Introduction
One of the key systems that corresponds to oxidative deterioration, generating rancidity, is lipid oxidation in food products, which occurs during storage and processing (Harlina et al., 2015). The negative consequences of lipid oxidation eventually result in nutritional imbalance, negative physiological impacts, vitamin and fatty acid (FA) retrogression. Furthermore, the lipid oxidation reaction produces hazardous products that cause a variety of negative consequences, such as pathological changes in the mucous membrane, decreased enzymatic capacity, increased cholesterol and peroxide levels in the blood, and finally, accelerated atherosclerosis disease within the arteries (Karpińska et al., 2001). The carcinogenic potentiality of the products was previously proven to be caused by a lipid oxidation process. As a result, using antioxidants to limit and manage lipid oxidation is an effective strategy for product improvement (Karpińska et al., 2001).
Antioxidants are substances that, at certain doses, inhibit or postpone oxidative reactions by a mechanism that usually involves the oxidation of the antioxidant itself (Mendis et al., 2005). Natural antioxidants have various advantages, including being well-liked by customers, being secure and safe, and requiring fewer regulatory requirements. Some materials, such as vitamins, phenolic components (flavonoids, terpenoids, and carotenoids), and phytoestrogens, can have antioxidant capabilities (Calucci et al., 2003).
Antioxidants have long been used in food processing to keep the oxidation process running smoothly. In recent years, the use of antioxidant spices to inhibit oxidative reactions has gotten a lot of attention in the food industry. As a natural functional spice, rosemary extract (RE) has become the focus of interest. RE have been widely employed as flavoring agents in the food industry, and have been shown to have substantial antioxidant properties (Nogala-Kalucka et al., 2005). The antioxidant properties of RE were linked to its phenolic content, according to Mc Carthy et al. (2001). RE has a significant advantage over synthetic compounds [butylated hydroxyanisole (BHA) and butylated hydroxytoluene (BHT)] in decelerating the oxidation reaction of oils (Frutos and Hernández-Herrero, 2005). The ability of rosemary to reduce lipid oxidation in food products has been proven in numerous researches (Estévez et al., 2005; Shahidi et al., 1992). Frankel et al. (1996) found that rosemary effectively inhibited the generation of hydroperoxides.
Previous research (Harlina et al., 2015; Harlina et al., 2018; Harlina et al., 2019) found that adding natural spices like garlic oil, clove extract, and galangal extract to salted duck eggs had a significant impact on quality characteristics, microstructural analysis, and flavor properties when compared to the control. Based on these findings, we hypothesized that RE’s functional features would influence the quality aspects of egg products. Nonetheless, there has been no systematic study to date that has demonstrated the beneficial effect of RE’s antioxidant activity on salted duck egg. The purpose of this study was to determine the effect of RE in duck eggs by measuring their in vitro antioxidant capacity, lipid oxidation, and FA profiles.
Materials and Methods
Ethanol, NaOH, NaCl, potassium ferricyanide, FeCl3, 2-thiobarbituric acid, trichloroacetic acid (TCA), chloroform (99.5%), methanol, ethylenediamine tetraacetic acid disodium salt, isooctane, acetic acid, Na2HPO4·12H2O, NaH2PO4, and 2-propanol were obtained from Sinopharm Chemical Reagent (Shanghai, China). P-anisidine purity 99% was obtained from Aladdin Industrial Corporation (Shanghai, China). 1-diphenyl-2-picrylhydrazyl (DPPH) was purchased from Shanghai Yuanye Biological Technology (Shanghai, China). n-Hexane for chromatography (grade 99.5%) was purchased from Tianjin Guangfu Fine Chemical Research Institute (China). Borontrifluo-ride-methanol (BF3-MeOH purity>50% (14wt/v%) was obtained from ANPEL Scientific Instruments (Shanghai, China).
Approximately 350 fresh duck (Anas platyrhucus) eggs weighing 65–75 g, which were retrieved within 3 days of laying. RE was bought from Henan Yuzhong Biotechnology, China. RE was extracted from dried rosemary (Rosmarinus oficinalis L.) which is water-soluble/oil-soluble materials (supplementary Fig. S1). In addition, the extract contains the main components such as rosmanol, carnosol, and carnosic acid. Moreover, the studied RE composition is in agreement with Nakatani and Inatani (1981), Zhang et al. (2010), and Rocío Teruel et al. (2015) who proved that carnosol, rosmanol, and carnosic acid are the main compound in the RE.
Fresh duck eggs were cleaned manually with water before being salted by soaking in a 13% salt solution (salt solution: egg=1:1, w/w). The egg samples were then treated with RE at concentrations of 0.1% and 0.5% (w/v). Salted duck eggs were prepared without RE as a control sample. After that, all samples were kept at room temperature (25°C) for 28 days. For the thiobarbituric acid reactive substances (TBARS) testing, the salting time was increased to 49 days. The experiment was carried out in three consecutive replications, with analysis taking place once a week. With minor adjustments, the sample preparation process employed here was the same as that used by Harlina et al. (2015).
The egg white and yolk were mixed with 95% ethanol in a 1:10 (w/v) ratio and extracted at 60°C in a water bath for 2 hours with continuous shaking at 170 g force. The resulting slurry was centrifuged for 10 min at 10,000×g using a centrifuger (Model 3740, Kubota, Osaka, Japan). The antioxidant activity of the supernatant was then measured. DPPH radical scavenging activity and reducing power assays were used to assess the antioxidant potential of salted duck egg supplemented with RE (0.1% and 0.5%, w/v).
With minor adjustments, the free radical scavenging ability (RSA) of eggs was investigated using the methodology of Harlina et al. (2015). The ability of antioxidants to donate hydrogen atoms or electrons on egg samples was tested by bleaching a purple-colored ethanol solution of DPPH. The stable radical DPPH is used as a reagent in this spectrophotometric assay. At a concentration of 0.002%, DPPH was prepared. In each test tube, different amounts of supernatant were used to make quantities up to 2 mL. Then, in each test tube, 2 mL of DPPH solution was added, and the solutions were maintained in the dark for 30 min. All of the samples were tested three times. Later, using a UV-Visible spectrophotometer, the optical density was measured at 517 nm (NanoDrop 2000C spectrophotometer, Thermo Fisher Scientific, Waltham, MA, USA). As a control, ethanol was combined with DPPH. The following is the calculating formula:
Where: A = optical density of control; B = optical density of sample.
