Introduction
The excessive use of antibiotics produced resistance in animals’ microbiota, with the ability to transfer antibiotics resistant genes into human microbiota (Mingmongkolchai and Panbangred, 2018). As more and more cases of antibiotic resistance emerged from all over the world, the controversy on their use became a global issue (Cogliani et al., 2011). It was reported that the use of antibiotics in poultry feed caused diseases in humans, due to the development of drug-resistant strains of Campylobacter jejuni (Iovine and Blaser, 2004). Health issues related to the use of antibiotics have been systematically reviewed (Muhammad et al., 2020). Many studies have now identified the presence of antibiotics in water and soil (Jiang et al., 2013; Kim et al., 2017; Yang et al., 2011), and the excessive use of antibiotics enrich the environment with antibiotic-resistant bacteria and genes (Franklin et al., 2016). Due to all the problems associated with their use, antibiotics have been prohibited for the animal feed industry, and Sweden was the first country to ban them in 1986 (Castanon, 2007). However, prohibiting the use of antibiotics caused a decrease in production, which suggested the urgent need to find a potential alternative to antibiotics.
In recent years many researchers focused to find out some novel and beneficial replacements of antibiotics with the potential of promoting growth and preventing birds from pathogens (Yadav et al., 2016). Due to their useful applications for animals and humans, probiotics were considered as a potential alternative to antibiotics (Zorriehzahra et al., 2016). Probiotics include different species, such as bacteria, fungi, and yeast (Iannitti and Palmieri, 2010). The use of probiotics has been increased during the last few years, as they can improve the production performance and enhance the immune system (Mingmongkolchai and Panbangred, 2018).
However, the researchers now believe that spore-forming probiotics (SFPs) are the best alternative among the available options (Popov et al., 2021). The mechanism of antibiotics as growth promoters is based on their interaction with intestinal microbiota (Niewold, 2007). Due to their encapsulation ability, the SFPs can reach the specific part of the gastrointestinal (GI) tract easily. During the last few decades, the SFPs showed significant improvement in prophylactic activities, growth promotion, and feed conversion rate in broiler as well as laying hens (Popov et al., 2021).
Many studies reported the use of different strains of SFPs in poultry feed. So, to help the researchers for a better insight into the effect of SFPs on poultry, we summarized the valuable literature.
Why Spore-Forming Probiotics
Despite of no clear boundaries, the microbiota found in different parts of the GI tract varies in quantity, characteristics, and composition (Oakley et al., 2014). Due to differences in the environment, all microorganism are not able to survive in different types of environment, it became one of the most important parameter, while choosing the probiotic species. Because the probiotic should be able to reside in the area of interest in GI tract. The diversity and dynamic nature of the GI environment make gut microbiology a quite complicated area of research (Cartman et al., 2007). Hence, it is necessary to find potential probiotics, which can colonize in the area of interest.
SFPs have a greater ability to survive and pass through the GI tract and also to proliferate and colonize the digestive tract of animals (Popov et al., 2021). The stability of spores during their passage through the upper GI tract was better as compared to vegetative cells, and the spores were able to proliferate in lower parts of the GI tract and exhibit their beneficial properties (Mingmongkolchai and Panbangred, 2018). This ability makes SFPs a best feed additive for livestock, especially in the poultry industry (Popov et al., 2021).
Furthermore, the veterinary sector has now acknowledged that the application of spores has several benefits over the vegetative cells. As compared to vegetative cells, spores are more easily stored, manipulated, and delivered to farm animals (Todorov et al., 2021).
Mechanism of Action of Spore-Forming Probiotics in Poultry
Poultry production in a commercial environment faces continuous exposure to the various microbes usually through their mucosa (Broom, 2019). Depending upon the interaction of host and microbe diseases may occur (Garcia et al., 2010).
The mechanism of action of SFPs is still not very clear. However, it is reported that the mechanism of action of SFPs is the same as the normal probiotic microorganisms (Cartman et al., 2007).
