There are 42 species of domestic products and 7 species of imported products (Table 1). Natural plants were freeze-dried and ground into a powder with a grinder (Cgolenwall, Hangzhou, China) before being used for extraction (Table 1). Three grams of natural plant powder was added to distilled water and 50% ethanol (40 mL), and the mixture was stirred at 120 rpm for 24 h. Distilled water and 50% ethanol extracts were prepared at 90°C and room temperature, respectively. The extracts were centrifuged at 2,691×g for 5 min, and the supernatants were pooled. Samples were then filtered using filter paper (Whatman No. 1) to remove any impurities. The ethanol extract was extracted using a rotary evaporator (Eyela N-3000; Shanghai Eyela, Shanghai, China) to remove the organic solvent. Finally, the sample was lyophilized and stored in a deep freezer at –70°C.
Four foodborne pathogens (L. monocytogenes, C. perfringens, Salmonella spp., and E. coli) related to foodborne illnesses in meat products were used (Table 2). Salmonella spp. and E. coli strains were activated in 10 mL tryptic soy broth (TSB; Becton, Dickinson, and Company, Sparks, Philadelphia, PA, USA) and incubated at 37°C for 24 h. C. perfringens strains were activated in 10 mL prereduced TSB and incubated under anaerobic conditions at 37°C for 24 h. L. monocytogenes strains were activated in 10 mL TSB with 0.6% yeast extract (TSBYE) and incubated at 30°C for 24 h. Next, 0.1 mL of the culture was transferred to 10 mL TSB or TSBYE and incubated under different incubation conditions. Bacterial cells were centrifuged at 1,912×g for 15 min at 4°C, and the cell pellets were washed twice using 0.85% sterile saline. The bacteria were then resuspended and used for experiments.
Spot assays were performed to confirm the antimicrobial activities of the natural extracts. Bacterial mixtures were diluted with 0.85% sterilized saline to adjust to 6–7 Log CFU/mL. Cells were then overlaid on Muller-Hinton agar (MHA; Becton, Dickinson, and Company) using cotton swabs and then air-dried for 15 min at room temperature. Natural extracts (50 or 200 mg/mL) described in Table 1 were spotted with 10 μL MHA. The plates were incubated at 30°C (L. monocytogenes) or 37°C (C. perfringens, Salmonella spp., and E. coli) for 24 h, followed by measurement of the diameter of the bacterial inhibition zone.
Total polyphenol content was determined according to the method of Folin and Denis (1912). Briefly, extracts (1 mL) prepared in different solvents were mixed with 5 mL distilled water and 0.5 mL Folin-Ciocalteu reagent (1 N). The mixtures were allowed to react for 5 min, and 1 mL sodium carbonate (5%) was then added. The mixture was vortexed and left in the dark for 1 h at room temperature. The absorbance was measured at 725 nm using a spectrophotometer (Optizen 2120 UV Plus; Mecasys, Daejoen, Korea). The total polyphenol content was calculated using a standard curve prepared using gallic acid.
The electron donating ability was measured according to the DPPH free radical scavenging method described by Blois (1958). Extracts of natural plants (100 μL) were mixed with 100 μL of 0.4 mM DPPH solution. The mixtures were left in the dark for 10 min at room temperature, and absorbance values were measured at 517 nm using a spectrophotometer (Optizen 2120 UV Plus; Mecasys). The half-maximal inhibitory concentration (IC50) was expressed as the concentration of the sample that decreased the absorbance of DPPH by 50%. DPPH free radical-scavenging activity was calculated using the following formula:
where Asample is the absorbance of the reaction solution to which the sample was added, and Acontrol is the absorbance of the reaction solution to which ethanol was added instead of the sample.
Fresh pork ham muscles (semitendinosus, semimembranosus, and biceps femoris muscles) and pork back fat were obtained from a local processor 48 h postmortem. The visible connective tissue was removed from the ham muscle, and lean meat and pork back fat were chopped using a 3-mm plate. The emulsion sausage was formulated with ground lean meat (50%), pork back fat (25%), and ice water (25%). Emulsion sausage was prepared using the method described by Sembring and Chin (2021). Briefly, ground lean meat was homogenized, ground for 20 s in a silent cutter, and then mixed with ice water. Salt (1.5%) was added to the mixture for 1 min, and pork back fat was then added after 3 min. The natural extract (2.0%) was added after 5 min and combined using a silent cutter. The meat emulsion batters were homogenized for 4 min, and the temperature of the meat emulsion batter was maintained below 10°C. The meat emulsion batter was stuffed into a collagen casing and then cooked at 85°C for 30 min in a smoke chamber (MAXi3501 chamber; Kerres, Postfach, Germany). The sample was cooled until the core temperature reached room temperature (21°C). Each portion of emulsion sausage was placed in a polyethylene bag, and a portion of emulsion sausage was packed as described earlier and used for storage analysis after 0–15 days.