The reducing power assay was evaluated according to the method specified by Xiao et al. (2014). Briefly, 2 mL sample was combined with 2 mL sodium phosphate buffer (pH 6.6) and 2 mL potassium ferricyanide (1%, w/v). For 20 min, the above mentioned solution was incubated at 50°C. After cooling, 2 mL of 10% TCA were added. The supernatant was collected (about 2 mL) and mixed with 2 mL of H2O and 0.4 mL of 0.1% (w/v) FeCl3 after centrifugation at 3,000×g force for 10 min. The mixture was then placed in a 25°C for 10 min, after which the absorbance was measured at 700 nm. As a blank, the same volume of H2O was used.
With minor modifications, the egg yolk lipids were extracted using the method of Wang et al. (2014). Yolk samples (30 g) were homogenized in a homogenizer for 2 min at 11,000×g force in a combination of chloroform, methanol, and H2O (120:120:60, v/v/v). Ultrasound (20°C, 80% power, 30 min) was used to treat the homogenizer. A Büchner filter funnel was used to filter the mixture. The chloroform phase (bottom phase) was drained off into an Erlenmeyer flask. A little amount of anhydrous sodium sulfate (1–2 g) was added, and the mixture was firmly shaken to eliminate any remaining water. Through a filter paper, the lipid in the chloroform was decanted into a round-bottom flask (Whatman No. 1). A rotary evaporator was used to evaporate the chloroform at 55°C, and the remaining solvents were flushed off with nitrogen. The lipid was kept under nitrogen at –20°C in an amber vial until it was tested.
The fatty acid methyl ester (FAME) of egg yolk lipid was studied using gas chromatography - mass spectrometry (GC-MS) (Wang et al., 2014). Using a DB-WAX capillary column, the experiment was carried out on an Agilent 7890B/5977A/7693 Autosampler Series Gas Chromatograph (Agilent Technologies, Palo Alto, CA, USA; 30 m×0.25 mm×0.25 mm film thickness, Agilent Technologies). Split injection at a ratio of 10:1 was used to evaluate the experiment, with ultra-high purity helium as the carrier gas and a flow rate of 1.0 mL/min. Temperatures for the injector and detector were 250°C and 260°C, respectively. The temperature was set at 150°C for 0 min, then increased to 230°C at a rate of 10°C/min for 15 min. The experiment lasted 23 min, and the injection amount was 0.2 mL. The mass spectrometer was operated in electron impact mode with continuous scanning from m/z 50 to 500 at 70 eV of ionization voltage. By comparing the mass spectrum data to the NIST 11 library database and referring the literature, the detection of FAME in egg yolk lipid was investigated. Only components having a resemblance of greater than 60% (highest similarity is 100%) were included in this study.
As indicated in previous research, oxidative rancidity of fat was assessed using TBARS assay (Harlina et al., 2015). The conjugated dienoic acid (CDA) content of duck eggs was determined using methods of Kim et al. (2013). One hundred milligrams of samples were dissolved in 25 mL isooctane and let to stand for 10 min. A UV–Vis spectrophotometer was used to measure the absorbance at 233 nm after the combinations were diluted with 10-fold isooctane (v/v). For each sample, three replicates were measured. The CDA was determined using the formula below:
Where, A is the absorbance at 233 nm, b is the path length of the cell (cm), c is the con-centration (g-1L), and K0 is the absorptive of the acid groups (0.03).
The p-anisidine value (p-AV) of duck egg yolk was calculated using the methods of Kim et al. (2013). A UV-Vis spectrophotometer was used to test the absorbance of the sample (100 mg) in 25 mL of isooctane. After mixing the aforesaid mixture (2.5 mL) with 0.5 mL 0.5% (w/v) p-anisidine in acetic acid, the absorbance was measured at 350 nm after 10 min. Each sample was made in three different ways. The following expression was used to calculate the value of p-AV:
Where A1 is the absorbance at 350 nm before the addition of p-anisidine, A2 is the ab-sorbance at 350 nm after the addition of p-anisidine, and W is the weight of the sample (g).
Headspace solid phase microextraction (HS-SPME) GC-MS was used to evaluate the volatile taste components of salted duck egg yolk control and egg treated with RE. The GC-MS analysis of volatile flavor compounds in both egg-treated groups was carried out on an Agilent 7890B/5977A/7693 autosampler Series Gas Chromatograph (Agilent Technologies) with a DB-WAX capillary column (Agilent Technologies; 30 m×0.25 mm×0.25 mm film thickness, Agilent Technologies). SPME Fiber Assembly (75 m CAR/PDMS Fused Silica 24 Ga, Manual Holder, 3 pk; Supelco, Bellefonte, PA, USA) was utilized for the volatiles fiber. The fiber was put into the GC injector before analysis at a temperature of 250°C. HS-SPME was determined by incubating 5 g of fresh egg yolks for 120 min at 50°C in a 20 mL glass vial. The volatiles were then directly desorbed in the GC injection port for 5 min at 250°C using split less injection at 250°C inlet temperature and 260°C MS detector temperature. The carrier gas was ultra-high purity helium at a pressure of 7.07 KPa. The program temperature was set to 40°C for 2 min, then to 230°C at 5°C/min for 5 min, then to 230°C for 10 min. Mass spectra were taken at a temperature of 250°C and an ionization voltage of 70 eV in the electron impact mode, with a scan range of 30–500 m/z and a scan period of 0.69 s. The mass spectra of the volatile flavor compounds were compared to the NIST 11 mass-spectral database to determine their qualification.
A FOX-3000 E-nose (Alpha MOS, Toulouse, France) was used to examine the flavor of yolks. It included a sampling device, an array of sensors, an HS-100 autosampler, air generating equipment, and software (Alpha Soft V11) for data collection and analysis. The sensory array consisted of 18 metal oxide sensors that were separated into three chambers: LY, T, and P. Yolk samples (3 g) were precisely weighed into 10 mL headspace vials, sealed, and extracted for 30 min at 25°C. The vials were then put into the autosampler tray and extracted for 10 min at 50°C with 200 mL/min of pure air as the carrier gas. 2,500 mL, 3 s, and 250 g were the injection volume, duration, and stirring rate, respectively. The acquisition time was 50 seconds, while the time between injections was 180 seconds. The analysis was based on the E-highest nose’s response points, which were automatically recorded for each of the 18 sensors (Wang et al., 2014). For each sample, three replicates were measured.
Experiments were carried out in triplicate on salted duck egg with two different concentrations of RE (0.1% and 0.5%, w/v). The results were shown as mean values with SD. SPSS 16.0 was used to perform the ANOVA (SPSS, Chicago, IL, USA). Duncan’s multiple-range test was used to identify the least significant difference between means at a significance level of 0.05. PCA has been used to compare e-nose data between groups.