The mode of action of probiotics in poultry was reported in many different ways (Fig. 1). The first strategy was known as the competitive exclusion, competition for the adhesion sites prevents the colonization of pathogenic bacteria (Chichlowski et al., 2007; O’Dea et al., 2006). This competitive exclusion was achieved through the adhesion of beneficial bacteria (Chichlowski et al., 2007). Maintenance of this activity depends upon the ability of the SFPs strain to adhere to the intestinal wall (Fuller, 1989). It was reported that the Bacillus spores can not only germinate and grow in the intestine (Nakano and Zuber, 1998) but also re-sporulate in the lower part of the small intestine (Tam et al., 2006). Furthermore, the spore’s adhesion to the intestinal wall can help to retain the spores in the intestine (Popov et al., 2021), thus can contribute to competitive exclusion. The adhesion of probiotics to the gut mucosa is important for colonization and interaction with the host (Bermudez-Brito et al., 2012).
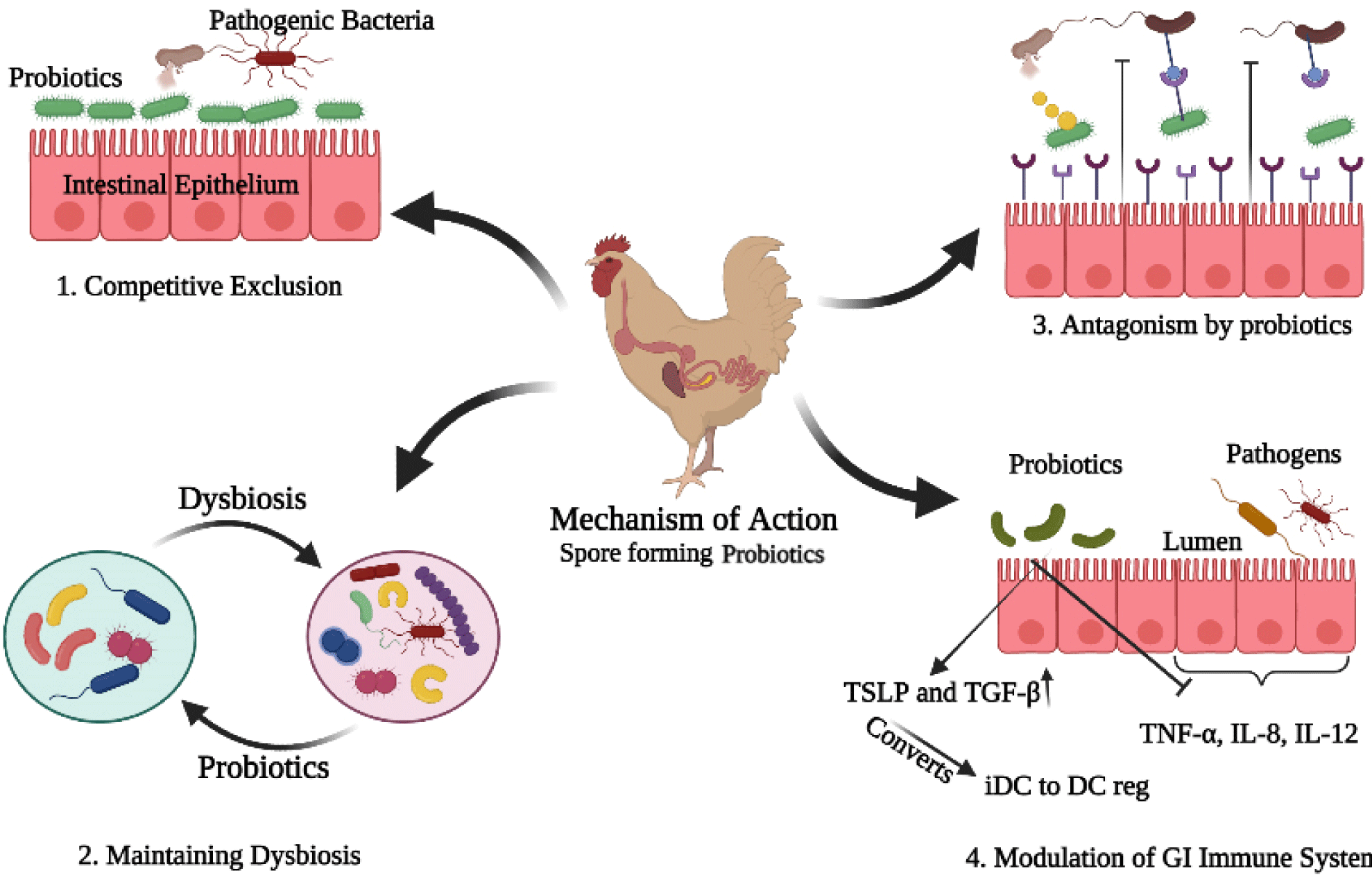
The gut bacteria produce metabolites that control the growth of pathogenic compounds and compete for adhesion in the intestinal epithelium. These metabolites are short-chain organic acids, bacteriocins as well as hydrogen peroxides (Dankowiakowska et al., 2013), help in attachment to the intestinal mucosal layer (Buck et al., 2005). The newborn has a sterile digestive system and before its organs produce antibodies, microbes from the environment start to colonize in the gut. Therefore the use of probiotics due to their adhesion ability with the intestinal mucosa creates a natural blockade against disease pathogens (Dankowiakowska et al., 2013).