The emulsion sausage (5 g) was homogenized (8,000 rpm) with distilled water (20 mL). The pH of each homogenate was determined using a pH meter (Mettler-Toledo GmbH, Schwerzenbach, Switzerland).
The CIE L*, CIE a*, and CIE b* values of emulsion sausages were determined using a CR-410 colorimeter (Minolta, Tokyo, Japan) calibrated using a white plate (Illuminate C Observer 2°).
Lipid oxidation was determined using the TBARS method described by Tarladgis et al. (1960), with modifications. Ten grams of emulsion sausage was blended with 50 mL distilled water and 50 μL BHT at 10,000 rpm for 60 s and then transferred to a distillation tube. The cup used for blending was rinsed with an additional 47.5 mL distilled water, which was added to the same distillation flask with 2.5 mL of 4 N HCl and 1 mL antifoam agent. The distilled mixture was collected, and 5 mL of 0.02 M 2-thiobarbituric acid was then added to the test tube. The sample solution was mixed well, heated in a water bath for 30 min, and cooled to room temperature for the reaction between malondialdehyde (MDA) and 2-thiobarbituric acid. The absorbance was measured at 538 nm using a UV/Vis spectrophotometer.
Analysis of antimicrobial activity showed that the natural extracts exhibited the strongest antimicrobial effects against C. perfringens; thus, C. perfringens growth was tested on sausages manufactured with selected natural extracts. Sausage samples were cut into pieces weighing approximately 10 g, transferred to a sterile plastic container containing 3 Log CFU/mL C. perfringens, and then stirred using a sterile spoon for 2 min. The inoculated samples were air-dried at room temperature for 15 min, and each sample was then vacuum-packed in sterile plastic bags and stored at 20°C for up to 14 days. C. perfringens cell counts in sausages were analyzed on days 0, 1, 2, 3, 4, 6, 8, 10, 12, and 14. Then, 90 mL of 0.85% sterile saline was added to the sample bag, and the sample was mixed vigorously for 2 min. The solution was serially diluted with 0.85% sterile saline. Diluents were plated on C. perfringens agar with selective supplement and egg yolk (TSC; Oxoid, Basingstoke, Hampshire, UK) and then overlaid with 10 mL egg yolk-free TSC. The plates were then incubated anaerobically at 37°C for 24 h. Only typical C. perfringens colonies (black and positive for lecithinase activity) were counted.
The data were analyzed using two-way analysis of variance and Duncan's multiple range test (p<0.05) with statistical package for the social sciences (SPSS) statistical software (SPSS Ver. 20.0; IBM, Chicago, IL, USA). For evaluation of emulsion sausage, the addition of natural extract and the storage period were considered fixed terms, and replicates were considered random terms.
Results and Discussion
Among the natural extracts evaluated in this study, 13 natural extracts prepared by ethanol extraction showed antimicrobial effects against one of the tested bacteria, and three natural extracts prepared by water extraction showed antimicrobial activity (Table 3). Based on this result, natural extracts prepared by ethanol extraction were found to be more effective than those prepared by water extraction, and ethanol was used to prepare natural extracts. Ethanol has been known as a good solvent for polyphenol in natural compounds, and polyphenol, found in natural compounds, have been known having antibacterial activity (Coppo and Marchese, 2014). To identify the correlation between bacterial inhibition and the concentration of natural extracts, high concentrations of natural extracts (200 mg/mL) were tested for antimicrobial activity. From the results, we confirmed that antimicrobial activity also increased as the concentration of the extract increased (Table 4). For Schisandra chinensis extract (no. 4), the antimicrobial activity increased as the concentration of the extract increased. At a concentration of 50 mg/mL, antimicrobial activity was only observed for C. perfringens, whereas at a concentration of 200 mg/mL, antimicrobial activity against L. monocytogenes and E. coli was also observed. Furthermore, the antimicrobial activity of Ecklonia cava (no. 23) was improved against all three pathogens, except C. perfringens, when a concentration of 200 mg/mL was used. By contrast, extracts of Elaeagnus umbellata Thunb. f. nakaiana (Araki) H. Ohba (no. 27) did not show concentration-dependent effects, and better antimicrobial activity was observed at 50 mg/mL. Punica granatum L. peel (no. 32) and Rubus coreanus Miq. (no. 36) extracts had antimicrobial activity against all four pathogens, and the activity increased as the concentration increased. Nelumbo nucifera Gaertner (ovary) extracts (no. 46) inhibited only C. perfringens, and the inhibitory effect was concentration-dependent.