Results and Discussion
The RSA by DPPH method and reducing power values of RE are presented in Fig. 1. The scavenging ability of RE was significantly different with those BHT and gallic acid (p<0.05). For the seven working solutions, the scavenging percentages of RE were 62.23%, 55.62%, 53.41%, 46.33%, 41.68%, 40.19%, and 37.16%, respectively. The dose-dependent absorbance of RE’s reducing power activity is 2.94, 2.68, 2.51, 2.37, 2.08, and 0.85 for six working concentrations, respectively.
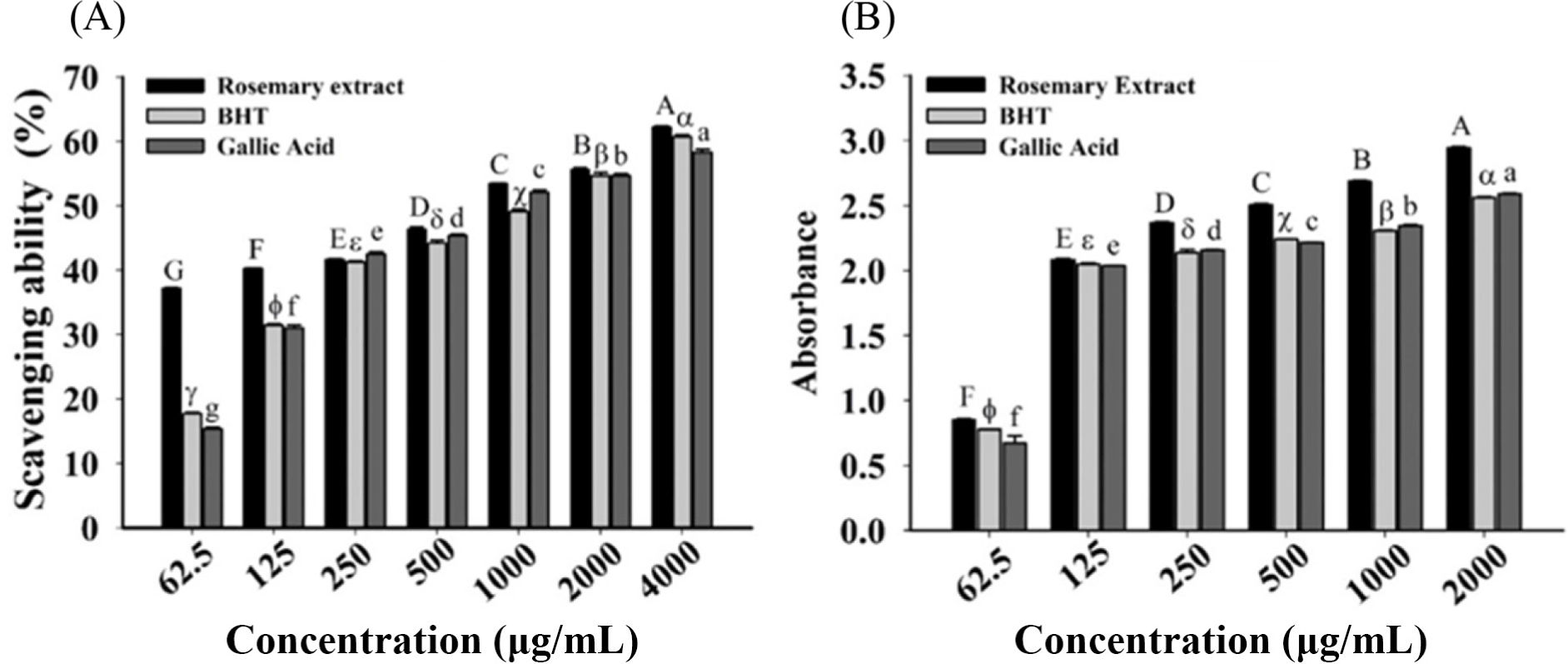
RE is a powerful antioxidant that is widely used in the food preservation, fragrance, and aromatherapy industries (Azizkhani and Tooryan, 2015). The presence of some phenolic diterpenes components, such as carnosol, rosmanol, carnosic acid, rosmariquinone, and rosmaridiphenol, has been linked to the antioxidant activity by stopping the chain reactions of free radical molecules by contributing a hydrogen atom (Zhang et al., 2010). Rosemary has the ability to extend the shelf life of food and protect its quality during storage. As a result, rosemary was greatly used in the food industry as a bio-preservative (Ojeda-Sana et al., 2013). Additionally, rosemary has a variety of health benefits due to its antioxidant properties. According to the European Medicines Agency (EMA) in 2010, rosemary is used to treat gastrointestinal illnesses such dyspepsia and moderate irregular disorders, as well as minor peripheral circulatory disorders and discomfort from minor muscular and articular injuries (Rašković et al., 2014).
RE was identified to quench superoxide radical elements (Rocío Teruel et al., 2015). Rosmanol 3 and carnosol were identified to be more potential than α tocopherol, BHT, and BHA (Nakatani and Inatani, 1981). An attempt to study and compare the antioxidant abilities, RE found to have a higher ability of antioxidation due to the presence of phenolic components, which are more than essential oil extracted from black seeds (Erkan et al., 2008). Amarowicz et al. (2004) investigated an explicit connection between antioxidant capacity and reducing power assay, regulated by free radical molecules. Antioxidation mechanism of REs found to be closely related to that of polyphenols based mechanisms. RE, greatly applied in food preservations, approved as one of the powerful spices. For food items processing, it is commercially consumed as flavoring spice in the US and EU.
The effect of RE on RSA of salted egg over 28 days of salting is presented in Fig. 2A. The results showed that the RSA of salted eggs supplemented with 0.5% (w/v) RE was significantly greater than the control samples (p<0.05) after 28 days of salting for both egg white and egg yolk samples. Synthetic antioxidants had fewer potentials than rosmanol and carnosol (Nakatani and Inatani, 1981). The antioxidant ability of rosemary is similar to that of other phenolic components. The catechol class’s presence in the C11–C12 aromatic particle ring of the rosemary phenolic diterpene structure is the key structural fraction in the phenolic components’ antioxidant potential (Shan et al., 2005). As a result, extracts with a high concentration of phenolic components, particularly carnosic acid and carnosol, can block the formation of free radical molecules and produce higher antioxidant capabilities (Rocío Teruel et al., 2015). Rosemary has been investigated as a lipid oxidative inhibitor, metal chelator, and superoxide radical quencher.