The second mode of action of probiotics was reported through balancing the dysbiosis. The enteric dysbiosis may cause alteration in host-microbial interaction, leading to disease conditions (Byndloss and Bäumler, 2018; Plaza-Díaz et al., 2018). Recently, it was suggested that probiotics can treat dysbiosis and restore the imbalanced or disturbed microbiota (Mendes et al., 2018; Vieira et al., 2016). Another study suggested the same findings that probiotics can maintain the colonization of disturbed gut microbiota (Plaza-Diaz et al., 2019). This mechanism suggests that the balanced gut microbiota also helps to reduce the emission of ammonia through feces.
The third mechanism was achieved through the antagonistic activity of probiotics. Probiotics produce a class of small antimicrobial molecules (bacteriocins, mucin, defensins) which obstruct the pathogens, and their colonization (Khan and Naz, 2013). One study reported that bactericidal substances produced by bacteria can lyse the pathogens, and receptors on the surface of probiotics can mimic the intestinal wall, and antagonize the pathogens, and then they neutralize them (Sleator, 2010). Similarly, it was suggested that the mucin secreted by the intestinal epithelial cells, reduces the adhesion of pathogenic bacteria (Preedy, 2010).
The fourth mechanism of probiotic action is known to modulate the GI immunity, which is also considered as the most important benefit of probiotics. Different studies have shown that probiotics induce anti-inflammatory mediators, such as interleukin-10 (IL-10), thymic stromal lymphopoietin (TSLP), and transforming growth factor beta (TGF-β). And simultaneously decrease the production of pro-inflammatory cytokines such as interleukin 8 (IL-8) and tumor necrosis factor-alpha (TNF- α) (Georgieva et al., 2015).
Effect of Spore-Forming Probiotics on Different Key Aspects of Poultry
Among all spore formers, bacterial spores are particularly well studied for use as a probiotic because they are dormant, metabolically inactive, and highly resistant to environmental stresses (Elisashvili et al., 2019). These distinctive properties are beneficial for the commercial perspective which means that they have a long shelf life and can maintain viability during distribution and storage. Despite all this knowledge, how the bacterial spores may function as probiotics are limited at present.
Spore germination is requisite to function as a probiotic. Jadamus et al. (2001) first time experimented to check the germination of bacterial spores in the GI tract of poultry and concluded that Bacillus cereus var. toyoi germinates rapidly. Later, a similar study was performed by Cartman et al. (2008) suggested that the spores must be viable to function as a probiotic and to produce the antimicrobial compounds, and the results reported that germination of Bacillus subtilis spores occurs in the GI tract of poultry. Another study reported that the spore germination in the GI tract of poultry occurs at such a faster rate that 90% of spores germinated within one hour (Latorre et al., 2014).
Bacillus spp. are generally known as aerobic bacteria, and it is important to know that how they survive in the anoxic environment of the GI tract if germinates. So, the studies concluded that these bacteria can use nitrate or nitrate as an electron acceptor instead of oxygen for their growth and survival (Nakano and Zuber, 1998; Shivaramaiah et al., 2011).