Schisandra chinensis has antimicrobial activity, and its extracts can inhibit L. monocytogenes, E. coli, and Salmonella enteritidis (Choi et al., 2013). E. coli is inhibited by the release of cellular contents, resulting in destruction of the bacterial cells, following incubation with Schisandra chinensis extracts (Song et al., 2018). Furthermore, Eckloniacava, a seaweed, is also known to have antimicrobial activity against foodborne pathogens, such as Salmonella spp. (Choi et al., 2010; Roohinejad et al., 2017). Elaeagnus umbellata Thunb. f. nakaiana (Araki) H. Ohba is a wild shrub found in Korea (Potter, 1995) and has been shown to have antimicrobial activity against various bacteria; thus, this plant has also been used as a substitute for antibiotics (Manandhar et al., 2019). P. granatum L. is a medicinal plant that is rich in bioactive phenolic compounds, such as flavonoids and hydrolysable tannins (Singh et al., 2018). Although P. granatum L. peel is not edible, it contains many bioactive phenolic contents that are beneficial to health (Abid et al., 2017), and its extracts have been reported to be nontoxic (Singh et al., 2018). P. granatum L. interferes with the growth of various bacteria, including E. coli, L. monocyogenes, and Salmonella spp., and has antimicrobial effects by destroying bacterial cells or inhibiting enzyme reactions (Hanani et al., 2019). Various papers and many other studies have reported the anti-bacterial effects of polyphenols from natural substances including citrus, Ecklonia cava and Cynanchi wilfordii (Eom et al., 2015; Fratianni et al., 2019). Chibane et al. (2019) reported that polyphenols from natural substances exhibit high antibacterial activity and strong anti-oxidant effects. Naz et al. (2007) revealed that the compounds isolated from P. granatum L. such as gallic acid and quercetin exhibit antibacterial activity toward species of corynebacteria, staphylococci, streptococci, Bacillus subtilis, Shigella, Salmonella, Vibrio cholera, and Escherichia coli (Naz et al., 2007). Rubus coreanus contains anthocyanins, which have antimicrobial effects, and can inhibit E. coli and gram-positive bacteria (Jeon et al., 2012). In addition, phlorotannins including dieckol and phlorofucofuroeckol isolated from Ecklonia cava exhibit antimicrobial activity. These results indicate that polyphenols and phlorotannins from natural resources could be used as anti-bacterial agents in foods (Choi et al., 2014). Rubus coreanus contains anthocyanins, which have antimicrobial effects, and can inhibit E. coli and gram-positive bacteria (Jang et al., 2019; Kim et al., 2015). In 2001, C. perfringens was reported to be inhibited by N. nucifera extracts (Kim et al., 2001); however, most previous studies have evaluated the antimicrobial activity of extracts against L. monocytogenes, Salmonella spp., and E. coli, and few studies have evaluated antimicrobial activity against C. perfringens. Notably, in our study, there were more extracts with antimicrobial activity against C. perfringens than other foodborne pathogens, highlighting the activity of these extracts against spore-forming bacteria. Taken together, the results of this study provided insights into the discovery of natural extracts that could inhibit foodborne pathogens, such as C. perfringens.
The total polyphenol contents of the different extracts are shown in Table 5. Total polyphenol contents increased the following order: Schisandra chinensis (Turcz.) Baill<Elaeagnus multiflora Thunb.<R. coreanus Miq.<Ecklonia cava<P. granatum<N. nucifera Gaertner. The polyphenol contents of 50% ethanol extracts were higher than those of water extracts for all samples tested. When using the water extraction method, the total polyphenol contents of N. nucifera Gaertner, P. granatum, and Ecklonia cava were 10.07%, 8.17%, and 3.61%, respectively. By contrast, the total polyphenol contents obtained using the 50% ethanol extraction method were 22.73%, 12.51%, and 10.53%, respectively. These results were consistent with several studies showing that the total polyphenol contents were higher in ethanol extracts than in water extracts (Do et al., 2014; Thummajitsakul et al., 2020).