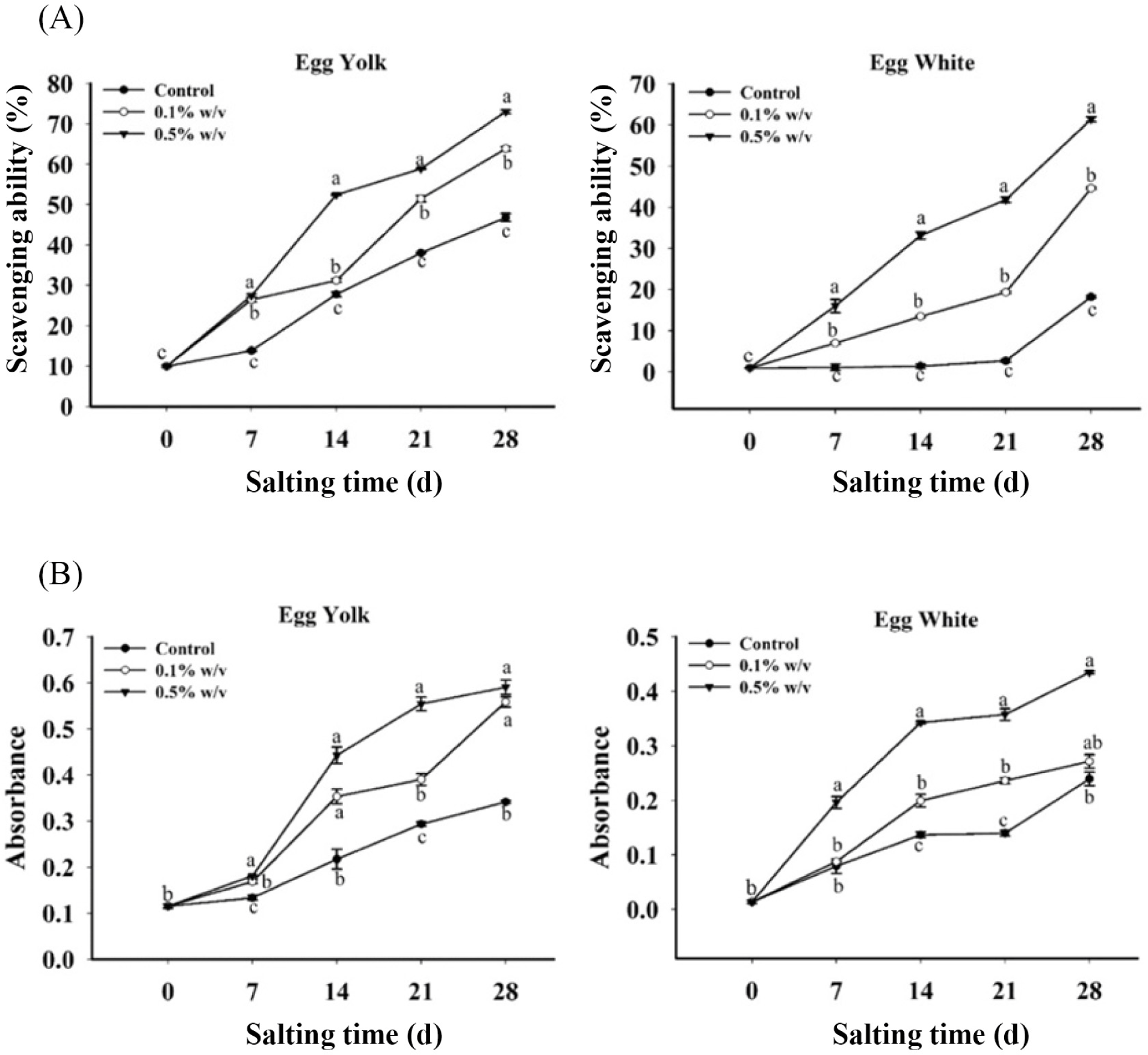
The synthetic antioxidants provide a simple aromatic ring with one hydroxide fraction donating hydrogen. At the same time, RE particularly carnosic acid, provides a single particle ring but provide more two hydroxide ions that can serve as hydrogen contributors. Additionally, adjoining OH fractions can chelate the prooxidative metals so that could inhibit oxidation via 2 mechanisms. And rosmarinic acid provides two aromatic rings, each with two hydroxide ions that able to donate a hydrogen atom and chelating the metals. There are rosemary substances, which contribute important collaboration to the antioxidative ability of extract. It could be a solubility action of the rosemary portions used compared with α-tocopherol which added to the food processing (Brewer, 2011). In the lipid system, carnosic acid and carnosol successfully chelate metal ion and extinguish peroxyl radical. RE was able to quench the DPPH radicals (0.2 μm) with ratios 39% and 55% at the concentrations 100 and 500 mcg/mL; RE at the concentration of 100 mcg/mL prevented liposome (egg lecithin with ferric iron/hydrogen peroxide) oxidation by 98% (Brewer, 2011).
The reducing power activity is commonly used to assess the potential of natural antioxidants to provide an electron (Dorman et al., 2003). According to You et al. (2010), reducing power ability occurs in analyzed samples due to the presence of antioxidants, which causes the Fe3+/FeSCN combination to degrade to the Fe2+ form. At 700 nm, the maximum absorption indicated the best potential to reduce power (Duh, 1998). Depending on each component, the yellow color of the sample reagent changes to green or blue during the processing assay. The ferricyanide complex is subtracted by antioxidants in the reduction step, resulting in the simple ferrous form. As a result, measuring the establishment of Perl’s Prussian blue at 700 nm can reveal the Fe2+ level (Ferreira et al., 2007). Fe (III) degradation is widely seen as a measure of electron contributing ability, which is a significant step of phenolic antioxidant pretension (Dorman et al., 2003).
The reducing power assay of salted duck egg treated with RE (0.1% and 0.5%, w/v) during 28 days of salting is shown in Fig. 2B. The results showed that as the salting time was increased, the reducing power assay climbed. At 28 days after salting, the reducing power of salted duck eggs treated with 0.5% (w/v) RE was substantially higher (p<0.05) than control samples for egg white and egg yolk samples. The salted duck egg treated with 0.5% RE (w/v) had the maximum reducing power of egg white and egg yolk, followed by 0.1% (w/v) and the control sample, respectively. Various phenolic components, which are hydroxyl fractions on an aromatic particle ring, are found in plants. By donating hydrogen or chelating ion metals, phenolic components interfere with chain oxidation responses, acting as antioxidants and reducing agents (Bursal and Köksal, 2011). RE is made up of phytochemical components that are known to have antioxidant properties. Carnosic acid breakdown products, such as rosmanol and carnosol, have been shown to have powerful antioxidant properties (Ban et al., 2016; Liu et al., 2013).