Currently, the excess emission of ammonia is a major environmental problem related to the poultry industry. The SFPs and the environment of the GI tract can contribute to overcoming this serious issue (Fig. 2). It was reported that the uric acid produced in the chicken’s liver is excreted into the intestine and microbial urease converts it into ammonia by hydrolysis (Karasawa et al., 1988). Many intestinal microorganisms (Clostridia, Bacteroides, and Proteus) possess urease activity (Ahmed et al., 2014), through which uric acid is hydrolyzed into ammonia. Gram-positive, SFPs have been reported to inhibit the production of urease-producing bacteria, either by inhibiting the growth of pathogenic bacteria, producing antimicrobial compounds, or by lowering the pH, which in turn reduce ammonia production in ceca (Goudarzi et al., 2017). Similar studies reported that Bacillus subtilis reduced the emission of ammonia and promoted the growth performance by inhibiting the pathogenic bacteria in broiler chicken (Chen et al., 2012; Teo and Tan, 2007). Thus the stabilized gut microbiota maintains the intestinal health of poultry (Hong et al., 2005). Zhang et al. (2013) reported that the dietary supplementation of B. subtilis can improve the enzymatic activity of intestinal microbiota and improved microbial balance in the GI tract was the main reason for the reduction in ammonia production. According to Han et al. (2001), several studies reported that infeed probiotics have reduced ammonia by improving the feed efficiency and microbial ecology of GI tract. Similarly Jeong and Kim (2014) observed a significant decrease in ammonia excretion when they used Bacillus strains in poultry feed. Ahmed et al. (2014) suggested that Bacillus amyloliquefaciens is also an effective microorganism for suppressing ammonia production by improving the environment of GI tract.
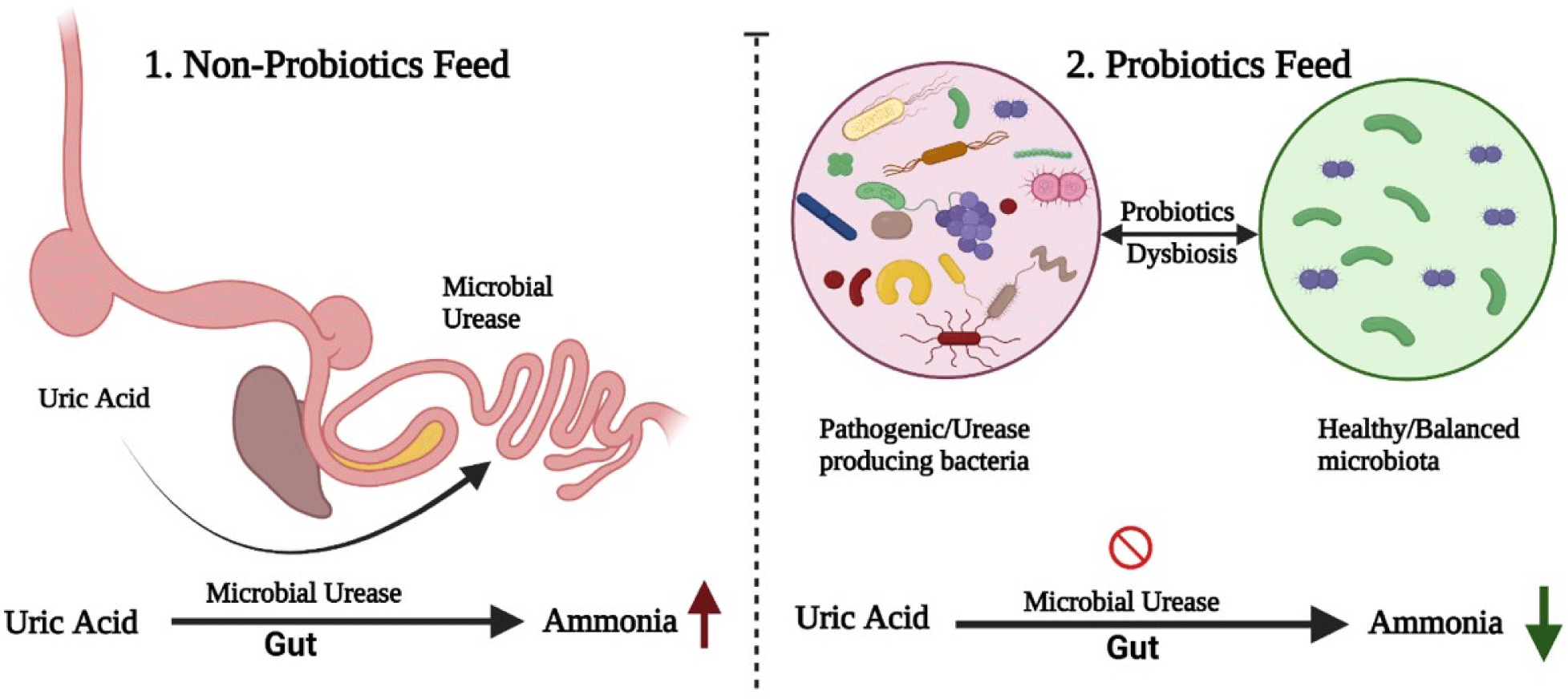
Poultry farmers aimed to produce eggs with normal shape, eggshell thickness, and eggshell strength to maintain the freshness of eggs according to the consumer’s demand (Fouad et al., 2016). Studies suggested that diets supplemented with B. subtilis; a spore-forming bacterium can improve the production performance and eggs quality for economic benefits (Xu et al., 2006).