The DPPH radical scavenging activities of the various extracts are shown in Table 5. The DPPH radical scavenging activities of water extracts of N. nucifera Gaertner, P. granatum, and Ecklonia cava were 0.27, 1.43, and 4.49 mg/mL, respectively, whereas those of ethanol extracts were 0.10, 0.06, and 2.73 mg/mL, respectively. With the exception of R. coreanus Miq., all 50% ethanol extracts exhibited lower DPPH radical scavenging activities than water extracts (p<0.05). Moreover, higher polyphenol contents were associated with stronger antioxidant activities, similar to a previous report (Do et al., 2014).
Changes in the pH and color values of meats during storage and the effects of various extracts on pH and color values of meats are shown in Table 6. Changes in pH can be induced by various physicochemical or microbial phenomena during storage. On 0 day, T5 had the lowest pH value, followed by T2 and T6 (p<0.05), and all treated sausages had lower pH values than control sausages. This difference may be related to the unique characteristics of the natural extracts (Ramachandraiah and Chin, 2021). In addition, PC samples had lower pH values than NC samples (p<0.05), which may be related to the increased sodium ion concentration following the addition of sodium sorbate (Kim et al., 2019b). When the effect of storage was observed, the pH value tended to decrease slightly as the storage period increased. This reduction could be related to various factors, including differences in the components and metabolites of the microorganisms as well as existing oxygen in the packaging, which could induce pH changes during storage (Liu et al., 2009; Shin et al., 2017).
Color values were also affected by treatment and storage period (Table 6). On day 0, the intrinsic color values of the natural extracts had an impact on the color values of the emulsion sausages. When comparing the CIE L* value, the control group had the highest value (p<0.05). Among the treatment groups, T1 had the highest value, and T6 had the lowest value (p<0.05). For CIE a*, the control groups had lower values than the treatment groups, and T6 had the highest value (p<0.05). The CIE b* of T2 was the highest, and that of T6 was the lowest (p<0.05). The addition of pigmented natural extracts may explain these differences in CIE values. Shin et al. (2017) also observed the impact of natural extracts on the color of meat products, and other previous studies that applied natural extracts to meat products also showed significant differences in meat product color (Kim et al., 2019a; McMurtrie et al., 2012). As the storage period increased, there were no significant differences in CIE L* values between the control groups and the T4 group; CIE a* values among the PC, T3, and T4 groups; and CIE b* values among the control, T1, T2, T4, and T5 groups (p>0.05). Overall, only the CIE L*a*b* values of T4 did not change during storage. Color pigments, such as myoglobin, flavonoids, carotenoids, and anthocyanin, can be changed by oxidation, and loss of color pigments in natural extracts or meat products may explain these changes (Jeong et al., 2020; Suman and Joseph, 2013). According to Table 5, N. nucifera ovary had the highest polyphenol contents and the lowest value in IC50 of DPPH activity was also observed. With these high antioxidant capacity, N. nucifera ovary may have the highest color stability when applied to meat products.
NC, negative control (sausage with no preservative); PC, positive control (sausage with sorbic acid); T1, sausage with Elaeagnus umbellata Thunb. f. nakaiana (Araki) H. Ohba (27); T2, sausage with Punica granatum L. (peel) (32); T3, sausage with Ecklonia cava (23); T4, sausage with Nelumbo nucifera Gaertner (ovary) (45); T5, sausage with Schisandra chinensis (Turcz.) Baill. (4); T6, sausage with Rubus coreanus Miq. (36).
Lipid oxidation in meat products affects the taste, flavor, color, and texture of the meat, thereby representing an important quality characteristic (Domínguez et al., 2019). When lipid oxidation occurs, the predominant aldehyde formed is MDA (Fernández et al., 1997). In this study, MDA content was measured using TBARS tests to determine the lipid oxidation value, and the results are shown in Fig. 1. All groups showed the lowest TBARS values on 0 day (p<0.05). The TBARS values significantly increased with increasing storage days. After 8 days of storage, the TBARS values of the NC and PC samples were over 0.60 and 1.60 mg MDA/kg meat, respectively. In general, the TBARS threshold for consumer acceptance is 0.5 mg MDA/kg meat, after which rancidity begins to be detected (Fernández et al., 1997). Thus, we concluded that sausages in the NC and PC groups should not be stored for longer than 2 and 8 days, respectively. However, the treatment groups prepared with natural extracts had TBARS values lower than 0.5 mg MDA/kg for all storage days. The lowest TBARS values were detected in T4 and T6 (0.05 mg MDA/kg) at 0 days of storage, whereas T4 had a TBARS value of 0.07 mg MDA/kg after 15 days of storage (p<0.05). When the treatment groups were stored for 15 days, the TBARS values increased in the order of T4<T6<T2=T3<T5 (p<0.05).