The use of antioxidants is a contemporary technique for preventing lipid oxidation. Fig. 3A shows the effect of antioxidant spices on the TBARS values of salted duck eggs after 49 days of salting. The TBARS assay measures the amount of malondialdehyde produced by secondary lipid oxidation products (Qi et al., 2015). TBARS levels tended to rise as salting time increased. Over the whole salting period, TBARS levels for salted egg yolk treated with RE were considerably lower than those for the control (p<0.05). This implies that the antioxidant inhibited lipid oxidation during the salting process. RE’s antioxidant properties are used to characterize and stabilize lipids and dietary lipids. RE’s potency is typically used to extend induction times during the lipid oxidation process. Phenolic diterpenes are the most common chemicals in RE. Several studies have looked into the effectiveness of RE in preventing lipid oxidation in food products, such as Jordán et al. (2014) who used 500 mg/kg to 1,000 mg/kg in beef steaks; Ortuño et al. (2014) who recommended 200 mg/kg or 400 mg/kg concentrations to improve meat quality during preservation; Sebranek et al. (2005) found that supplementing precooked frozen sausage products with 1,000 mg/kg of antioxidants was just as effective as synthetic antioxidants (BHA/BHT) in lowering TBARS levels. Naveena et al. (2013) found that adding carnosic acid from dried rosemary leaves to cooked chicken patties reduced TBARS by 37% to 87% during refrigerated storage when compared to control samples. The antioxidant effects of rosemary are mostly attributable to rosmarinic acid, with carsonic acid and carnosol serving as minor contributors to the lipid systems. When RE comprising the three key antioxidant chemicals is applied in a proper method, the oxidation of lipidic and aqueous phases of lipid systems can be reduced (de AR Oliveira et al., 2016). Since TBARS is a marker for the formation of secondary products of lipid oxidation, the results showed that adding RE to the salted duck egg significantly reduced lipid oxidation. Because it has DPPH scavenging abilities and reducing power capabilities, it was proven that the RE reduced lipid oxidation by blocking radical chain assault during the oxidation process.
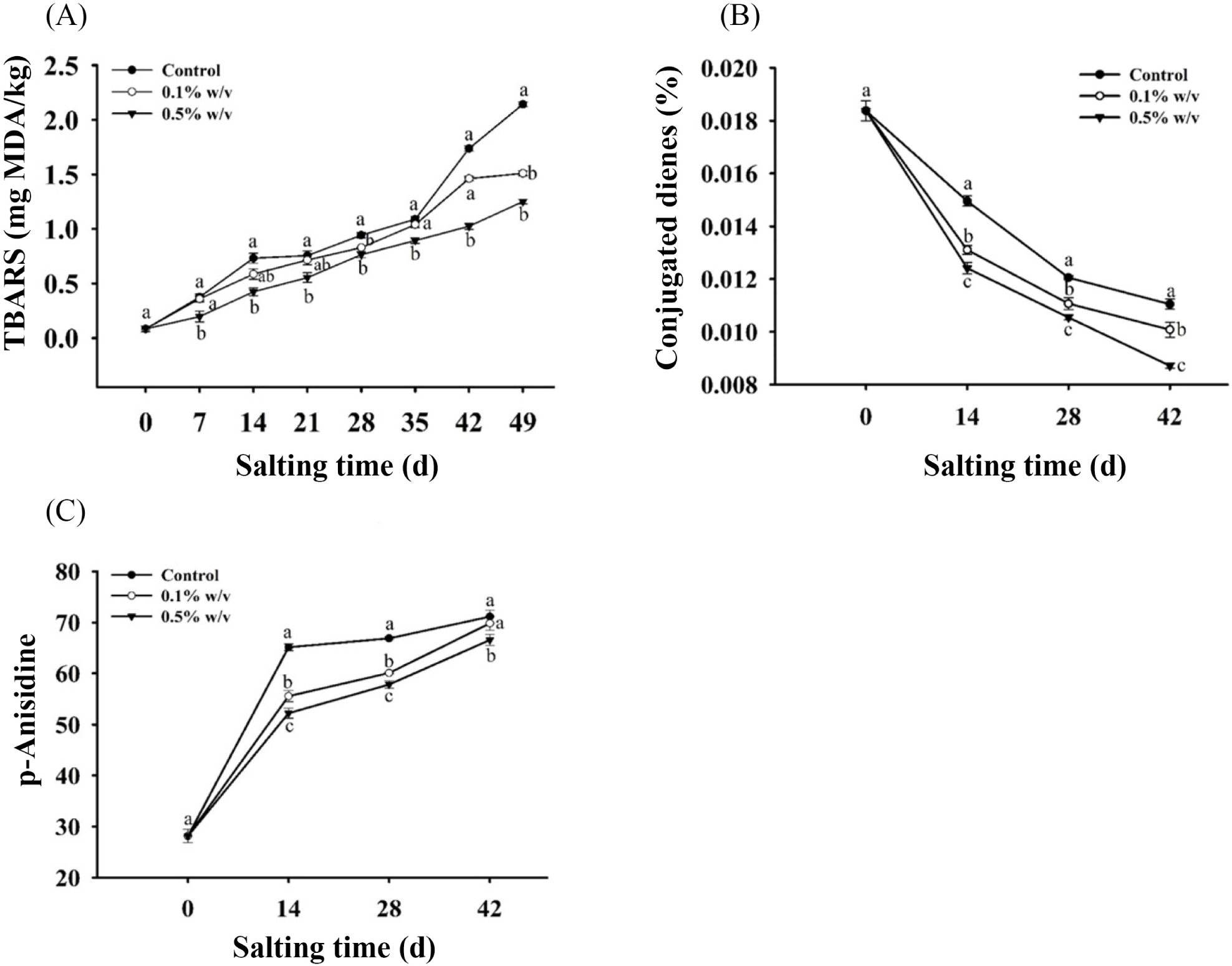
The natural phenolic components in the spices may delay the formation of conjugated dienes (CD), hence, preserved the linoleic acid from degradation. Donation of the hydrogen atom from phenolic compounds to lipid peroxyl radicals, generate the aryloxyl, which failed to act as chain bearer, thereafter, joins second radical to extinguish the mechanism of the radical element (Ruberto et al., 2001). The phenolic components have antioxidative properties, such as hydrogen contributors, reducing agents and extinguish the free radical. Effects of antioxidants on CD at the different concentration levels are shown in Fig. 3B. Both the conjugated dienes value in salted egg yolk treated with RE and control samples declined significantly during salting process period (p<0.05). Control samples exhibited significantly higher values of CD compared to the salted egg yolk supplemented with RE samples over the whole salting process period (p<0.05). It shows that RE was proficient in significantly lowering the CD formation for the whole duration of 42 d during salting as compared to control.