It was very important to note that the stress conditions affect the eggshell thickness, and thus increase the number of cracked or broken eggs. SFPs are reported to heal the birds suffering several stressors (Fathi et al., 2018). It was reported that the increase in the availability of intestinal calcium produced a positive effect of probiotics on the eggshell strength (Świątkiewicz et al., 2010). An increase in eggshell thickness and reduction in damaged eggs was recorded in the hens, fed diet fortified with SFPs B. subtilis, which may be related to the calcium retention (Fathi et al., 2018).
Another study reported that compared to the control group significant increase in the egg production rate was observed, when hens were fed with Lactobacillus sporogenes, as well the persistency was better in the probiotic group (Panda et al., 2008). But some studies reported contradictory results, which might be pertinent to the bacterial strain, form, and concentration used (Nahashon, 1992; Tortuero and Fernández, 1995).
The stabilized gut microbiota can help to improve the feed conversion ratio and consequently enhance the digestion as well as absorption of nutrients (Pan and Yu, 2014). Panda et al. (2003) revealed that the Lactobacillus sporogens supplemented diet improved weight gain and FCR in broilers. The inclusion of B. subtilis C-1302 at the level of 300 and 600 mg/kg of diet showed positive effects on the growth rate (increased average daily gain) and decrease the FCR in broilers (Jeong and Kim, 2014). Similarly, Fathi et al. (2018) concluded that dietary supplementation of B. subtilis had no significant effect on the FCR of laying hens. According to Lei et al. (2013) adding Bacillus licheniformis to the diet showed no significant effect on the feed consumption and FCR of the laying hens. Furthermore, probiotics have been reported to give inconsistent results for average daily feed intake (ADFI; Otutumi et al., 2012) sometimes showing no effect at all. A significant increase in ADFI was observed when Hy-Line Brown laying hens fed a diet supplemented with Bacillus velezensis (Ye et al., 2020). Ross 308 broiler chicks fed a diet supplemented with Clostridium butyricum also showed significant increase in ADFI (Zhao et al., 2013). However, many studies reported no significant effect increase in ADFI, such as Broiler Cobb 500 fed a B. subtilis supplemented diet (Oladokun et al., 2021), Hy-Line Brown laying hens fed a diet supplemented with B. amyloliquefaciens (Zhou et al., 2020) and Hy-Line Brown laying hens fed a diet supplemented B. licheniformis (Yang et al., 2020). Zhu et al. (2009) explained that the benefits associated with probiotics depend upon many factors, such as probiotic species, strain, application method, age of flocks, over all hygiene and housing conditions on farm, and external environmental factors.