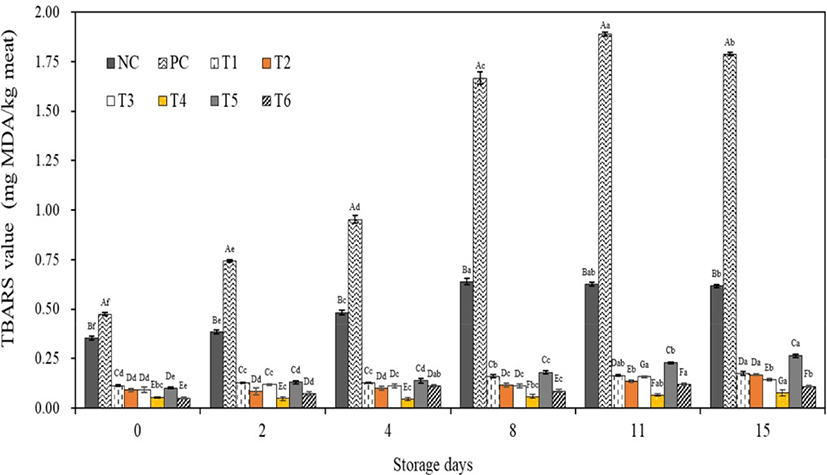
Lipid oxidation is primarily caused by the autocatalytic mechanism of free radicals, called autoxidation. This continuous free-radical chain reaction is the most important process of lipid oxidation in meat and meat products (Fernández et al., 1997). Accordingly, when sources with radical scavenging effects are added to meat products, they can retard the lipid oxidation process (Domínguez et al., 2019). The DPPH radical scavenging effects of the natural extracts evaluated in this study are presented in Table 5. The findings suggested that these radical scavenging activities could have affected the TBARS values of the treatment groups (T1–T6).
Various studies have been conducted to determine the effects of potassium sorbate on the lipid oxidation value of meat products; however, the results have been inconsistent. When raw chicken breast and leg were dipped in 5% potassium sorbate and stored for 30 days, TBARS values were significantly increased compared with those in the control (Kolsarici and Candogan, 1995). Hsu and Sun (2006) also reported that increasing potassium sorbate content (0%–0.2% addition) increase the TBARS value of emulsified meatballs. The addition of potassium sorbate to emulsified meat balls significantly affect the post-storage TBARS (p<0.05). Beef patties with 4% potassium sorbate also had no significant effect on TBARS values (Negbenebor et al., 1995). By contrast, ground buffalo meat preblended with 0.3% potassium sorbate showed lower TBARS values than the control on days 0 and 8 of storage (El-Aal, 2005). Overall, our current findings suggested that using natural extracts (particularly T4) could inhibit lipid oxidation in emulsion sausages as an alternative to potassium sorbate.
Next, we evaluated C. perfringens growth in sausages without preservatives (NC), sausages with sorbic acid (PC), and sausages with natural extracts (T1, T2, T3, T4, T5, and T6) for 14 days. The average initial concentration of C. perfringens was 2.2 Log CFU/mL. C. perfringens growth did not changed until the first day after storage in any of the treated groups. However, C. perfringens growth was significantly (p<0.05) different between samples prepared with and without natural extracts. Any C. perfringens colony was not detected in sausages with natural extracts, whereas C. perfringens growth was observed in sausages without natural extracts. Interestingly, sausages containing sorbic acid, which is a commonly used chemical additive, did not differ (p>0.05) compared with sausages lacking chemicals and natural preservatives. In the NC and PC groups, C. perfringens counts increased to 6.6 and 6.5 Log CFU/g, respectively. By contrast, in all sausages prepared using natural extracts (T1, T2, T3, T4, T5, and T6), a 99% reduction in bacterial counts was observed compared with that in untreated groups on day 2, reaching over 99.9% after day 3 (Fig. 2). Based on these results, the six natural extracts evaluated in this study could stop the growth of C. perfringens and kill this bacterium. These could be resulted that the natural extracts in sausage had an effect on the C. perfringens cell membrane or changes its internal pH (Gonelimali et al., 2018).