According to a study conducted by Frankel et al. (1996), rosemary’s antioxidant ability in some oil types stored for 20 days at 60°C was evaluated after the supplementation of RE, containing carnosic acid 44 mg/kg and carnosol 6 mg/kg in soybean oil at a concentration of 1,000 mg/kg, effectively inhibited the formation of CD when compared to samples used as controls. Phenolic compounds at specific concentrations considerably lowered the rate of CD formation. When antioxidants were absent, the concentration of linoleic acid declined greatly, representing oxidation. The potency of antioxidation activity for phenolic compounds was associated with the capability to extinguish peroxyl radical molecules (Pawar et al., 2012).
Anisidine analysis is based on secondary oxidation products and is used to determine the amount of aldehyde produced by hydroperoxide breakdown, primarily 2-alkenals and 2,4-dienals. The p-AV of all tested samples increased dramatically as the salting period increased, as seen in Fig. 3C. In comparison to a control sample, adding antioxidant spices to duck egg resulted in a substantial reduction in p-AVs (p<0.05). In a study Samotyja and Małecka (2010), adding RE to soybean oil held at 60°C for 312 hours reduced the final p-AVs from 14 to 8 points when compared to a control sample. Samotyja and Małecka (2010) also reported that adding tocopherol could inhibit the hydroperoxides particles construct 1.70 times more than the control group, it created the higher value of anisidine. This circumstance might be associated with the existence of phenolic compounds that contribute a hydrogen atom to peroxy radicals, and to create constant hydroperoxides (Alizadeh et al., 2015).
Supplementary Fig. S2 shows the GC-MS total ion chromatogram of FA components in salted duck egg treated with RE. The percentage of total FAs in salted yolk lipids supplemented with RE is shown in Table 1. There were 18 FAs detected in all of the group samples. In yolk lipid, monounsaturated fatty acid content was significantly lower (p<0.05) than saturated fatty acid (SFA) and polyunsaturated fatty acid (PUFA) content, and ω-6 PUFA content was significantly greater (p<0.05) than ω-3 PUFA content. A suitable balance of ω-6/ω-3 in the range of 1–4 in terms of cardiovascular diseases; according to the British Department of Health and Nutrition (Wang et al., 2014), an optimal balance of ω-6/ω-3 in the range of 1–4 in terms of cardiovascular problems is a key component for balanced nutritional intake. The ω-6/ω-3 ratio ranged between 20.53 to 36.66 in this study. When FA values in fresh duck egg yolks were compared to those supplemented with RE, the ratio ω-6/ω-3 was reduced dramatically from 36.66 to 15.72 after 28 days of salting (p<0.05). Moreover, the egg yolk treated with RE 0.5% (w/v) had a PUFA/SFA ratio of 0.65, which is comparable to the recommended value for human diet (Wang et al., 2014).
Palmitic acid (16:0) was the most prevalent FA after 28 days of salting, followed by linoleic acid, and arachidonic acid (ARA; C20:4ω-6). At 28 days after salting, palmitic acid was the most prominent SFA in all samples, and its concentration was considerably greater than the control samples (p<0.05). The most dominating PUFA in all treatment groups was linoleic acid, followed by ARA, and the concentration of linoleic acid in egg supplemented with RE was substantially greater than control samples (p<0.05) at 28 days after salting. It is widely acknowledged that omega-3 PUFAs have major health benefits for humans (Fraeye et al., 2012), particularly when it comes to cardiovascular activity. The results of this study show that after 28 days after salting, the contents of PUFA in control samples and those treated with RE 0.5% (w/v) had significant lowest and maximum values at (p<0.05), respectively. In the mammalian brain, omega-3 PUFA, particularly docosahexaenoic acid (DHA), is the most plentiful. Surprisingly, the egg treated with RE had a significantly higher level of DHA (C22:6ω-3) than the control samples (p<0.05). Looking into the PUFA metabolic pathway, this trend could be revealed, with DHA being created from alpha-linolenic acid via a mechanism of hydrocarbon chain elongation and desaturation (Gładkowski et al., 2011). It is critical to understand that ARA (C20:4ω-6) is produced in lipids via the in vivo biosynthesis pathway from linoleic acid. Furthermore, the eggs supplemented with RE had significantly higher ARA content than the control group (p<0.05).
In the presence of RE, the FA composition changed significantly during salting. These effects may be related to the presence of phenolic diterpenes in rosemary, such as carnosol, rosmanol, carnosic acid, rosmariquinone, and rosmaridiphenol, which have been associated to antioxidant activity by preventing free radical chain reactions by providing a hydrogen atom (Zhang et al., 2010). These phenolic diterpenes chemicals are thought to help extend the structural matrix’s intermolecular connections on salted eggs.
Lipid oxidation is one of the most important reactions in the production of volatile compounds in processed foods, which aids flavor development. Supplementary Fig. S3 shows the findings of a total ion chromatogram of volatile chemicals in salted duck egg treated with RE. Table 2 shows the GC peak data for fresh duck egg and salted egg control samples, as well as the qualifications of each component chemical. For fresh duck egg, 25 volatile compounds were identified and classified into nine groups: 3 esters, 3 alkenes, 8 nitrogenous compounds, 1 alcohol, 2 ethers, 5 organic acids, 1 aldehyde, and 2 others; for salted egg control samples, 1 esters, 5 acids, 4 alcohols, 4 alkanes, 2 ketenes, 3 aldehydes, 2 nitrogenous compounds, 3 ethers, and other. In the fresh egg and salted egg control, seven volatiles chemicals (n-hexadecanoic acid, 2-methylaminomethyl-1,3-dioxolane, 3-azabicyclo [3.2.2] nonane, 1,4,7,10,13,16 hexaoxacyclooctadecane, nonanal, octaethylene glycol monododecyl ether, and 15-crown-5) were detected.
At 28 days after salting, Table 2 reported all quantifiable components of salted egg augmented with RE 0.1% and 0.5% (w/v). For salted egg enhanced with RE 0.1% and 0.5% (w/v), 22 and 25 volatiles were qualified and categorized into 9 groups, namely 5 esters, 5 organic acids, 3 alcohols, 1 alkane, 1 ketone, 4 aldehydes, 2 nitrogenous compounds, 1 ether, and 4 organic acids, 1 alcohol, 3 alkenes, 2 ketenes, 7 aldehydes, 2 nitrogenous compounds, 3 ethers, and 3 others. Five volatiles compounds were discovered in salted duck treated with RE 0.1% and 0.5% (w/v): acetic acid, n-hexadecanoic acid, ethanol, 1, 4, 7, 10, 13, 16 hexaoxacyclooctadecane, and 15-crown-5.