The basic and most important organs of the immune system consisted of the thymus, bursa of Fabricius, and spleen, which are involved in the production and differentiation of immune cells and production of antibodies (Fouad et al., 2016). Stress leaves damaging effects on the natural defense system and intestinal epithelial cells of hens (Söderholm and Perdue, 2001; Taché et al., 2001). The response to the immune system as well as intestinal epithelial cells is directly controlled by the neuroendocrine system (Levite, 2001; Petrovsky, 2001).
The commensal bacteria present in the gut, influence the development of the immune system by interacting with the probiotics (Tannock, 1999) because these commensal bacteria have close contact with the cells of the immune system (Haghighi et al., 2005). Colonization of microbes in the chicken gut begins early after hatching and becomes stabilized within the first fifteen days of their life (Rehman et al., 2007). Another study suggested that some immunomodulatory effects of probiotics involve changes in signaling pathways and activation of transcription factors and thus enhance the expression of messenger RNA genes, related to innate immunity (Al-Khalaifah, 2018). RNA of the immune system, for example, the maturation process of the intestinal TCRαβ T cell (Mwangi et al., 2010) and the immunoglobulin repertoire (den Hartog et al., 2013) is dependent on the enteric microbiota.
The storage time and processing depend upon the physicochemical properties of the meat (Popova, 2017). Bacillus-based probiotics were reported to improve the pH, stiffness, and color of the meat (Pelicano et al., 2003). Color is an important quality parameter because more than 80% of the consumers focus on the color of meat when they tend to buy it (Bai et al., 2017; Węglarz, 2010). Recently, a spore-forming bacteria, B. amyloliquefaciens B-1895 was reported to improve the meat mass in broilers. Similarly, Liu et al. (2012) showed that hens drinking B. licheniformis supplemented water showed significantly improved meat color, juiciness, and also high protein content. Studies showed that SFPs can improve meat quality, but the type of probiotic and the approach of administration can influence the performance (Bai et al., 2017). As observed by Hossain et al. (2012) the higher efficiency ratio of protein may help to promote meat yield.
Bacillus-based SFPs can produce beneficial enzymes, which help to regulate the function of the digestive system (Ramlucken et al., 2020). Enzymes such as protease and carbohydrate help to lower the non-digestible proteins and carbohydrates, which act as a nutrient source for pathogenic bacteria (Kiarie et al., 2013). Ramlucken et al. (2020) reported that poultry is not known to produce enzymes for the digestion of non-starch polysaccharides, which if remain un-hydrolyzed results in low feed conversion. So, the addition of enzymes through the feed is necessary, and enzyme-producing probiotics are the best alternative. The study reported that Bacillus spp. can produce several exogenous enzymes (protease, β-glucanase, amylase, xylanase, phytase, lipase, and cellulase) which are crucial for the digestion in poultry (Latorre et al., 2015). These probiotic enzymes improve the nutrient availability to the microflora in the GI tract (Ramlucken et al., 2020). Wang and Gu (2010) reported that Bacillus coagulans increased the activity of protease and amylase, which in turn improved the broiler growth. Study performed in our lab has isolated protease and β-glucanase producing B. velezensis Y1 strain from the manure of piglets (Khalid et al., 2021). Several other studies have already reported the enzyme production ability of Bacillus species (Adeola and Cowieson, 2011; Latorre et al., 2014). All these enzymes (protease, lipase, glucanase, cellulase, xylanase, and phytase) can help to balance the anti-nutritional factors in the feed (Popov et al., 2021).
Future Implications
The review concluded that several SFPs have been studied until now, but a few of them were used commercially such as B. subtilis, B. licheniformis, and B. cereus (Larsen et al., 2014). However, another recent study used B. amyloliquefaciens B-1895 in poultry feed and reported beneficial results (Farhat-Khemakhem et al., 2018).
Recently, our lab has isolated a strain of spore-forming B. velezensis from the manure of piglets (Khalid et al., 2021), described its characteristics (Ye et al., 2018), and used it in poultry feed. The results showed significant improvements in different growth parameters, blood biochemistry, and egg quality indices of laying hens (Ye et al., 2020). Hence, we suggest that B. velezensis being a spore-forming species can also be used as a probiotic in poultry feed.