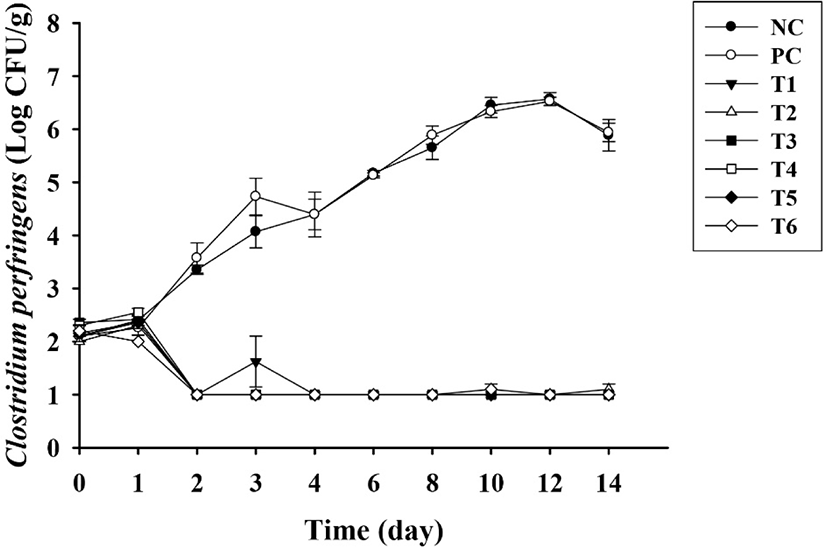
In a study by Chang et al. (2020), chitosan was added during the preparation of sausages, and the applicability of chitosan as a preservative was confirmed. As the amount of chitosan added increased, the growth of C. perfringens was suppressed, similar to the results of our study. In addition, inhibition of C. perfringens growth was observed in sausages formulated with essential oils extracted by Satureja montana L., an aromatic herb (de Oliveira et al., 2011). Aspalathus linearis is a medicinal plant rich in polyphenols, and A. linearis extracts have been shown to inhibit C. perfringens growth in meat products (Park et al., 2014). Moreover, C. perfringens contaminated with pig trotters formulated with A. linearis in an anaerobic environment was found to decrease gradually, although the antimicrobial activity was observed to be somewhat lower compared with that in our findings; in our study, C. perfringens counts were below the detection limit (1.0 Log CFU/g) from day 2, whereas in the study by Park et al. (2014), counts were as high as 3.0 Log CFU/g on day 15. Sous-vide products are prepared by placing in a vacuum, and C. perfringens, which are anaerobic foodborne pathogens that form spores, can be more problematic in these products (Smith et al., 2021). Extracts obtained from natural ingredients, such as citrus and lemons, were used to treat sous-vide chicken products, and the products were found to be protected from C. perfringens growth, confirming the potential applications of natural extracts (Smith et al., 2021). The cell counts of C. perfringens in sous-vide chicken products formulated with citrus and lemons were 3.4 and 2.9 Log CFU/g, respectively (Smith et al., 2021). Thus, the antimicrobial activities of citrus and lemon extracts were slightly lower than those of the natural extracts identified in our study, suggesting that our natural extracts had excellent antimicrobial activities. The natural extracts contain bioactive compounds such as tannin, alkaloids and quinones (Vaou et al., 2021). These compounds affect the cytoplasmic membrane structure and permeability, making it impossible to function properly (Vaou et al., 2021). It is also known to inhibit the quorum sensing of pathogens and efflux pump related with antimicrobial resistance (Radulovic et al., 2013; Savoia, 2012; Vaou et al., 2021). Based on the results of this study, it is considered necessary to investigate how the natural extracts used in the study inhibit the growth of C. perfringens.
Conclusion
In this study, we evaluated the antimicrobial activities of 49 natural product candidates against L. monocytogenes, C. perfringens, Salmonella spp., and E. coli, and six natural materials with excellent antibacterial activities were selected. The antioxidant activities of the six selected natural extracts were investigated, and natural extracts with antimicrobial and antioxidant activities were used to prepare emulsion sausages. Lipid oxidation and C. perfringens growth were then analyzed. Overall, our findings confirmed the antimicrobial activities and lipid oxidation effects of the six selected natural extracts during storage, suggesting that these natural products may be good substitutes for sorbic acid. Accordingly, the natural extracts prepared in this study may have potential applications as natural preservative sources for meat products.