Several esters were detected in the yolk when RE 0.1% (w/v) was added. Esterification is a chemical reaction that produces esters by combining free fatty acids and alcohols. Alcohols are thought to play a little role in food taste creation due to their high flavor margins (Mensink et al., 2003). Salted egg enriched with RE 0.1% and 0.5% (w/v) provided three and one alcohols, respectively. The process of lipid oxidation has also been linked to the creation of alcohol (Qin et al., 2012). Furthermore, acetic acid was discovered in duck eggs containing RE, which is thought to be a by-product of the long chain FA breakdown process. In addition, hexanoic acid was discovered in salted duck egg supplemented with RE, which was generated enzymatically using PUFA via the lipoxygenase route (Wei et al., 2013).
This report qualified alkanes, which make little contributions to food flavor systems. Several previous studies identified aldehydes as the major components in fried eggs (Wang et al., 2014). Numerous aldehydes were discovered in the current study. The aldehydes were formed through the Strecker degradation of amino acids or the destruction of oxidative elements in unsaturated fatty acids during processing (Wang et al., 2014). Decarboxylation of ketonic is the most common approach to make ketone compounds by changing two carboxylic acid molecules during the heating process (Renz, 2005). The ketones compounds could have been produced by carboxylic acid secondary reactions after processing, as there were no ketones compounds discovered in the fresh egg groups (Martins et al., 2000). Furthermore, only a few ketone molecules were discovered in duck eggs supplemented with RE. In egg samples supplemented with RE 0.1% and 0.5% (w/v), two nitrogenous compounds were identified (Table 3), while one and three ether compounds were also found in egg samples supplemented with RE 0.1% and 0.5% (w/v).
Using an array of sensors that respond to volatile chemical substances, E-nose attempts to mimic mammalian olfactory systems (Cramp et al., 2009). Recent advancements in e-nose, as well as sophisticated statistical techniques involving chemometric characteristics and artificial intelligence, have vastly expanded the scope of flavor analysis. E-nose machine is a piece of equipment that consists of an air sampling instrument and a system of gas sensors that is connected to a computer. The ability of an E-nose device’s sensor array function to react differently to different flavors is one feature that distinguishes it from other flavor-related devices. Thousands of different volatile flavor chemicals can make up a single flavor. Individual flavor chemicals in a single flavor sample can be certified and determined using common spectrometry analytical techniques like GC-MS. The e-nose, on the other hand, can respond to complete taste compound samples in the same way that a human nose can. In the human olfactory system, it is not necessary to isolate specific components as part of the sample analysis process; the flavor is processed as a whole and detected using our brain (for example, identifying a flavor pattern from a memory bank; Sohn et al., 2008).
Response value (Supplementary Fig. S4) and radar chart of the volatile flavor compounds in salted duck egg treated with RE at 28 d of salting was shown in Fig. 4A. The radar outline of salted duck egg enriched with RE aroma changed after 28 days of salting, according to the findings. Fresh egg samples had considerably lower relative values of LY2/AA, LY2/GH, LY2/G, and LY2/gCTL than salted duck egg samples. While the relative values of P30/1, PA/2, T30/1, P10/1, T70/2, P30/2, P40/1, P10/2 for salted duck egg treated with 0.5% (w/v) RE were significantly higher than the control samples.
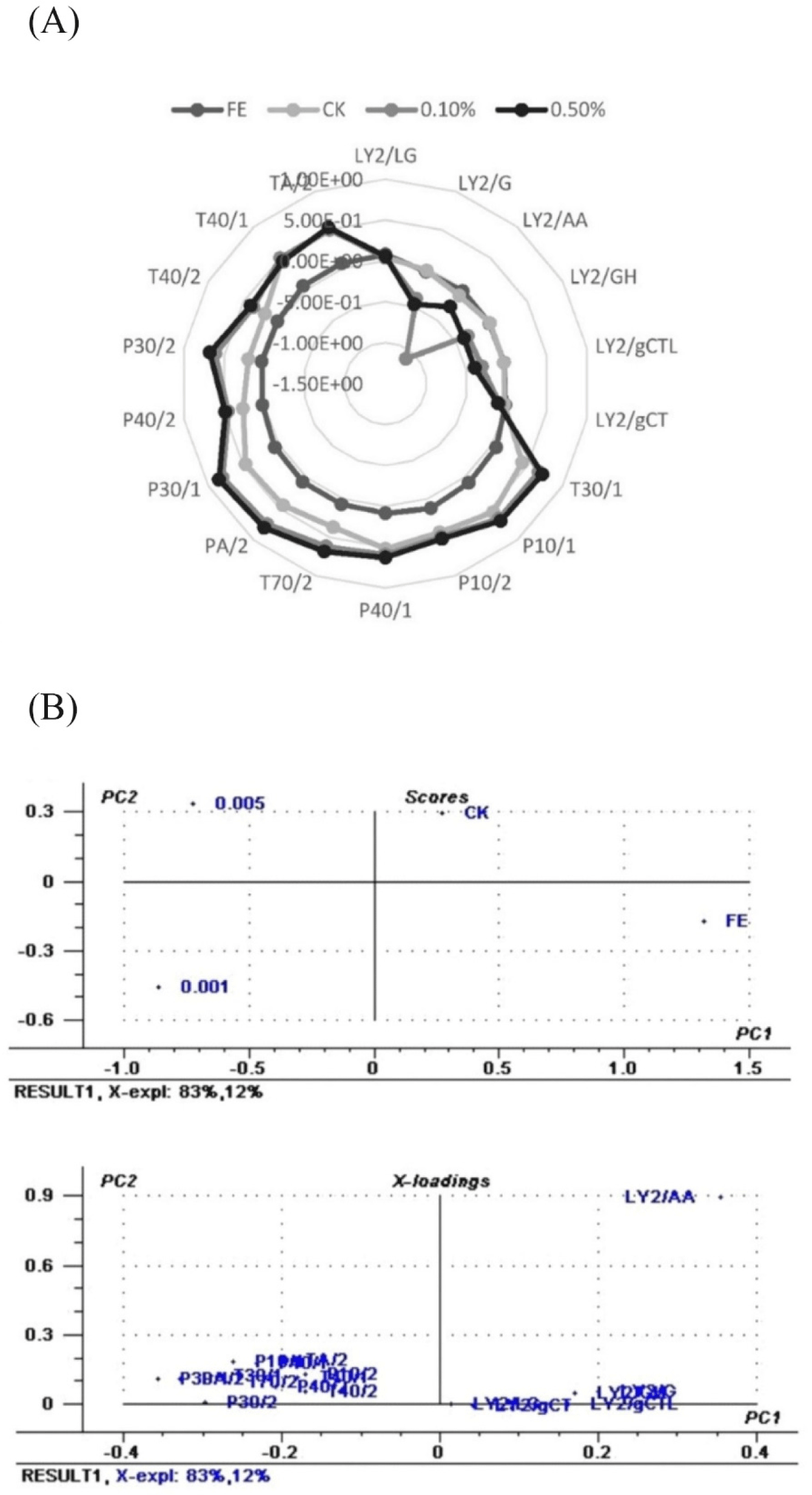
Fig. 4B shows the score and loading plot of the salted egg control, as well as salted eggs treated with RE 0.1% and 0.5% (w/v) sensor response data. PC1 and PC2 both contain 100% of the entire variance (PC1-83% and PC2-12%). After 28 days of salting, four groups were clearly separated: Fresh egg, control, and groups supplemented with 0.1% and 0.5% (w/v) RE, showing that adding varying concentrations of RE to the duck egg salting solution played crucial characteristics in the new flavor of salted duck egg.
E-nose technology has 18 distinct types of sensors that can detect flavor particles as well as concentration. Fig. 5 shows typical curves created by E-nose for volatile compounds in flavor. During the salting time, constant transforming signals were noticed, reflecting eighteen various types of sensors. Salted duck egg with RE were found to be significantly different from fresh and control duck eggs, indicating a clear alteration of volatile flavor components in duck egg throughout salting time. The disparities were discovered due to the establishment of unique volatile flavor components in each of the tested samples at different quantities (Cui et al., 2015).
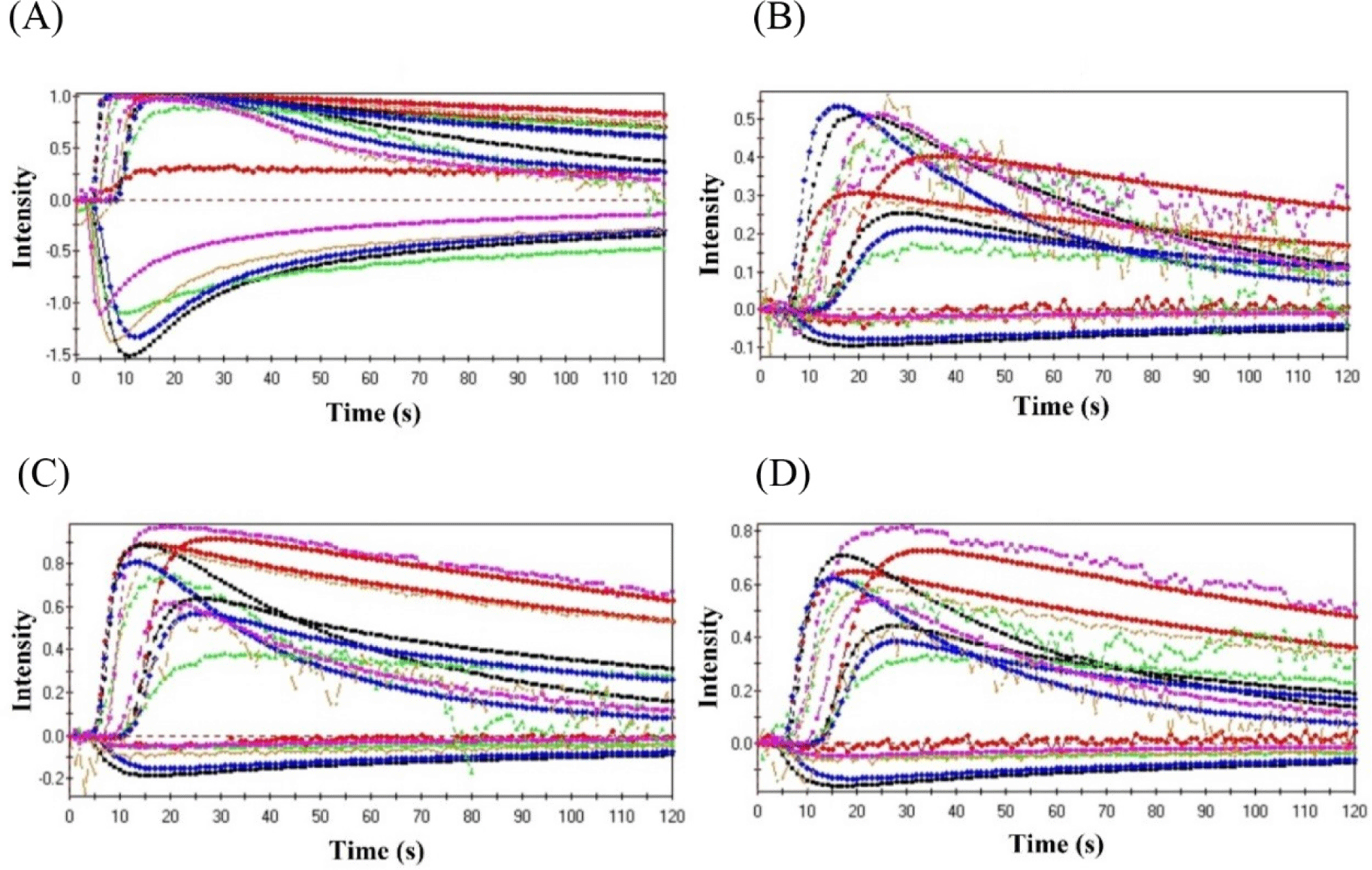
Conclusion
In a time-dependent manner, the RSA and reducing power of salted duck eggs treated with 0.5% (w/v) RE were considerably higher than the control at 28 days after salting for egg white and egg yolk. The salted duck egg treated with 0.5% RE (w/v) had the maximum reducing power of egg white and egg yolk, followed by 0.1% RE (w/v) and the control sample, respectively. The FA profiles of salted egg were significantly affected by RE and salting time. Palmitic acid was the most abundant FA in salted egg improved with RE, followed by linoleic acid and ARA. Furthermore, The RE applied to duck eggs also had higher DHA than the control samples. TBARS levels were substantially lower in salted egg supplemented with RE than in the control, and TBARS levels increased with increasing salting period. In comparison to the control, RE was also capable of dramatically decreasing conjugated dienes production throughout the course of 42 days of salting. In comparison to a control sample, adding antioxidants to duck egg resulted in a considerable reduction in anisidine levels. The findings suggest that this spice slowed the oxidation process during the salting procedure. The RE’s protective effect may be attributed to its ability to suppress the lipid oxidation process.